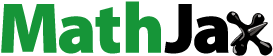
ABSTRACT
Spherical silver nanoparticles of required size were synthesized employing a seed-mediated approach using leaf extract of Azadirachta indica. Reaction pH was used to manipulate the rate of reduction of the precursor salt for achieving a fast rate of reaction needed during the synthesis of silver nanoparticles seeds and a slower rate of reaction needed for controlled growth and inhibition of secondary nucleation. A mathematical model was developed for staggered growth of silver nanoparticles using a multi-step growth approach. The developed model was used to predict the size of silver nanoparticles after various growth steps and was compared with the experimentally achieved results. Transmission electron microscope images were used for the determination of the size of the synthesized silver nanoparticles. The mathematical model appears to be a promising tool in enabling real-life application of green synthesized silver nanoparticles.
1. Introduction
Nanotechnology is the dominant technology in today’s world because of the scope of tailoring of the functionalities by altering the size, shape or constitution of nanomaterials [Citation1–3]. The optimization of nanoparticle synthesis, therefore, has attracted a lot of attention as it is the prime tool in controlling the morphology of synthesized nanoparticles [Citation4–7]. Silver nanoparticles are one of the most widely researched nanoparticles because of their wide applicability in fields such as biomedical applications [Citation8], catalysis [Citation9], electronics [Citation10] and in antimicrobial applications [Citation11–14]. The last one decade has seen a surge in research in the field of green synthesis of nanoparticles [Citation11–17], owing to environmental concerns [Citation18]. The properties of nanoparticles are extremely sensitive to their morphology [Citation19–21], which increases the potential for their applications but at the same time makes their precise and reproducible synthesis imperative. Synthesis of silver nanoparticles is most commonly carried out by the reduction of silver precursor salt in solutions. Precision in synthesis requires a very precise understanding of the kinetics involved in the nucleation and the growth kinetics involved in the formation of the nanoparticles. A keen understanding of the kinetics of crystallization so that we can use it as a tool for the engineered synthesis of nanoparticles. Green synthesis, however, in terms of understanding of crystallization kinetics is a dark zone primarily because of the lack of understanding of the reduction and the stabilization mechanism involved in the formation of silver nanoparticles. Leaf extracts are a rich cocktail of many organic compounds [Citation22], which may undergo seasonal and regional variations [Citation23] and it is difficult to ascertain the role of the many organic compounds present some of which may be synergistically contributing towards the reduction and stabilization of nanoparticles. This is the reason for greater control in case of chemical synthesis in comparison to the green synthesis of silver nanoparticles.
The aim of the present article is to develop a mathematical model to enable the green synthesis of spherical nanoparticles of required size. The model is based on the growth of “seed” silver nanoparticles in multiple steps. The degree and rate of creation of supersaturation play a very crucial role in the process of crystallization [Citation24]. In the present study, a rapid creation of high supersaturation was used in the creation of a large number of nuclei by “burst nucleation”; a frequently used a technique for the synthesis of monodisperse seed nanoparticles [Citation25]. The nanoparticles synthesized by burst nucleation are of narrow size range because of the short time duration during which the nucleation occurs. The growth of the pre-synthesized silver nanoparticle (AgNP) seeds to larger sizes was carried out in multiple growth steps, which enabled the synthesis of nanoparticles of required size.
2. Materials and methods
2.1. Materials
Silver nitrate and sodium hydroxide, used in the study, were of analytical grade and were procured from Merck, India. Fresh leaves of Azadirachta Indica were picked from the institute, the IIT Delhi, campus in the month of April. The water used was ultra-filtered water (resistivity 18.2 MΩ-cm).
2.2. Preparation of leaf extract
Fresh leaves of Azadirachta indica were thoroughly washed with distilled water and dried in shade. Finely cut leaves were added to ultra-filtered water (20%w/Vv) and was heated at 65–70°C for 10 min with constant stirring using a magnetic stirrer. The extract was filtered using Whatman No.1 filter paper and was stored at 4°C for subsequent use.
