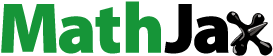
Abstract
The purpose of this study was to develop a self-nanoemulsifying drug delivery system (SNEDDS) for the improvement of solubility and bioavailability of luteolin in order to enhance its therapeutic benefits. Formulations were prepared by spontaneous emulsification method which involved low energy mixing of appropriate amounts ofcastor oil, kolliphor & polyethylene glycol 200. The developed formulae were optimized and characterized for self-nanoemulsifying capacity, thermodynamic stability, size behaviour and solubility. The globule size of the optimized formula F1–A1:1 was 112 ± 15 nm, with narrow PDI (0.31 ± 0.09) and good zeta potential (−16.2 ± 3.2 mV). The optimized formula exhibited approximately 83, 17 and 3-fold enhancement in the solubility in vitro release and ex vivo permeation respectively. The luteolin SNEDD exhibited better antioxidant activity (DPPH scavenging activity) than ascorbic acid. Per cent inhibition in rat paw oedema by the SNEDDS formulation was statistically significant when compared with luteolin suspension at equivalent dose (P < 0.05).
1. Introduction
Luteolin is a yellow coloured pigment present in different parts of various plants, herbs and dietary sources [Citation1]. The common sources of luteolin include oregano, Origanum vulgare (Lamiaceae); Mexican oregano, Lippia graveolens (Verbenaceae) and celery, Apium graveolens (Apiaceae) [Citation1,Citation2]. Chemically, luteolin is a polyphenolic natural material that belongs to a group of compounds called flavonoids [Citation2]. It is a small molecule with a molar mass of 286.24 gmol−1 and a molecular formula of C15H10O6 containing a flavone or phenyl chromone ring (Figure ). It has been reported to have various pharmacological activities such as anti-oxidative [Citation1–7], anti-allergic [Citation8–12], and anti-inflammatory [Citation13–18], anti-cancer [Citation19–24] and anti-diabetic activities [Citation25–27]. However, it is reported to have low aqueous solubility [Citation28,Citation29]. Poorly water-soluble drugs often have a low or erratic absorption leading to low oral bioavailability with high variability [Citation30]. The oral bioavailability of luteolin in the rats was reported to be <30% [Citation31,Citation32]. The poor water solubility and poor oral bioavailability of luteolin limit its clinical applications despite having several beneficial pharmacological activities. Therefore, overcoming the poor solubility is a critical process to improve bioavailability and thus therapeutic efficacy and clinical benefits.
There are several reports of enhancement of solubility of luteolin in order to enhance the bioavailability and efficacy. For instance, inclusion complex formation of luteolin with cyclodextrins improved the antioxidant activity of luteolin which could be due to improved solubility and bioavailability of luteolin [Citation33,Citation34]. Similarly, complexation of luteolin with phospholipids is reported to improve cellular uptake, bioavailability and efficacy of luteolin [Citation35–37]. Nanofabrication and nano-encapsulations have also been reported with enhanced solubility, bioavailability and efficacy of luteolin. For instance, nanoencapsulation of luteolin in liposomes [Citation38–41], micelles [Citation42–45] and nanoparticles [Citation46] were reported to improve solubility/bioavailability and efficacy as compared to pure luteolin. Self-nanoemulsifying drug delivery systems (SNEDDS) are promising carriers for the delivery of poorly water-soluble drugs. These are isotropic mixtures of oil, emulsifier and co-emulsifier which form oil-in-water nanoemulsion when diluted with water [Citation47]. SNEDDS are known to improve solubility, dissolution [Citation48–53], bioavailability [Citation54–57] and therapeutic benefits of loaded drugs [Citation58,Citation59].
In this paper, we are reporting, SNEDDS to enhance solubility and efficacy of luteolin. There is no report of luteolin-loaded SNEDDS to the best of our knowledge.
2. Materials and methods
2.1. Materials
Luteolin, ascorbic acid and 2,2-diphenyl-1-picrylhydrazyl were purchased from Sigma–Aldrich (St Louis, MO, USA). The oils (castor oil, oleic acid, isopropyl maleate, sesame oil) emulsifiers (Tween 20, Tween 80 and kolliphor) and co-emulsifiers/co-solvents (polyethylene glycol 200, propylene glycol, diethylene glycol monoethyl ether, ethanol and butanol) were also purchased from Sigma–Aldrich (St. Louis, MO, USA). Kolliphor was purchased from BASF (Ludwigshafen, Germany). Ultrapure water was produced by Milli-Q, Merck, MA, USA.
2.2. Methods
Methods adopted were qualitative and quantitative experiments wherein the influence of independent variables viz; types and amount of oils, emulsifiers and co-emulsifiers/co-solvents on the dependent variable such as appearance, dispersability, globule size, polydispersity index, zeta potential and thermodynamic stability of SNEDDS were investigated.
2.3. Screening of excipients for luteolin-loaded SNEDDS
Excipients for SNEDDS development were screened and selected based on maximum saturated solubility of the drug in the emulsifier, co-emulsifier and oil phases tested [Citation60]. Excess amount of luteolin was shaken in a thermostatic shaker at 37°C for 72 h, with various oils (castor, sesame, oleic acid, isopropyl maleate), emulsifiers (Tween 20, Tween 80, kolliphor) and co-emulsifiers (ethanol, butanol, PEG 200, propylene glycol and diethylene glycol monoethyl ether). Suspensions were centrifuged at 10,000 × rcf for 10 min to separate the supernatants (Centurian Scientific, UK) [Citation61,Citation62]. The amount of luteolin in the supernetants were quantified by UV spectrophotometer (V 630 Jasco, Japan) at λmax of 284 nm after appropriate dilution.
2.4. Development of SNEDDS
The development of SNEDDS was based on the maximum equilibrium solubility of luteolin as obtained in the phase solubility studies of oil phases, emulsifiers and co-emulsifiers. Based on the results of solubility studies, castor oil, kolliphor and PEG 200 were chosen as oil, emulsifier and co-emulsifier/co-solvent, respectively. Different SNEDDS were prepared by mixing varying amount of oil phase (10–50% v/v) with the varying amount (90–50% v/v) of various ratio (1:1, 2:1 and 1:2) of emulsifier:co-emulsifier/co-solvent as shown in Table . Briefly, the first formula was prepared by mixing 10% v/v of oil (0.5 ml) and 90% v/v of emulsifier (4.5 µl of emulsifier mixture containing different ratios, such as 1:1, 1:2, 2:1 of emulsifier:co-emulsifier). Alternative formulations were prepared with increasing volumes of oils (20, 30, 40 and 50% v/v) and decreasing volumes of emulsifier mixtures (80, 70, 60, 50% v/v). A fixed amount of luteolin (10 mg/ml) was incorporated in all mixtures and vortexed for 60 s to prepare luteolin-loaded preconcentrate of SNEDDS.
Table 1. Composition of luteolin-SNEDDS.
2.5. Optimization of SNEDDS
The optimization of formulated SNEDDS was performed based on the dispersability or self-nanoemulsifying capacity, droplet characterization (droplet size, size distribution and zeta potential), thermodynamic stability and capacity to hold the maximum amount of luteolin. The optimized SNEDDS were selected for further characterization and investigations such as viscosity, conductivity, pH and refractive indices, surface characterization, in vitro release behaviour, permeability across the intestinal sacs and anti-inflammatory activity.
2.6. Self-nanoemulsifying capacity of SNEDDS
All prepared SNEDDS were first tested for self-nanoemulsifying capacity by investigating dispersability with the help of dissolution apparatus 2 DT 720 (Erweka, Langen, Germany). Two millilitres of each SNEDDS were added in 200 ml of 0.01 N HCl kept in dissolution basket at 37 ± 0.5°C. Gentle mixing was done by rotating the paddle at 50 rpm. The self-nanoemulsifying capacity was graded as A through E by visually observing the appearance of nanoemulsions formed and time of nanoemulsification [Citation63].
2.7. Optical clarity test of SNEDDS
The optical clarity of SNEDDS was evaluated by measuring % transmittance of the formulation by using double beam spectrophotometer (V 630, Jasco, Japan). Each formulation was diluted 100 times with distilled water and analysed spectrophotometrically at 650 nm using distilled water as blank [Citation64].
2.8. Size, polydispersibility and zeta potential evaluation of SNEDDS
The size, polydispersibility and zeta potential of SNEDDS were evaluated by dynamic light scattering (DLS) technique by using Zetasizer Nano ZS, model ZEN3500 (Malvern Instruments, UK) [Citation65]. The preconcentrates were diluted (100×) with 0.01 N HCl (pH 2.0), and transferred to the disposable plastic cuvette (∼3 ml). The droplet size and polydispersity index were analysed at 25°C keeping the measurement in automatic mode. The droplet size and size distribution of the dispersion are recorded as Z-average value (below equation), an intensity-based harmonic mean size and PDI, respectively.
(1)
(1) where Dz is the Z-average diameter of droplets, ∑Si the cumulative scattered intensity from droplets i and ∑Di the cumulative diameter of droplets i.
For the measurement of zeta potential, the preconcentrates were diluted (100×) with 0.01 N HCl (pH 2.0), mixed gently and 800 µl of this mixture was then transferred to zeta cells (Model DTS1017, Malvern Instruments). The zeta cell is then capped and inserted in zetasizer (Zetasizer nanoZS Malvern UK), and the mean zeta potential was then evaluated after three runs at 25°C keeping the measurement in automatic mode and fitting the Henrys function to the Smoluchowski approximation (below equation)
(2)
(2) where
is the electrophoretic mobility, z the zeta potential, ε the dielectric constant, f(k a)the Henrys function and
the viscosity.
2.9. Thermodynamic stability evaluations of SNEDDS
The luteolin-loaded preconcentrate SNEDDS formulations with sufficient self-nanoemulsifying capacity and excellent clarity upon dilution were subjected to thermodynamic evaluations for further optimization [Citation66]. Selected formulations were centrifugated at 4000 rpm for 15 min (Centurian Scientific, UK). The visual observation was done to screen out any metastable formulation showing any change in the appearance such as turbidity, creaming or cracking etc. The stable dispersions showing no change were exposed to three cycles of accelerated stressed conditions such as cooling–heating at 4° and 45°C then freezing–thawing at −20° and 25 ± 2 °C each for 48 h.
2.10. Saturation solubility of luteolin in SNEDDS
The saturation solubility of luteolin in the prepared SNEDDS was investigated by shake flask method [Citation67]. Approximately 200 mg of luteolin was incorporated in selected SNEDDS, vortex mixed for 30 s then horizontally shaken at 100 rpm for 48 h on the biological shaker set at 37°C (LBS-030S-Lab Tech, Korea). The supernatants were separated by centrifugating the suspensions at 10,000 × rcf for 10 min (refrigerated centrifuge, Centurian, UK) [Citation61,Citation62], and the amount of luteolin was quantified by the UV spectrophotometer at 284 nm (V 630, Jasco, Japan).
2.11. Characterization of SNEDDS
The selected formulations with optimum properties were characterized for viscosity, conductivity, pH and refractive index [Citation60]. The viscosity of the formulation was examined by using Brookfield DV I prime CP viscometer (Middleboro, MA, USA). One millilitre of formulation was introduced in the plate and viscosity is measured in triplicate at 25°C by using cone spindle CPE42 rotating at a speed of 20 RPM.
The refractive index of the formulation was examined by Rudolph Refractrometer J 257 (Rudolph, Japan), equipped with an easy to clean ultra flat, scratch-proof hard sapphire prism. The refractrometer was autozeroed by using few drop of distilled water. The sample holder is cleaned by wiping with a paper towel and three drops of SNEDDS were introduced and measured in triplicate at 25°C.
The conductivity of SNEDDS was studied by the benchtop Conductivity meter (4520, Jenway UK). The glass conductivity probe was immersed in a glass test tube containing ∼5 ml of SNEDD dispersions (diluted 100× with distilled water) and conductivity was measured in triplicate at 25°C.
The pH measurement of SNEDDS formulation was done by the benchtop pH meter (Sensodirect PH 200, Lovibond UK). The electrodes of the pH meter were immersed in a glass test tube containing ∼5 ml of SNEDD dispersions (diluted 100× with distilled water) and pH was measured in triplicate at 25°C.
The surface characterization of the optimized SNEDDS was carried out by Transmission Electron Microscopy (TEM) (JEOL, JEM 1011F, MA, USA). A drop of SNEDDS formulation was diluted (100×) with double-distilled water and deposited on the holey film grid, air dried for 30 s and then observed under TEM operating at 80 kV [Citation60].
2.12. In vitro release study
In vitro release study of selected SNEDDS formulation and pure luteolin was performed by the dialysis bag technique [Citation68]. One millilitre of SNEDDS or aqueous suspension containing equivalent amount of luteolin (20 mg/ml) was filled in dialysis bags (surface area 23.55 cm2) made up of regenerated cellulose (MWCO 12,000 Da, Sigma–Aldrich, St Louis, MO, USA) previously soaked in 0.1 N HCl overnight. Dialysis bags were suspended in glass vessels containing 50 ml of 0.1 N HCl maintained at 37°C in a thermostated biological shaker rotating at 100 rpm. Three millilitres of samples were withdrawn at 0, 15, 30, 45, 60 and 120 min time intervals, and the fresh medium was replaced after each sampling. The sample was diluted appropriately and measured by the double beam spectrophotometer at 284 nm in triplicate.
2.13. Ex vivo permeation study
Ex vivo permeation of luteolin suspension and luteolin-loaded optimized SNEDDS formulation was carried out by using non-everted gut sac method [Citation69]. Male Wistar albino rat, weighing ∼250 g, was provided by the Animal House of College of Pharmacy, Prince Sattam bin Abdulaziz University. Animal was fasted with free access to water overnight before it was sacrificed by cervical dislocation. A midline longitudinal incision of ∼3 cm was made to locate the small intestine. The small intestine was removed and put into a Petri dish containing physiological Tyrode solution. The intestine is cleaned by removing the content and then washed carefully by pushing oxygenated Tyrode solution with the help of a syringe equipped with a blunt needle. Approximately 5 cm long pieces of washed intestinal tract were cut to prepare the intestinal sacs. Firstly, one side of the intestine was tied tightly with thread, followed by filling of 0.5 ml of treatment solution/suspension (equivalent to 10 mg of luteolin) and tying of the other end tightly with thread. Filled intestinal sacs were measured as 3 ± 0.2 cm long with 5 ± 0.5 mm of diameter. The prepared intestinal sacs were placed in a glass conical flask containing 50 ml of oxygenated Tyrode buffer maintained at 37°C in a thermostated water bath shaking at 100 rpm. Approximately 3 ml of samples was withdrawn at 0, 10, 20, 30, 60, 90 and 120 min from the flask and replaced with fresh Tyrode solution. The concentration of luteolin in the samples was then measured spectrophotometrically in triplicate [Citation70,Citation71]. The cumulative amount permeated per unit area of sac (µg/cm2) was calculated and plotted against time of permeation to construct the permeation graph. The slope of the permeation graph was calculated to determine the flux of luteolin (µg/cm2/min) across the intestinal sac. The permeability of luteolin across the rat intestine was calculated by using the equation as follows:
(3)
(3) where Papp is the apparent permeability, J the flux of luteolin across the intestinal sac and C the initial concentration of luteolin loaded in the intestinal sac.
2.14. Antioxidant activity (DPPH scavenging assay)
The antioxidant activity of the optimized formulation was compared with pure luteolin and ascorbic acid, a standard antioxidant, by using 2,2′-diphenyl-1-picryl hydrazyl as reported (DPPH assay) with slight modification [Citation72]. The standard solution of 0.1 mM DPPH was prepared by dissolving 4 mg of DPPH in 100 ml of methanol. The test solutions of luteolin-loaded SNEDD, luteolin and ascorbic acid were prepared by serial dilution within the concentration range of 1–100 µg/ml. An aliquot of each dilution (0.5 ml) was added separately into 2 ml of 0.1 mM DPPH solution to prepare a reaction mixture in triplicate. The blank mixture (control) was prepared by adding 0.5 ml of methanol in 2 ml of 0.1 mM DPPH solution. All reaction mixtures were incubated in the dark for 30 min at ambient temperature (25°C). The absorbance of reaction mixtures was recorded at 517 nm by means of a UV/VIS spectrophotometer (V-630, JASCO International Co. Ltd, Hachioji, Tokyo, Japan). The % antioxidant activity (% DPPH scavenging) of samples was calculated by using the equation as follows:
(4)
(4) where Ac is the absorbance of the control (blank) and At the absorbance of the test samples (luteolin or ascorbic acid).