2.3. Synthesis of AgNP seeds
For the synthesis of AgNP seeds, 9 ml of 2 mM aqueous AgNO3 solution was reacted with 1 ml of Azadirachta indica leaf extract. The reaction pH was kept at 12. All the reactions were carried out at an ambient temperature of 26°C. The colour of the solution changed almost immediately from colourless to brown, indicating the formation of the silver seed particles. The seeds were spherical silver nanoparticles of size range 5–7 nm.
2.4. Stepped growth of AgNP seeds
For causing an increase in the size of pre-synthesized silver seed particles, the growth was carried out at two stages to enable the synthesis of a larger range of nanoparticle sizes.
2.4.1. Seed growth stage I
In this stage I, 0.15 ml of the seed colloid was added to a flask containing 8.85 ml of ultra-filtered water and 1 ml of Azadirachta indica leaf extract and was shaken well for even dispersal of the AgNP seeds in the aqueous medium. For each step, 0.1 ml of 2 mM AgNO3 was added drop by drop, waiting 5 min between each drop while shaking the flask gently. The reaction was carried out at a pH of 7 and the reaction time allowed was 20 min before repeating the procedure for the next step. All the reactions were carried out at an ambient temperature of 26°C. A set of 10 growth steps was carried out. An aliquot of 5.05 ml was withdrawn to be used as a seed solution for the “seeded growth stage II”.
2.4.2. Seed growth stage II
Here 5.5 ml of AgNP colloid at the end of seed growth stage I was added to 4.5 ml of mixture of Azadirachta indica leaf extract and ultra-filtered water mixed in the ratio of 1:9. From this, an aliquot of 1.25 ml was drawn and added to 1.25 ml of mixture of Azadirachta indica leaf extract and ultra-filtered water mixed in the ratio of 1:9. The number of AgNP in the growth solution is now 1/16 times that of growth stage I. Similar to the process followed for growth in growth stage I, 0.1 ml of 2 mM AgNO3 was added drop by drop. The procedure was repeated for a maximum of 10 steps, as required for the synthesis of AgNP of desired size keeping an interval of 20 min between each step.
2.5. Development of the mathematical model
The AgNP seeds act as templates for the growth into bigger nanoparticles by the addition of Ag atoms, generated by the reduction of AgNO3. The generation rate of Ag atoms is maintained at a level, which sustains the growth of pre-existing AgNP seeds without initiating fresh nucleation. The number of growth steps required to attain a specific size is dependent on the size of the seeds and the concentration of the seeds in the growth medium.
For the development of the mathematical model, we have assumed a 100% reduction of AgNO3 into Ag atoms and their subsequent incorporation into the NP seeds by the process of nucleation. The atoms generated by the reduction of AgNO3 are the monomers for the formation of AgNP. The percentage of reduction is therefore crucial in calculation of the AgNP concentration in the colloidal solution. A lesser percentage of the reduction will result in lesser concentration of AgNP seeds. The number of atoms “ns” present in a spherical nanoparticle seed of radius RS can be calculated on the basis of comparison of the AgNP volume with that of the atomic volume of silver. The size of the nanoparticle seeds was determined on the basis of transmission electron microscope (TEM) images of the synthesized AgNP seeds. The average number of atoms/AgNP seed can be determined using the relation
(1)
(1) where RS and RAg are the radii of the AgNP seeds formed and that of the Ag atom respectively and 0.74 is the fcc packing factor.
The number of AgNP seeds Ns within the reaction mix was calculated on the basis of two assumptions. The first assumption was that there was complete reduction of AgNO3 into Ag atoms by the reaction,
Here Ag0 is the Ag atoms. The second assumption was that all the Ag atoms in the reaction mix get incorporated into AgNP.
The total number of AgNP seeds in the reaction mix can be calculated as
Here NA is the Avogadro’s number and M(AgNO3) and V(AgNO3) are the molar concentrations of AgNO3 and its volume in the reaction mix, respectively. Therefore, the expression for NS will be
(2)
(2)
2.5.1. Growth stage I
During the growth of seeds, it is important to keep the concentration of Ag0 low so as not to initiate fresh nucleation. The AgNO3, therefore, was introduced drop-wise in the reaction mix, which was constantly shaken. In view of this,
No. of atoms added to seed/step/AgNP will be
Here,
is the number of seeds introduced in the growth medium.