The % antioxidant activity was plotted against the concentration of the luteolin or ascorbic acid to observe the dose-dependent inhibition or scavenging behaviour. The IC50 of DPPH scavenging was calculated by using Quest Graph™ IC50 calculator based on four-parameter logistic regression model [Citation73]. The obtained experimental data were subjected to statistical evaluation using one-way analysis of variance (ANOVA) followed by post hoc Tukey test for multiple comparison [Citation74]. A value of P < 0.05 was considered as statistically significant difference between groups.
2.15. In vivo anti-inflammatory activity
Anti-inflammatory activity of the optimized formulation was compared with pure luteolin suspension using rat hind paw oedema model [Citation75]. The animal study was approved by the ethical committee of Prince Sattam bin Abdulaziz University (Approval number-BERC-001-05-19), and conducted according to the science and ethical principles for animal care, at the college of pharmacy, Prince Sattam bin Abdulaziz University. The Wistar Albino rats within a weight range of 180–250 g were kept on fasting overnight with free access to water and adapted to standard laboratory conditions at a temperature of 25 ± 1°C before the commencement of study. Single dose, fasting state parallel design was followed for this study; therefore, 12 rats were randomly selected and separated into four groups of three animals each. Acute inflammation was induced by injecting 0.1 mL of 1% w/v carrageenan in normal saline in the left hind paw of all the rats in each group. Animals in the group one were given vehicle only (0.5% w/v aqueous sodium carboxymethyl cellulose, SCMC) that served as the negative control. Animals in the remaining three groups were administered with different treatments prepared in 0.5% SCMC, at a fixed dose of 10 mg/kg body weight according to their weight, 30 min prior to the induction of inflammation. Briefly, animals in the group two were gavaged with SNEDDS formulation containing luteolin equivalent to 10 mg/kg body weight. Animals in group three were gavaged with pure drug, luteolin, equivalent to 10 mg/kg body weight as a comparator to the luteolin-loaded SNEDD formulation. Animals in group four were gavaged with indomethacin at a dose of 10 mg/kg body weight as a standard therapy or positive control. The paw oedema was recorded at definite time intervals (0, 1, 2 and 4 h) by using the plethysmometer. The anti-inflammatory effect of the treatments was calculated as the per cent inhibition of oedema compared with the control group by using the following formula:
(5)
(5) where C and T are the mean paw oedema of animals in the control group and treatment group, respectively.
2.16. Statistical evaluation
The obtained experimental data were subjected to statistical evaluation using one-way ANOVA followed by post hoc Tukey test for multiple comparison [Citation74]. The value of P < 0.05 was considered as statistically significant difference between groups.
3. Results and discussions
3.1. Preparation of luteolin-loaded SNEDDS
The preparation of luteolin-loaded SNEDDS employed spontaneous emulsification technique utilizing low energy and simple vortex mixing of the SNEDDS components such as luteolin, oil phase, emulsifier and co-emulsifier. The optimum formula for the preparation of a good SNEDDS requires mixing of a specific type and optimized proportions of oil, emulsifier and co-emulsifiers. In general, the selection of components was based on the ability to solubilize the drug to be loaded. Furthermore, the ability of emulsifying agent to emulsify the oil in water and the ability of co-emulsifying agent to decrease the interfacial tension were some other critical processess that help in selection of optimum components of SNEDDS. The SNEDDS components viz. oil, emulsifier and co-emulsifier/co-solvent, once selected and combined together in an optimum proportions formed an excellent self-nanoemulsifying preconcentrate which upon simple dilution with the aqueous phase resulted in clear, transparent, isotropic mixture of oil and water. A slight change in the optimized type and proportion of any component of the SNEDDS degrade the quality of the preparation, that result in translucent, bluish, hazy, unclear or milky appearance upon dilution with the aqueous phase.
3.2. Phase solubility evaluation of luteolin
Phase solubility evaluation of luteolin employed analysis of supernatant obtained from different vehicles such as oil, emulsifier and co-emulsifier spectrophotometrically at 284 nm by using linear equation of the calibration plot of luteolin. A calibration plot was found linear in the range of 1–100 µg/ml with a correlation coefficient of 0.999. The mean equilibrium solubility of luteolin is presented in Table . The solubility of luteolin in water was found to be 0.85 ± 0.03 mg/ml which is approximately similar as reported [Citation28,Citation29]. Drugs having aqueous solubility <1 mg/ml are considered as very slightly soluble or poorly soluble drugs [Citation76,Citation77]. Poor aqueous solubility of drug is considered as the main cause of poor bioavailability of several drugs [Citation78].
Table 2. Mean equilibrium solubility of luteolin in SNEDD excipients.
3.3. Screening and selection of excipients for SNEDDS of luteolin
Excipients for the development of luteolin-loaded SNEDDS were screened and selected on the basis of saturated solubility of luteolin in various phases such as oil phase (castor, sesame, oleic acid, isopropyl maleate), emulsifier phase (Tween 20, Tween 80 and kolliphor) and co-emulsifiers (ethanol, butanol, PEG 200, propylene glycol and diethylene glycol). Based on the maximum equilibrium solubility of luteolin, as evaluated in the phase solubility studies, castor oil, kolliphor and polyethylene glycol were chosen as oil, emulsifier and co-emulsifier, respectively, as shown in Figure .
3.4. Development of SNEDDS
The development of SNEDDS was based on the maximum equilibrium solubility of luteolin obtained in the particular oil phase, emulsifier and co-emulsifier. Based on solubility data, castor oil, kolliphor and PEG 200 were selected as oil, emulsifier and co-emulsifier /co-solvent, respectively. Some group of components such as castor versus sesame oil, Tween 80 versus kolliphor and PEG 200 versus diethylene glycol monoethyl ether had approximately similar equilibrium solubilities. In a preliminary study, sesame oil was used as an alternative to castor oil, which did not result in clear nanoemulsion upon dilution with water. Similary, Tween 80 was tried instead of kolliphor as an emulsifier, but it did not result in clear nanoemulsion. Likewise, diethylene glycol monoethyl ether was also explored as co-emulsifier /co-solvent in place of PEG 200, but it also did not result in clear nanoemulsion. Therefore, development of SNEDDS was performed by using castor oil, kolliphor and PEG 200. Different amount of castor oil was mixed with different amount of emulsifier mixtures containing different propositions of kolliphor and PEG 200 as shown in Figure . All the mixtures were mixed gently by using vortex mixer for 30 s to prepare preconcentrate of possible SNEDDS.
3.5. Optimization of SNEDDS
The optimization of prepared SNEDDS was achieved based on self-nanoemulsifying capacity, rapid dispersability, desired droplet properties (droplet size, size distribution and zeta potential) thermodynamic stability and capacity to solubilize the maximum amount of luteolin.
3.6. Self-nanoemulsifying capacity of SNEDDS
All prepared formulations were first subjected to dispersibility testing in order to optimize the SNEDDS for luteolin based on self-nanoemulsifying capacity of the formula. The dispersibility test was performed in 0.01 N HCl (pH 2.0) to simulate the dilution of the SNEDDS in the acidic environment of gastric fluid. All the prepared formulations were dispersed rapidly by gentle mixing. However, all the formulae did not produce clear (C) isotropic nanoemulsion, rather, some formulae resulted in bluish, slightly whitish or milky emulsions as shown in Figure . Self-nanoemulsifying capacity and resulting nanoemulsions are graded as A, B, C, D and E, based on their visual appearance and time of emulsification [Citation63]. A grade SNEDDS formulations were rapidly self-emulsified within a minute when diluted with 0.01 N HCl and formed clear nanoemulsions. Grade B SNEDDS were also rapidly emulsified within a minute but resulted in slightly less clear nanoemulsion with bluish appearance. Grade C SNEDDS were emulsified within 2 min with slightly milky nanoemulsions. Grade D SNEDDS took more than 2 min to be emulsified and resulted in milky nanoemulsions. E grade denotes poor SNEDDS that exhibit no or minimal emulsification with large oil droplets present on the surface [Citation63].
Figure 4. Self-nanoemulsifying capacity and dispersability grades of luteolin-SNEDDS; A11–F11, A21–E21 and A12–C12 denote different formulation prepared by using 1:1, 2:1 and 1:2 ratio of emulsifier:co-emulsifier, respectively. (a) Rapidly self-emulsified within a minute with clear nanoemulsions, (b) rapidly emulsified within a minute with bluish appearance, (c) emulsified within 2 min with slightly milky nanoemulsions and (d) emulsified after 2 min resulted in milky nanoemulsions.
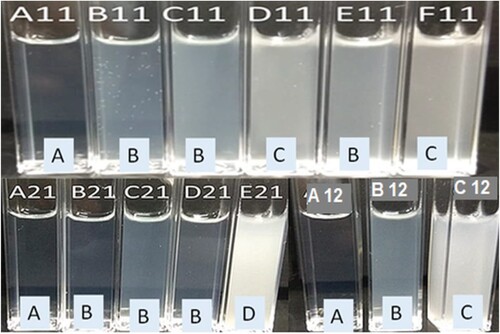
3.7. Optical clarity test of SNEDDS
Orally administered SNEDDS formulations are supposed to self-nanoemulsify in gastric juice and result in transparent nanoemulsion. Therefore, the optical clarity of SNEDDS formulations diluted in 0.01 N HCl was evaluated by measuring % transmittance to screen the optimum SNEDDS formula. Formulations considered as grade A in dispersibility or self-nanoemulsifying testing exhibited transmittance over 95% due to the transparent nature of nanoemulsions, as shown in Figure . Grade B and grade C SNEDDS formulations exhibited transmittance between 80–95% and 60–70%, respectively, while grade D formulations had transmittance values below 50%. Transmittance values closer to 100% are indicative of small globule size in the nanoemulsions while lower transmittance values indicate larger globule size with poor nanoemulsions. Based on dispersibility, self-nanoemulsifying capacity and optical clarity test, formulation F1–A11, F8–A21 and F13–A12 were selected for further characterization and evaluations (Figure ).
3.8. Size, polydispersibility and zeta potential evaluation of SNEDDS
The size, polydispersibility and zeta potential of SNEDDS were measured by DLS technique. The DLS technique employs the measurement of variation in light scattered due to different size of droplets in Brownian motion. As smaller droplets diffuse faster when compared with larger ones, they, therefore, result in more rapid fluctuations in scattering which are captured by the photon counter [Citation65]. The globule size, polydispersity index and zeta potential are presented in Figure . All the SNEDDS resulted in nanoemulsions (globule size <1 µm) when diluted 100 times with water or 0.01 N HCl with the polydispersity index between 0.3 and 0.9. The zeta potential of all the formulations ranged within −3 to −16 mV. The lower and negative zeta potential values could be due to the presence of fatty acid esters in the castor oil used. The globule size of SNEDDS was influenced by both amount of oil and emulsifiers. Moreover, it was also affected by the proportion of co-emulsifier in the emulsifier mixture. An increase in the amount of castor oil with respect to emulsifier kolliphor resulted in larger globules and vice-versa (formulation F1 through F8). Similarly, an increase in co-emulsifier PEG 200 with respect to emulsifier kolliphor also resulted in larger globules even with fixed amount of castor oil (formulation F9–F11 versus F13–F15). This could be due to lower emulsifying capacity of co-emulsifier PEG 200 when compared with emulsifier kolliphor. Smaller globule size of nanoemulsions are considered as better than the larger counterpart as smaller globules provide more interfacial surface area for drug release and absorption.
3.9. Thermodynamic stability evaluations of SNEDDS
The SNEDDS with dispersibility grade A and B were subjected to three cycles of various stressed conditions such as centrifugation, heating/cooling and freezing/thawing in order to evaluate the thermodynamic stability of these formulations to choose the most stable optimum formula. The purpose of these tests was to screen out any metastable formulation that would be destabilized during the storage period. The metastable formulation, if any, would show signs of instability or phase separation such as creaming, flocculation, cracking or coalescence [Citation79]. All tested SNEDDS remained stable and did not show any sign of phase separation after thermodynamic stress testing.
3.10. Saturation solubility of luteolin in SNEDDS
The saturation solubility of luteolin in the prepared SNEDDS was evaluated to select the optimum SNEDDS capable of solubilizing the maximum amount of luteolin. Only stable formulations having transmittance over 85% and established as grade A or B in dispersibility test were selected for solubility study. The saturation solubility of luteolin in different SNEDDS is presented in Figure . Formulation F1, with a composition of 10% castor oil, 45% kolliphor and 45% PEG 200, exhibited maximum saturation solubility of luteolin (72.46 ± 0.16 mg/ml) which is∼83-fold higher than those observed in water (0.85 ± 0.04 mg/ml). Formulations with increasing amount of castor oil (F2, F3 and F8) exhibited decreasing solubility of luteolin as solubility of luteolin in castor oil (1.91 ± 0.02 mg/ml) was substantially lower than those observed in kolliphor (21.03 ± 0.16 mg/ml) or PEG 200 (26.08 ± 0.57 mg/ml). A similar trend was exhibited by other formulations such as F8 through F10 and F13 versus F14. Different proportion of emulsifier and co-emulsifier in the emulsifier mixture also influenced the saturated solubility of the luteolin. Formulations (F8 and F9) with higher proportion of emulsifier with respect to co-emulsifier (2:1) exhibited lower saturated solubility of luteolin compared with formulations (F13 and F14) which contained lower proportions of emulsifier with respect to co-emulsifier (1:2). This could be explained due to lower solubilizing capacity of emulsifier with respect to co-emulsifier. However, it was noteworthy that formulation F1 with 1:1 mixture of emulsifier:co-emulsifier exhibited better drug solubility than F13 which contained 1:2 mixture of emulsifier:co-emulsifier. This could be possibly due to larger interfacial area provided by 1:1 mixture compared with 1:2 mixture. Nevertheless, one formulation from each group emulsifier mixtures, i.e. 1:1 (F1–A1;1), 2:1 (F8–A2;1) and 1:2 (F13–A1;2), was selected for further characterization and investigations.
3.11. Characterization of SNEDDS
The formulations optimized on the basis of nanoemulsifying capacity, thermodynamic stability, saturated solubility of luteolin and globule size analysis were selected for further characterization and investigations. Some parameters such as viscosity, refractive index, conductivity and pH of selected formulations are presented in Table . Low viscosity of SNEDD formulations are desirable as it makes pourable and facilitate in administration [Citation80]. The refractive indices of formulation were very close to that observed with distilled water indicating transparency and optical clarity of the SNEDD dispersions [Citation81]. The high electrical conductivity of the SNEDD formulations indicated o/w nanoemulsions with ultralow interfacial tension due to the formation of bicontinuous or solution type systems [Citation82]. The acidic pH of the SNEDD formulations would be beneficial for the long-term stability of luteolin as luteolin degrades faster in basic medium [Citation83]. The size and surface characterization of the optimized SNEDDS was carried out by transmission electron microscopy (TEM), as shown in Figure . The nanoemulsion droplets were found spherical and in nanorange.
Figure 8. Transmission electron microscopy photograph of optimized luteolin-SNEDDS (F1–A1:1) at 50,000× magnification, by JEOL (JEM 1011F, MA, USA).
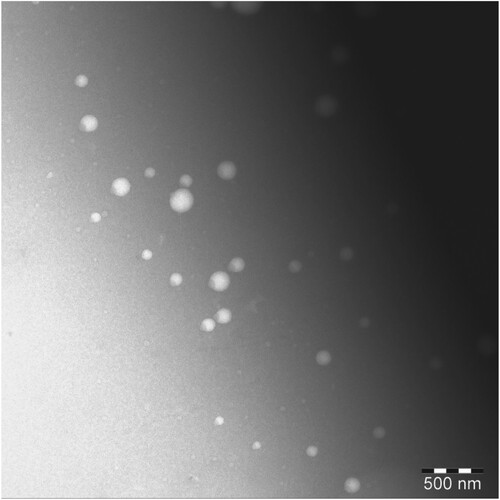
Table 3. Characterization of selected luteolin-SNEDDS.