The total volume of seed colloid is actually the total volume of the reaction mix, which is equal to VAgNO3 + VLB. Here VLB is the volume of leaf broth in the reaction mix for AgNP seed synthesis. Hence, number of Ag0 generated per growth step will be Mg × Vg × NA. Also, the number of atoms added to an AgNP seed per growth step will be
(3)
(3) Here Mg is the molar concentration of AgNO3 solution used for the growth of AgNP seeds and Vg is the volume of AgNO3 solution used per step of growth in ml.
The number of added atoms to the AgNP seed after “n” steps in stage I will be represented by the expression (on substituting the expression for NS)
The total increase in volume of a AgNP seeds after “n” steps will be
Increased volume of the grown AgNP will therefore be
The increase in the radius of the AgNP after “n” steps in stage I, therefore, is given by the relation
(4)
(4) A maximum of 10 growth steps are executed in the seeded growth stage I since as the size of the AgNP grows, the addition of the same number of atoms/step were not causing a significant increase in size. For stage II, the number of AgNP was reduced to 1/16 of the number of seeds present in the growth medium of growth stage I.
2.5.2. Growth stage II
The AgNPs with increased size after 10 steps of growth stage I were used as seeds for growth stage II. The number of seeds in the seed solution for growth stage II were . The number of seeds were reduced for growth stage II, to enable more number of atoms to be added per AgNP per step. The AgNP at the end of growth stage I are larger in comparison to that at the beginning and maintaining the same addition rate of atoms would lead to a very insignificant change in radius, making the growth steps impractically high to achieve the target size. The number of atoms added/growth step were same as in the case of stage I since the molarity and the volume of AgNO3 solution added per growth step was kept the same as in growth stage I. Hence, the number of atoms added per seed per growth step in stage II is given by
The number of added atoms to the AgNP seed after “n′” steps in stage II will be represented by the following expression (on substituting the expression for NS)
The growth conditions in the growth stage II are different from that in the growth stage I and therefore the number of atoms added per step will also be different. The growth conditions of the growth stage I are applicable after completing the 10 steps of the growth stage I and therefore n′; the number of growth steps in stage II in terms of total number of growth steps will be, “n” = n – 10.
The increase in the volume of AgNP seeds after n′ steps in stage II is given by the expression
The increased volume after n′ steps of growth stage II is the sum of the initial volume of AgNP seeds, increase in volume of seeds at the end of growth stage I and increase in volume in n′ steps of growth stage II. This can be calculated as
(5)
(5)
The increased size of the nanoparticle at the end of a step during seed growth stage I/stage II is given by Equations (6) and (7). The grown AgNP at the end of stage I acted as the seeds for growth stage II. Therefore, the radius of AgNP after “n” growth steps is given by
(6)
(6)
(7)
(7)
Equation (1) where n ≤ 10 represents the growth during stage I whereas Equation (2) where 11 ≤ n ≤ 20 represents the growth during stage II.
2.6. Characterization of silver nanoparticles
2.6.1. UV–Vis spectra analysis
The formation of silver nanoparticles was verified by analysing the UV–Vis absorption spectrum of the aqueous reaction mix. The spectrum was recorded using Perkin Elmer, Lambda 35 Spectrophotometer. The scanning was carried out for wavelength ranging from 300 to 700 nm. AgNO3 solution having a concentration of 2 mM was used for baseline correction.
2.6.2. Transmission electron microscopy
A drop of silver nanoparticle colloidal solution was loaded on a carbon-coated copper TEM grid. The grid was dried at room temperature for 5 h. Philips TEM (CM-10) was used for recording micrograph images at various magnifications.
3. Results and discussion
3.1. Preparation of AgNP seeds
Seeded growth is a very commonly adopted synthesis technique for producing nanoparticles of uniform morphology. In case of using leaf extracts which are a rich cocktail of many phytochemicals (as reducing agents), the reduction, as well as the stabilization, may be due to synergistic involvement of many organic compounds present in the extract. The process of seeded growth of silver nanoparticles by using leaf extract as a reducing agent is shown in Figure .