3.12. In vitro release and ex vivo permeation studies
In vitro release behaviour of luteolin form selected SNEDDS was compared with luteolin suspension. Release profile of luteolin form luteolin suspension and optimized formulations are presented in Figure (a). Luteolin suspension exhibited only 3.2% release of luteolin during 120 min. Slow release of luteolin may be attributed to the hydrophobic nature of the drug as well as small volume of release medium. Moreover, dissolution medium with more acidic nature is known to slow the dissolution when compared with weakly acidic or neutral dissolution medium [Citation84]. Formulation F1–A1:1 and F8–A2:1 released approximately 55% during 120 min while formulation F13–A1:2 exhibited about 38% in the same time period. Therefore, formulation F1–A1:1 and F8–A2:1 exhibited about 17-fold enhancement while F13–A1:2 exhibited 12-fold enhancement in the in vitro release when compared with luteolin suspension.
Figure 9. In vitro release profiles across regenerated cellulose dialysis bag (% release ± SD, n = 3) (a) and ex vivo permeation profiles across non-everted rat sacs (µg/cm2 ± SD, n = 3) of luteolin from luteolin suspensions and luteolin-SNEDDS.
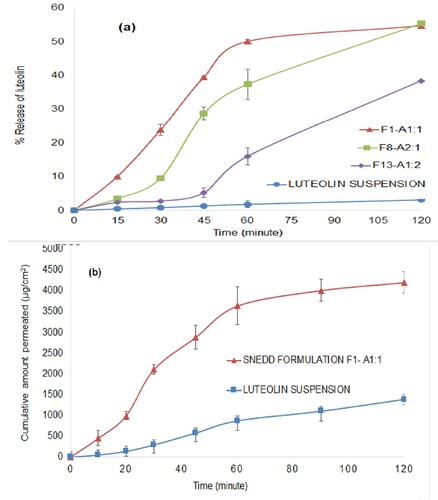
The enhanced release of luteolin from SNEDDS formulations may be attributed to better solubilizing potential of the components of SNEDD when compared with water in the luteolin suspension [Citation85].
Formulation F1–A1:1 was selected for ex vivo permeation study as it gave consistently higher in vitro release compared to other formulations. Ex vivo permeation of luteolin through the rat intestinal sac is shown in Figure (b). The optimized SNEEDS exhibited ∼3-fold more permeation across the rat intestine when compared with drug suspension during the study period. Apparent permeability of luteolin suspension and luteolin-loaded SNEDDS across the rat intestine was found to be 1.03 × 10−5 and 2.8 × 10−5cm/s, respectively. The components of SNEDD along with drug enclose within superfine oil droplets with an enhanced surface area responsible for better permeation of drugs from SNEDD when compared with drug alone [Citation86].
3.13. Antioxidant activity (DPPH radical scavenging activity)
The concentration-dependent DPPH radical scavenging activity of the formulation in comparison to luteolin and a standard antioxidant (ascorbic acid) was performed within a concentration range of 1–100 µg/ml. As the luteolin or ascorbic acid was further diluted by five times in all the reaction mixtures during assay procedure, the final concentration of luteolin or ascorbic acid range varied from 0.02 to 20 µg/ml, as shown in Figure . The one-way ANOVA and post hoc Tukey test was performed to find out the difference among the luteolin, formulations or ascorbic acid. Luteolin and luteolin-loaded SNEDD exhibited better and statistically significant (**P < 0.001) DPPH scavenging activity than ascorbic acid at all tested concentrations except the last one, i.e. 20 µg/ml, at which, no significant difference was observed (P > 0.05). The better DPPH scavenging of luteolin may be attributed to faster hydrogen transfer from luteolin when compared with ascorbic acid. The phenolic compounds such as flavones and flavonoids possess multiple phenolic OH groups and inherent delocalized large pi-electron density; therefore, they exhibit better antioxidant potential than non-phenolic compounds [Citation87,Citation88].
Figure 10. Antioxidant activity (DPPH scavenging activity) presented as % mean scavenging ± SD (n = 3) of luteolin, luteolin-SNEDDS and ascorbic acid (standard antioxidant or positive control). “*” represents significant difference between the means of the treatments as P values were <0.05, “**” represents significant difference between the means of the treatments as P values were <0.01.
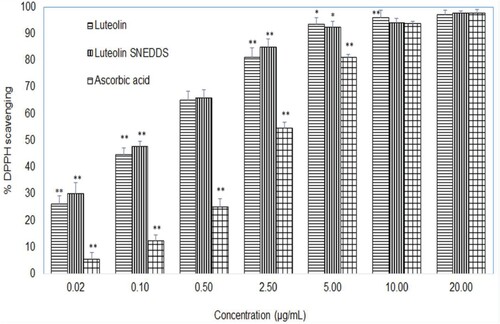
The flavonoids such as luteolin and quercetin that contain a 3′,4′-dihydroxy (o-dihydroxy group) in the B ring, 3-OH moiety of the C ring and C2–C3 double bond conjugated with a 4-keto group are reported to have a strong antioxidant potential [Citation89]. Several flavonoids such as quecetin, rutin, tannic acid gallic acid etc. have been reported to exhibit better antioxidant activity than standard (positive control) such as ascorbic acid, vitamin E and butylated hydroxyl toluene etc [Citation90–92].
In this study, all the tested samples exhibited concentration-dependent DPPH scavenging. The luteolin and luteolin-loaded SNEDD resulted faster reactions even at lower concentrations have shown plateau at a concentration of 5 µg/ml, while ascorbic acid exhibited slow reactions with a plateau at 10 µg/ml. This could be due to dimerization potential of flavonoids [Citation93]. The IC50 of luteolin and luteolin formulation were calculated as 0.18 and 0.23 µg/ml, respectively, while the IC50 of ascorbic acid was found as 2.56 µg/ml. There are few studies that have reported DPPH scavenging activity of luteolin better (IC50 ≈ 2 µg/ml) than ascorbic acid (IC50 ≈ 6 µg/ml) and tocopherol (IC50 ≈ 10 µg/ml) [Citation94]. Similarly, in another report, the DPPH scavenging activity of luteolin was found better (IC50 = 1.8 µM, that is equivalent to about 0.5 µg/ml) than vitamin E, α-tocopherol (IC50 = 5.2 µM) [Citation95]. Despite the slightly different IC50 of luteolin-loaded SNEDD from that of pure luteolin, the differences between DPPH scavenging activities at all tested concentration levels were not statistically significant. This may be due to the fact that in vitro DPPH assays involved comparison of luteolin and SNEDD formulation containing an equivalent amount of luteolin, in which both of the treatments were already dissolved completely in the test medium. Thus, in both cases, equal amount of drug was available at molecular level. Therefore, the clear-cut benefit of the formulation over pure luteolin could not be observed in this test. However, in the test such as saturation solubility test or ex vivo permeation test or in vivo absorption tests which involve solubility and permeability challenges, SNEDD formulation exhibited better results than pure luteolin.