The AgNP seeds are the templates of growth and need to be uniform to grow into nanoparticles of uniform size and shape. The seeds of uniform size were obtained by burst nucleation, which needs a very rapid creation of super-saturation above the critical value. The reaction pH influences the redox reaction leading to the generation of atoms; the monomers for a metal nanoparticle formation [Citation26,Citation27]. The stability of synthesized nanoparticles is also affected by the reaction pH [Citation28]. We achieved this by enabling a fast reduction of AgNO3 using Azadirachta indica leaf extract at a highly alkaline reaction pH of 11 [Citation29,Citation30]. The pH was, therefore, kept highly alkaline during the synthesis of seeds to enable a very high reduction rate, leading to burst nucleation and the pH was kept neutral during the growth to keep the reaction rate moderate to allow the seeds to grow and yet to minimize fresh nucleation.
The reactivity of the leaf extract depends on the phytochemicals present in it, which undergo a seasonal change as well regional variation [Citation31–33]. The size of the seeds, therefore, will not be same always. The AgNP seeds synthesized under the study was ∼5 nm. The UV absorption spectra of the colloidal solution of AgNP at 50% dilution and the TEM image of the AgNP seeds are shown in Figures and , respectively.
The high concentration of AgNP seeds can be seen from the TEM image as well as from the high absorbance seen in the UV absorbance spectra. The absorbance is directly proportional to the concentration of nanoparticles [Citation34].
3.2. Using the mathematical model for size-specific synthesis
The AgNP seeds synthesized were of the size ∼5 nm. Using the mathematical model to predict the size of AgNP for the experimental conditions adopted, the sizes of AgNP at various growth steps during stage I and stage II are given in Tables and , respectively.
Table 1. Predicted silver nanoparticle size at the end of each step during growth stage I.
Table 2. Predicted silver nanoparticle size at the end of each step during growth stage II.
The TEM images of AgNP after some specific growth steps have been shown in Figures (a–d). The TEM images of the AgNP seeds and after growth steps 5, 10, 15 and 20 show that the size of the AgNP is close to the sizes predicted by the mathematical model, as listed in Tables and , after the specified growth step. The high concentration of monodisperse AgNP seeds of size ∼5 nm can be seen in Figure (a). The size predicted after 5 and 10 steps were 8.37 and 10.17 nm, respectively. In Figure (b), the size of majority of the AgNP is ∼7–9 nm and some newly nucleated AgNP can also be seen. The average size of the AgNP after 10 growth steps as seen in Figure (c) is 10–12 nm. There is, however, a loss of monodispersity during growth which kept on increasing with each growth step. This can be attributed to the occurrence of fresh nucleation during the various growth steps, which is quite visible in Figure (b), resulting in increase of size distribution. The size difference in AgNP starts narrowing on subsequent growth because when the growth is uniform the size difference which was very prominent in smaller size becomes less significant in larger sized AgNP. This can be seen in Figure (d and e), which are the TEM images for AgNP after 15 and 20 growth steps, respectively, and show the average size of the synthesized AgNP to be close to the predicted size of 20.37 nm after 15 steps and 25.12 nm after 20 steps.
Figure 4. TEM image of silver nanoparticles at different stages of growth (a) n = 0, (b) n = 5, (c) n = 10, (d) n = 15 & (e) n = 20.
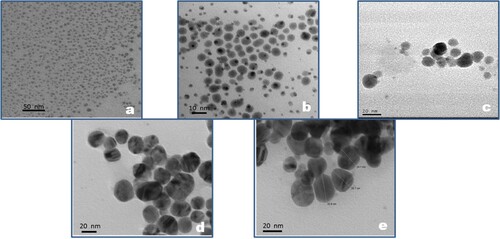
The UV absorbance spectra of the AgNP after specific growth steps are shown in Figure . The peak of the UV absorbance spectrum shows a shift towards higher wavelengths for AgNP with increasing number of growth steps. The shifting of the UV–Vis absorbance peak towards higher wavelengths signifies an increase in the size of the nanoparticles [Citation35]. There is an increase in the magnitude of absorbance also, as the number of growth steps increases. This is because of the increase in the magnitude of the extinction coefficient of AgNP as the size increases [Citation36]. All the spectra, various growth steps show a single peak for UV absorbance, which is a characteristic for spherically shaped AgNP. The increase in the size range of the AgNP after higher number of growth steps is also reflected by the increased broadening of the UV-absorbance spectra with increasing number of growth steps.
Figure 5. UV-absorbance spectra of silver nanoparticle colloidal solution after various growth steps.
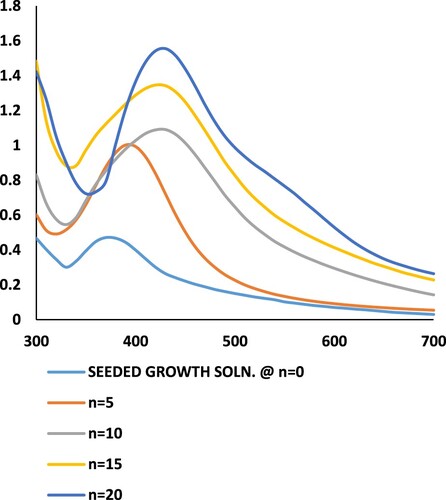
The seeded growth technique accompanied with mathematical modelling has been previously reported for controlled synthesis of metal nanoparticles by chemical synthesis where the precise chemical kinetics is known and, therefore, can be controlled [Citation37–39]. To the best of our knowledge, it is for the first time that mathematical modelling for green synthesis has been accomplished.
4. Conclusions
The mathematical model developed in this study will enable engineered synthesis of silver nanoparticles of desired size within a narrow size range by controlling the rate of reduction of the silver precursor salt. The rate of reaction was made high during seed synthesis to trigger burst nucleation, while controlled growth was achieved by limiting the rate of reaction to a moderate rate. The simplicity of the model will allow its application to silver nanoparticles synthesized using different leaf extracts also, enhancing the potential for the applicability of the “green” silver nanoparticles in various applications. More studies need to be carried out however to increase the homogeneity in the synthesized silver nanoparticles’ size. The stepped growth approach allows for greater control on tuning of the resultant size of the synthesized silver nanoparticles. A similar approach can also be extended to mathematically model the green synthesis of gold nanoparticles using other “green” reducing agents as well as for other metals nanoparticles. This will require, however, optimization studies for the rapid synthesis of stable nanoparticles for synthesizing seeds and conditions for a slower reduction rate of the metal salt to be used during growth stages.
Acknowledgements
Authors gratefully acknowledge Department of Science and Technology, Govt. of India for financial support (Women Scientist Scheme-A: SR/WOS-A/PS-46/2013).
Disclosure statement
No potential conflict of interest was reported by the author(s).
Additional information
Funding
References
- Cheon JY, Kim SJ, Rhee YH, et al. Shape-dependent antimicrobial activities of silver nanoparticles. Int J Nanomedicine. 2019;14:2773. doi: 10.2147/IJN.S196472
- Kinnear C, Moore TL, Rodriguez-Lorenzo L, et al. Form follows function: nanoparticle shape and Its Implications for nanomedicine. Chem Rev. 2017;117(17):11476. doi: 10.1021/acs.chemrev.7b00194
- Dwivedi N, Yeo RJ, Dhand C, et al. Boosting contact sliding and wear protection via atomic intermixing and tailoring of nanoscale interfaces. Sci Adv. 2019;5(1):eaau7886. doi: 10.1126/sciadv.aau7886
- Dorranian D, Solati E, Dejam L. Photoluminescence of ZnO nanoparticles generated by laser ablation in deionized water. Appl Phys A. 2012;109(2):307. doi: 10.1007/s00339-012-7073-5
- Hosseingholilou S, Dorranian D, Ghoranneviss M. Characterization of gold nanoparticle thin film prepared by electrophoretic deposition method. Gold Bull. 2020;53:1–10. https://doi.org/10.1007/s13404-020-00268-z.
- Kamali S, Solati E, Dorranian D D. Effect of laser fluence on the characteristics of graphene nanosheets produced by pulsed laser ablation in water. J Appl Spectrosc. 2019;86(2):238. doi: 10.1007/s10812-019-00806-4
- Solati E, Aghazadeh Z, Dorranian D. Effects of Liquid Ablation Environment on the Characteristics of TiO2 Nanoparticles. J Cluster Sci. 2019: 1–9. https://doi.org/10.1007/s10876-019-01701-w.