3.14. In vivo anti-inflammatory activity
The anti-inflammatory activity of luteolin suspension (10 mg/kg body weight) was compared with luteolin-loaded optimized SNEDDS (F1–A1:1) at equivalent doses (10 mg/kg body weight) by using rat hind paw oedema model. Indomethacin at a dose of 10 mg/kg body weight was used as standard therapy for the sake of comparison as a positive control [Citation96,Citation97]. The dose of the luteolin was kept at 10 mg/kg body weight of rats as reported earlier with good anti-inflammatory activity of luteolin in rats [Citation98]. Further, only a single-dose anti-inflammatory study was conducted as the purpose of the study was to observe the benefits of the formulation such as better solubility and better absorption of luteolin from SNEDD when compared with luteolin alone. A dose-dependent anti-inflammatory effect of luteolin has already been reported in the mice and rats [Citation99,Citation100]. Anti-inflammatory activities of all treatments are presented as % inhibition of paw oedema (mean ± SE, n = 3) as shown in Figure . Luteolin caused 13.9 ± 1.89, 36.4 ± 5.58 and 11.8 ± 8.45% inhibition in the paw oedema at 1, 2 and 4 h post-dose while luteolin-loaded SNEDDS at equivalent doses caused 43.1 ± 10.58, 62.88 ± 7.04 and 52.1 ± 8.11%inhibition at the said time periods. The differences in the % inhibition caused by luteolin and SNEDDS were statistically significant at 1 and 2 h post-dose (*P<0.05) while highly significant at 4 h post-dose (**P<0.01). Based on the % inhibition data, it can be observed that the optimized SNEDDS formulation exhibited 2–4-fold higher anti-inflammatory activity when compared with luteolin which could be attributed to better absorption and bioavailability of luteolin from SNEDDS formulation. As for as % inhibition caused by indomethacin is concerned, it was also statistically highly significant (**P<0.01) when compared with leuteolin suspension at 4 h post-dose. It was noteworthy that anti-inflammatory activity of the luteolin-loaded SNEDDS at 1 and 2 h were better than standard therapy, indomethacin, which may be attributed to better absorption of luteolin loaded in SNEDD, besides the COX-2 selectivity of luteolin [Citation100,Citation101]. However, the differences in % inhibition caused by luteolin-loaded SNEEDS formulation and indomethacin were statistically insignificant at all observed time periods. The anti-inflammatory effect of indomethacin at a dose of 10 mg/kg in rats with carrageenan-induced paw oedema has been reported with ∼47% and 52% inhibition at 2 h which is very close to 50% inhibition as observed in this study [Citation96,Citation97]. The oral bioavailability of indomethacin loaded in SNEDD formulation is reported to be enhanced more than five times when compared with indomethacin alone administered to rats at a dose of 3 mg/kg body weight [Citation102]. The oral bioavailability of luteolin loaded in SNEDD may have improved that may have led to enhanced anti-inflammatory activity of the luteolin when compared with luteolin alone.
Figure 11. Anti-inflammatory activity (% mean inhibition in the oedema of rat paw, ± SE, n = 3) of luteolin suspension and luteolin-SNEDDS formulation after oral administration of 10 mg/kg. “*” represents significant difference between the means of the treatments as P values were <0.05, “**” represents significant difference between the means of the treatments as P values were <0.01.
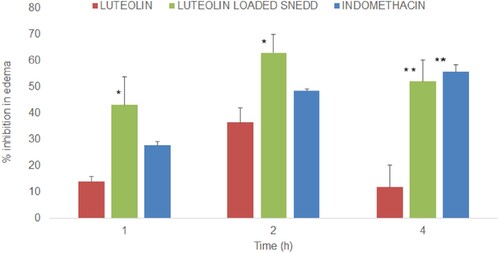
4. Conclusions
Luteolin is practically insoluble yellow pigment with several pharmacological activities. The purpose of the study was to improve solubility and permeability of luteolin in order to improve its bioavailability and efficacy. Based on the results obtained in this study, we conclude that castor oil, kolliphor and PEG 200 in a proportion of 10:45:45% v/v, respectively, produced an optimum preconcentrate of SNEDDS formulation for solubility and permeability improvement of loaded luteolin. The optimized formula exhibited improved solubility (∼83-fold), improved in vitro release (∼17-fold) and in vitro permeability (∼3-fold) of loaded luteolin when compared with luteolin suspension. Anti-inflammatory activity of luteolin-loaded SNEDDS formulation was enhanced 2–4-fold when compared with the activity of luteolin alone which could be due to improved absorption. Thus, we have observed significant improvement in solubility, in vitro release, ex vivo permeation and anti-inflammatory activity of luteolin from the formulation when compared with luteolin alone. The developed SNEDDS could be used as a potential carrier to improve the oral bioavailability of the luteolin and thereby improving the therapeutic potential and clinical benefits of luteolin.
Acknowledgments
We acknowledge that this publication was supported by the Deanship of Scientific Research at Prince Sattam bin Abdulaziz University.
Disclosure statement
The authors declare no conflict of interest.
Correction Statement
This article has been republished with minor changes. These changes do not impact the academic content of the article.
References
- Manzoor MF, Ahmad N, Manzoor A, et al. Food based phytochemical luteolin their derivatives, sources and medicinal benefits. Int J Agric Life Sci. 2017;3(2):195–207.
- Gutiérrez-Grijalva EP, Picos-Salas MA, Leyva-López N, et al. Flavonoids and phenolic acids from oregano: occurrence, biological activity and health benefits. Plants (Basel). 2017;7(1):1–23.
- Horváthová K, Novotný L, Tóthová D, et al. Determination of free radical scavenging activity of quercetin, rutin, luteolin and apigenin in H2O2-treated human ML cells K562. Neoplasma. 2004;51:395–399.
- Qiusheng Z, Yuntao Z, Rongliang Z, et al. Effects of verbascoside and luteolin on oxidative damage in brain of heroin treated mice. Pharmazie. 2005;60:539–543.
- Wölfle U, Esser PR, Simon-Haarhaus B, et al. UVB-induced DNA damage, generation of reactive oxygen species, and inflammation are effectively attenuated by the flavonoid luteolin in vitro and in vivo. Free Radic Biol Med. 2011;50:1081–1093.
- Wölfle U, Haarhaus B, Schempp CM. The photoprotective and antioxidative properties of luteolin are synergistically augmented by tocopherol and ubiquinone. Planta Med. 2013;79:963–965.
- Kimata M, Inagaki N, Nagai H. Effects of luteolin and other flavonoids on IgE-mediated allergic reactions. Planta Med. 2000;66:25–29.
- Ueda H, Yamazaki C, Yamazaki M. Luteolin as an anti-inflammatory and anti-allergic constituent of Perillafrutescens. Biol Pharm Bull. 2002;25:1197–1202.
- Hirano T, Higa S, Arimitsu J, et al. Flavonoids such as luteolin, fisetin and apigenin are inhibitors of interleukin-4 and interleukin-13 production by activated human basophils. Int Arch Allergy Immunol. 2004;134:135–140.
- Baolin L, Weiwei W, Ning T. Topical application of luteolin inhibits scratching behavior associated with allergic cutaneous reaction in mice. Planta Med. 2005;71:424–428.
- Kritas SK, Saggini A, Varvara G, et al. Luteolin inhibits mast cell-mediated allergic inflammation. J BiolReg Homeost Agents. 2013;27:955–959.
- Fei J, Liang B, Jiang C, et al. Luteolin inhibits IL-1β-induced inflammation in rat chondrocytes and attenuates osteoarthritis progression in a rat model. Biomed Pharmacother. 2019;109:1586–1592.
- Fan X, Du K, Li N, et al. Evaluation of anti-nociceptive and anti-inflammatory effect of luteolin in mice. J Environ Pathol Toxicol Oncol. 2018;37:351–364.
- Rakariyatham K, Wu X, Tang Z, et al. Synergism between luteolin and sulforaphane in anti-inflammation. Food Funct. 2018;9:5115–5123.
- Aziz N, Kim MY, Cho JY. Anti-inflammatory effects of luteolin: a review of in vitro, in vivo, and in silico studies. J Ethnopharmacol. 2018;225:342–358.
- Xiong J, Wang K, Yuan C, et al. Luteolin protects mice from severe acute pancreatitis by exerting HO-1-mediated anti-inflammatory and antioxidant effects. Int J Mol Med. 2017;39:113–125.
- Xia N, Chen G, Liu M, et al. Anti-inflammatory effects of luteolin on experimental autoimmune thyroiditis in mice. Exp Ther Med. 2016;12:4049–4054.
- Kanai K, Nagata S, Hatta T, et al. Therapeutic anti-inflammatory effects of luteolin on endotoxin-induced uveitis in Lewis rats. J Vet Med Sci. 2016;78:1381–1384.
- Yang PW, Lu ZY, Pan Q, et al. MicroRNA-6809-5p mediates luteolin-induced anticancer effects against hepatoma by targeting flotillin 1. Phytomedicine 2019;57:18–29.
- Pu Y, Zhang T, Wang J, et al. Luteolin exerts an anticancer effect on gastric cancer cells through multiple signaling pathways and regulating miRNAs. J Cancer. 2018;9:3669–3675.
- Palko-Labuz A, Sroda-Pomianek K, Uryga A, et al. Anticancer activity of baicalein and luteolin studied in colorectal adenocarcinoma LoVo cells and in drug-resistant LoVo/Dx cells. Biomed Pharmacother. 2017;88:232–241.