- Burduel AC, Gherasim O, Grumezescu AM, et al. Biomedical applications of silver nanoparticles: an up-to-date overview. Nanomaterials. 2018;8(9):681. doi: 10.3390/nano8090681
- Qi L, Zhang K, Qin W, et al. Highly efficient flow-through catalytic reduction of methylene blue using silver nanoparticles functionalized cotton. Chem Eng J. 2020;388:124252. doi: 10.1016/j.cej.2020.124252
- Mo L, Guo Z, Yang L, et al. Silver Nanoparticles Based Ink with Moderate Sintering in Flexible and Printed Electronics. International Journal of Molecular Sciences. 2019;20(9):2124–2151. doi: 10.3390/ijms20092124
- Chauhan N, Tyagi AK, Kumar P, et al. Antibacterial Potential of Jatropha curcas Synthesized Silver Nanoparticles against Food Borne Pathogens. Front Microbiol. 2016;7:1748–1760. https://doi.org/10.3389/fmicb.2016.01748.
- Savaloni H, Haydari-Nasab F, Abbas-Rohollahi A. Antibacterial effect, structural characterization, and some applications of silver chiral nano-flower sculptured thin films. J Theor Appl Phys. 2015;9:193. doi: 10.1007/s40094-015-0178-4
- Lateef A, Azeez MA, Asafa TB, et al. Biogenic synthesis of silver nanoparticles using a pod extract of<missing element: 562 Cola nitida: Antibacterial and antioxidant activities and application as a paint additive. J Taibah Univ Sci. 2016;10(4):551. doi: 10.1016/j.jtusci.2015.10.010
- Naika HR, Lingaraju K, Manjunath K, et al. Green synthesis of CuO nanoparticles using<missing element L. extract and their antibacterial activity. J Taibah Univ Sci. 2015;9(1):7. doi: 10.1016/j.jtusci.2014.04.006
- Sharma G, Soni R, Jasuja ND. Phytoassisted synthesis of magnesium oxide nanoparticles with<missing element: Swertia chirayaita. J Taibah Univ Sci. 2017;11(3):471. doi: 10.1016/j.jtusci.2016.09.004
- Dakshayani SS, Marulasiddeshwara MB, Kumar S, et al. Antimicrobial, anticoagulant and antiplatelet activities of green synthesized silver nanoparticles using Selaginella (Sanjeevini) plant extract. Int J Biol Macromol. 2019;131:787. doi: 10.1016/j.ijbiomac.2019.01.222
- Francis S, Nair KM, Paul N, et al. Catalytic activities of green synthesized silver and gold nanoparticles. Materials. Today Proc. 2019;9:97.
- de Souza TAJ, Souza LRR, Franchi LP. Silver nanoparticles: an integrated view of green synthesis methods, transformation in the environment, and toxicity. Ecotoxicol Environ Saf. 2019;171:691. doi: 10.1016/j.ecoenv.2018.12.095
- Jalili S, Hajakbari F, Hojabri A. Effect of silver thickness on structural, optical and morphological properties of nanocrystalline Ag/NiO thin films. J Theor Appl Phys. 2018;12:15. doi: 10.1007/s40094-018-0275-2
- Vankayala R, Kuo CL, Sagadevan A, et al. Morphology dependent photosensitization and formation of singlet oxygen (1Δg) by gold and silver nanoparticles and its application in cancer treatment. J Mater Chem B. 2013;1(35):4379. doi: 10.1039/c3tb20806k
- Ankamwar B, Kamble V, Sur UK, et al. Spectrophotometric evaluation of surface morphology dependent catalytic activity of biosynthesized silver and gold nanoparticles using UV–vis spectra: a comparative kinetic study. Appl Surf Sci. 2016;366:275. doi: 10.1016/j.apsusc.2016.01.093
- John J, Aravindakumar CT, Thomas S. SAJ Biotechnol. 2018;4(103):19.