- Lee YJ, Lim T, Han MS, et al. Anticancer effect of luteolin is mediated by downregulation of TAM receptor tyrosine kinases, but not interleukin-8, in non-small cell lung cancer cells. Oncol Rep. 2017;37:1219–1226.
- Ma L, Peng H, Li K, et al. Luteolin exerts an anticancer effect on NCI-H460 human non-small cell lung cancer cells through the induction of Sirt1-mediated apoptosis. Mol Med Rep. 2015;12:4196–4202.
- Yan J, Wang Q, Zheng X, et al. Luteolin enhances TNF-related apoptosis-inducing ligand’s anticancer activity in a lung cancer xenograft mouse model. Biochem Biophys Res Commun. 2012;417:842–846.
- Zang Y, Igarashi K, Li Y. Anti-diabetic effects of luteolin and luteolin-7-O-glucoside on KK-A(y) mice. Biosci Biotechnol Biochem. 2016;80:1580–1586.
- Choi JS, Islam MN, Ali MY, et al. The effects of C-glycosylation of luteolin on its antioxidant, anti-Alzheimer’s disease, anti-diabetic, and anti-inflammatory activities. Arch Pharm Res. 2014;37:1354–1363.
- Kim JS, Kwon CS, Son KH. Inhibition of alpha-glucosidase and amylase by luteolin, a flavonoid. Biosci Biotechnol Biochem. 2000;64:2458–2461.
- Shakeel F, Haq N, Alshehri S, et al. Solubility, thermodynamic properties and solute-solvent molecular interactions of luteolin in various pure solvents. J Mol Liq. 2018;255:43–50.
- Product information Luteolin LKT LABS. Available from: https://www.lktlabs.com/product/luteolin/.
- Lipinski CALF. Poor aqueous solubility – an industry wide problem in drug discovery. Am Pharm Rev. 2002;5:82–88.
- Chen Z, Tu M, Sun S, et al. The exposure of luteolin is much lower than that of apigenin in oral administration of FlosChrysanthemi extract to rats. Drug Metab Pharmacokinet. 2012;27:162–168.
- Lin LC, Pai YF, Tsai TH. Isolation of luteolin and luteolin-7-O-glucoside from Dendranthemamorifolium Ramat Tzvel and their pharmacokinetics in rats. J Agric Food Chem. 2015;63:7700–7706.
- Liu B, Li W, Zhao J, et al. Physicochemical characterization of the supramolecular structure of luteolin/cyclodextrin inclusion complex. Food Chem. 2013;141:900–906.
- Jullian C, Cifuentes C, Alfaro M, et al. Spectroscopic characterization of the inclusion complexes of luteolin with native and derivatized beta-cyclodextrin. Bioorg Med Chem. 2010;18:5025–5031.
- Khan J, Saraf S, Saraf S. Preparation and evaluation of luteolin-phospholipid complex as an effective drug delivery tool against GalN/LPS induced liver damage. Pharm Dev Technol. 2016;21:475–486.
- Khan J, Alexander A, Saraf S, et al. Luteolin-phospholipid complex: preparation, characterization and biological evaluation. J Pharm Pharmacol. 2014;66:1451–1462.
- Xu K, Liu B, Ma Y, et al. Physicochemical properties and antioxidant activities of luteolin-phospholipid complex. Molecules. 2009;14:3486–3493.
- Wu G, Li J, Yue J, et al. Liposome encapsulated luteolin showed enhanced antitumor efficacy to colorectal carcinoma. Mol Med Rep. 2018;17:2456–2464.
- Zhao G, Zang SY, Jiang ZH, et al. Postischemic administration of liposome-encapsulated luteolin prevents against ischemia-reperfusion injury in a rat middle cerebral artery occlusion model. J Nutr Biochem. 2011;22:929–936.
- Li J, Cheng X, Chen Y, et al. Vitamin E TPGS modified liposomes enhance cellular uptake and targeted delivery of luteolin: an in vivo/in vitro evaluation. Int J Pharm. 2016;512:262–272.
- Huang M, Su E, Zheng F, et al. Encapsulation of flavonoids in liposomal delivery systems: the case of quercetin, kaempferol and luteolin. Food Funct. 2017;8:3198–3208.
- Zheng S, Cheng Y, Teng Y, et al. Application of luteolin nanomicelles anti-glioma effect with improvement in vitro and in vivo. Oncotarget. 2017;8:61146–61162.
- Qiu JF, Gao X, Wang BL, et al. Preparation and characterization of monomethoxypoly(ethylene glycol)-poly(ε-caprolactone) micelles for the solubilization and in vivo delivery of luteolin. Int J Nanomed. 2013;8:3061–3069.
- Yan H, Wei P, Song J, et al. Enhanced anticancer activity in vitro and in vivo of luteolin incorporated into long-circulating micelles based on DSPE-PEG2000 and TPGS. J Pharm Pharmacol. 2016;68:1290–1298.
- Qing W, Wang Y, Li H, et al. Preparation and characterization of copolymer micelles for the solubilization and in vitro release of luteolin and luteoloside. AAPS PharmSciTech. 2017;18:2095–2101.
- Majumdar D, Jung KH, Zhang H, et al. Luteolin nanoparticle in chemoprevention: in vitro and in vivo anticancer activity. Cancer Prev Res. 2014;7:65–73.
- Weerapol Y, Limmatvapirat S, Nunthanid J, et al. Self-nanoemulsifying drug delivery system of nifedipine: impact of hydrophilic-lipophilic balance and molecula structure of mixed surfactants. AAPS PharmSciTech. 2014;15:456–464.
- Yoo JH, Shanmugam S, Thapa P, et al. Novel self-nanoemulsifying drug delivery system for enhanced solubility and dissolution of lutein. Arch Pharm Res. 2010;33:417–426.
- El-Badry M, Haq N, Fetih G, et al. Solubility and dissolution enhancement of tadalafil using self-nanoemulsifying drug delivery system. J Oleo Sci. 2014;63:567–576.
- Shakeel F, Haq N, Alanazi FK, et al. Thermodynamic modeling for solubility prediction of indomethacin in self-nanoemulsifying drug delivery system (SNEDDS) and its individual components. Drug Dev Ind Pharm. 2014;40:1240–1245.
- Dash RN, Mohammed H, Humaira T, et al. Design, optimization and evaluation of glipizide solid self-nanoemulsifying drug delivery for enhanced solubility and dissolution. Saudi Pharm J. 2015;23:528–540.
- Gamal W, Fahmy RH, Mohamed MI. Development of novel amisulpride-loaded liquid self-nanoemulsifying drug delivery systems via dual tackling of its solubility and intestinal permeability. Drug Dev Ind Pharm. 2017;43:1530–1538.
- Kim DH, Maharjan P, Kim JY, et al. Enhanced solubility, in-vitro dissolution and lipase inhibition of a self-nanoemulsifying drug delivery system containing orlistat. J Nanosci Nanotechnol. 2019;19:634–639.
- Karasulu HY, Gundogdu E, Turk UO, et al. Enhancing solubility and bioavailability of rosuvastatin into self-nanoemulsifying drug delivery system. Curr Drug Deliv. 2018;15:1072–1082.
- Mohsin K, Alamri R, Ahmad A, et al. Development of self-nanoemulsifying drug delivery systems for the enhancement of solubility and oral bioavailability of fenofibrate, a poorly water-soluble drug. Int J Nanomed. 2016;11:2829–2838.
- Larsen AT, Ohlsson AG, Polentarutti B, et al. Oral bioavailability of cinnarizine in dogs: relation to SNEDDS droplet size, drug solubility and in vitro precipitation. Eur J Pharm Sci. 2013;48:339–350.
- Kim RM, Jang DJ, Kim YC, et al. Flurbiprofen-loaded solid SNEDDS preconcentrate for the enhanced solubility, in-vitro dissolution and bioavailability in rats. Pharmaceutics. 2018;10:E247.
- Kim DH, Kim JY, Kim RM, et al. Flurbiprofen-loaded solid SNEDDS preconcentrate for the enhanced solubility, in-vitro dissolution and bioavailability in rats. Orlistat-loaded solid SNEDDS for the enhanced solubility, dissolution, and in vivo performance. Int J Nanomed. 2018;13:7095–7106.