- Villa-Ruano N, Pacheco-Hernández Y, Cruz-Durán R, et al. Seasonal variation in phytochemicals and nutraceutical potential of Begonia nelumbiifolia consumed in Puebla, México. J Food Sci Technol. 2017;54(6):1484. doi: 10.1007/s13197-017-2576-x
- Prathna TC, Chandrasekaran N, Raichur AM, et al. Kinetic evolution studies of silver nanoparticles in a bio-based green synthesis process. Colloids Surf A. 2011;377(1-3):212. doi: 10.1016/j.colsurfa.2010.12.047
- Thanh NT, Maclean N, Mahiddine S. Mechanisms of nucleation and growth of nanoparticles in solution. Chem Rev. 2014;114(15):7610. doi: 10.1021/cr400544s
- Contreras-Trigo B, Díaz-García V, Guzmán-Gutierrez E, et al. Slight pH fluctuations in the gold nanoparticle synthesis process influence the performance of the citrate reduction method. Sensors. 2018;18(7):2246–2254. https://doi.org/10.3390/s18072246.
- Mukha I, Vityuk N, Severynovska O, et al. The pH-dependent stucture and properties of Au and Ag nanoparticles produced by tryptophan reduction. Nanoscale Res Lett. 2016;11(1):1–7. doi:10.1186/s11671-016-1318-8.
- Fernando I, Zhou Y. Impact of pH on the stability, dissolution and aggregation kinetics of silver nanoparticles. Chemosphere. 2019;216:297. doi: 10.1016/j.chemosphere.2018.10.122
- Jain A, Malik A, Malik HK. 22nd International Conference and Expo on “Nanoscience and Molecular Nanotechnology”, Frankfurt, Germany. Abstract-p 79; 2017.
- Tripathy A, Raichur AM, Chandrasekaran N, et al. Process variables in biomimetic synthesis of silver nanoparticles by aqueous extract of Azadirachta indica (Neem) leaves. J Nanopart Res. 2010;12(1):237. doi: 10.1007/s11051-009-9602-5
- Samuolienė G, Sirtautas R, Brazaitytė A, et al. Supplementary red-LED lighting and the changes in phytochemical content of two baby leaf lettuce varieties during three seasons. J Food Agric Environ. 2012;10(3-4):701–706.
- Gololo SS, Shai LJ, Agyei NM, et al. Effect of seasonal changes on the quantity of phytochemicals in the leaves of three medicinal plants from Limpopo province, South Africa. J Pharmacognosy Phytother. 2016;8(9):168–172. doi:10.5897/JPP2016.0408.
- Sati P, Pandey A, Rawat S, et al. Phytochemicals and antioxidants in leaf extracts of Ginkgo biloba with reference to location, seasonal variation and solvent system. J Pharmacy Res. 2013;7(9):804. doi: 10.1016/j.jopr.2013.09.001
- Gharibshahi L, Saion E, Gharibshahi E, et al. Structural and optical properties of Ag nanoparticles synthesized by thermal treatment method. Materials. 2017;10(4):402. doi: 10.3390/ma10040402
- Haiss W, Thanh NT, Aveyard J, et al. Determination of size and concentration of gold nanoparticles from UV−Vis spectra. Anal Chem. 2007;79(11):4215. doi: 10.1021/ac0702084
- Paramelle D, Sadovoy A, Gorelik S, et al. A rapid method to estimate the concentration of citrate capped silver nanoparticles from UV-visible light spectra. Analyst. 2014;139(19):4855–4861. doi: 10.1039/C4AN00978A
- Leng W, Pati P, Vikesland PJ. Room temperature seed mediated growth of gold nanoparticles: mechanistic investigations and life cycle assessment. Environ Sci Nano. 2015;2(5):440. doi: 10.1039/C5EN00026B
- Niu J, Zhu T, Liu Z. One-step seed-mediated growth of 30–150 nm quasispherical gold nanoparticles with 2-mercaptosuccinic acid as a new reducing agent. Nanotechnology. 2007;18(32):325607. doi: 10.1088/0957-4484/18/32/325607
- Peng HC, Park J, Zhang L, et al. Toward a quantitative understanding of symmetry reduction involved in the seed-mediated growth of Pd nanocrystals. J Am Chem Soc. 2015;137(20):6643. doi: 10.1021/jacs.5b03040