- Abouhussein DMN, Bahaa El Din Mahmoud D, Mohammad FE. Design of a liquid nano-sized drug delivery system with enhanced solubility of rivaroxaban for venous thromboembolism management in paediatric patients and emergency cases. J Liposome Res. 2019;1:1–14.
- Ansari MJ, Ahmed MM, Anwer MK, et al. Formulation and characterization of fluconazole loaded olive oil nano-emulsions. Indo Am J Pharm Sci. 2017;4:852–860.
- Louis D. Formulation and evaluation of nanocrystals of a lipid lowering agent. Iran J Pharm Res. 2016;15(1):71–82.
- Kazi M, Al-Swairi M, Ahmad A, et al. Evaluation of self-nanoemulsifying drug delivery system (SNEDDS) for poorly water-soluble talinolol: preparation, in vitro and in vivo assessment. Front Pharmacol. 2019;10:459.
- Saritha D, Bose P, Nagaraju R. Formulation and evaluation of self-emulsifying drug delivery system (SEDDS) of ibuprofen. Int J Pharm Sci Res. 2014;5:3511–3519.
- Nasr A, Gardouh A, Ghorab M. Novel solid self-nanoemulsifying drug delivery system (S-SNEDDS) for oral delivery of olmesartanmedoxomil: design, formulation, pharmacokinetic and bioavailability evaluation. Pharmaceutics. 2016;8:E20.
- Ansari MJ, Alshahrani SM. Nano-encapsulation and characterization of baricitinib using poly-lactic-glycolic acid co-polymer. Saudi Pharm J. 2019;27:491–501.
- Khan AW, Kotta S, Ansari SH, et al. Self-nanoemulsifying drug delivery system (SNEDDS) of the poorly water-soluble grapefruit flavonoid naringenin: design, characterization, in vitro and in vivo evaluation. Drug Deliv. 2015;22:552–561.
- AlShahrani S, Ansari MJ. Solubility evaluations of osimertinib mesylate in physiological buffers. Indo Am J Pharm Sci. 2018;5:2610–2615.
- Kumar A, Ahuja A, Ali J, et al. Curcumin-loaded lipid nanocarrier for improving bioavailability, stability and cytotoxicity against malignant glioma cells. Drug Deliv. 2016;23:214–229.
- Ruan LP, Chen S, Yu BY, et al. Prediction of human absorption of natural compounds by the non-everted rat intestinal sac model. Eur J Med Chem. 2006;41:605–610.
- Reddy SA, Sen SA, Chakraborty RA, et al. Effect of gokhru plant extract on intestinal absorption of aspirin using everted sac technique. Int J Pharm Boil Arch. 2011;2:549–553.
- Lassoued MA, Khemiss F, Sfar S. Comparative study of two in vitro methods for assessing drug absorption: Sartorius SM 16750 apparatus versus everted gut sac. J Pharm Pharm Sci. 2011;14(1):117–127.
- Marinova G, Batchvarov V. Evaluation of the methods for determination of the free radical scavenging activity by DPPH. Bulg J Agric Sci. 2011;17(1):11–24.
- Quest Graph™ IC50 Calculator (v.1). AAT Bioquest, Inc. Available from: https://www.aatbio.com/tools/ic50-calculator-v1.
- Astata 2016, by Navendu Vasavada. Available from: https://astatsa.com/OneWay_Anova_with_TukeyHSD/_get_data/.
- Ansari MJ, Kohli K, Ali J, et al. Enhanced anti-inflammatory activity of curcumin, a naturally occurring pigment in turmeric via cyclodextrin complexation. Orient Pharm Exp Med. 2007;7:121–127.
- Jelveghari M, Nokhodchi A. Development and chemical stability studies of alcohol-free phenobarbital solution for use in pediatrics: a technical note. AAPS Pharm Sci Tech. 2008;9:939–943.
- Censi R, Di Martino P. Polymorph impact on the bioavailability and stability of poorly soluble drugs. Molecules. 2015;20:18759–18776.
- Kalepu S, Nekkanti V. Insoluble drug delivery strategies: review of recent advances and business prospects. Acta Pharm Sin B. 2015;5:442–453.
- Kale SN, Deore SL. Emulsion micro emulsion and nano emulsion: a review. Syst Rev Pharm. 2017;8:39–47.
- Emerenciano DP, Baracho BB, Medeiros ML, et al. Physicochemical characterizations and antioxidant property of copaiba oil loaded into SNEDDS systems. J Braz Chem Soc. 2019;30(2):234–246.
- Beg S, Swain S, Singh HP, et al. Development, optimization, and characterization of solid self-nanoemulsifying drug delivery systems of valsartan using porous carriers. AAPS Pharm Sci Tech. 2012;13(4):1416–1427.
- Kassem AA, Mohsen AM, Ahmed RS, et al. Self-nanoemulsifying drug delivery system (SNEDDS) with enhanced solubilization of nystatin for treatment of oral candidiasis: design, optimization, in vitro and in vivo evaluation. J Mol Liq. 2016;218:219–232.
- Subramanian G, Meyyanathan S, Byran G. Stability-indicating reverse-phase high-performance liquid chromatography method for the simultaneous quantification of apigenin and luteolin from Achillea millefolium Linn. Asian J Pharm Clin Res. 2019;12(4):169–172.
- Wang M, Jin X, Ren X, et al. Comparative in vitro dissolution of two commercially available Er-Zhi-Wan herbal medicinal products. Indian J Pharm Sci. 2015;77(4):391–398.
- Ghai D, Sinha VR. Nanoemulsions as self-emulsified drug delivery carriers for enhanced permeability of the poorly water-soluble selective β1-adrenoreceptor blocker Talinolol. Nanomedicine. 2012;8(5):618–626.
- Malik P, Tapan KM. Structure-function elucidation of antioxidative and prooxidative activities of the polyphenolic compound curcumin. Chin J Biol. 2014;396708.
- Djeridane A, Yousfi M, Nadjemi B, et al. Screening of some Algerian medicinal plants for the phenolic compounds and their antioxidant activity. Eur Food Res Techn. 2007;224(6):801–809.
- Amic D, Davidovic-Amic D, Beslo D. Trinajstic N: structure-radical scavenging activity relationship of flavonoids. Croat Chem Acta. 2003;76:55–61.
- de Queiroz FR, Avaca LA. Electrochemical determination of the antioxidant capacity: the ceric reducing/antioxidant capacity (CRAC) assay. Electroanalysis. 2008;20(12):1323–1329.
- de Queiroz FR, Avaca LA. Electrochemical determination of the antioxidant capacity of Brazilian woods as alternative materials for the aging of cachaça. Braz J Food Technol. 2008;4:27–33.
- Olugbami JO, Gbadegesin MA, Odunola OA. In vitro free radical scavenging and antioxidant properties of ethanol extract of Terminalia glaucescens. Pharmacog Res. 2015;7(1):49–56.
- Furusawa M, Tsuchiya H, Nagayama M, et al. Anti-platelet and membrane-rigidifying flavonoids in brownish scale of onion. J Health Sci. 2003;49:475–480.
- De Martino L, Mencherini T, Mancini E, et al. In vitro phytotoxicity and antioxidant activity of selected flavonoids. Int J Mol Sci. 2012;13(5):5406–5419.
- Furusawa M, Tanaka T, Ito T, et al. Antioxidant activity of hydroflavonoids. J Health Sci. 2005;51:376–378.
- Baloch J, Sohail MF, Sarwar HS, et al. Self-nanoemulsifying drug delivery system (SNEDDS) for improved oral bioavailability of chlorpromazine: in vitro and in vivo evaluation. Medicina (B Aires). 2019;55(5):210–218.
- Morales G, Paredes A, Olivares A, et al. Acute oral toxicity and anti-inflammatory activity of hydroalcoholic extract from Lampayamedicinalis Phil in rats. Biol Res. 2014;47(1):6–11.
- Gambhire MN, Wankhede SS, Juvekar AR. Antiinflammatory activity of aqueous extract of Barleriacristata leaves. J Young Pharm. 2009;1(3):220–227.
- Kanai K, Nagata S, Hatta T, et al. Therapeutic anti-inflammatory effects of luteolin on endotoxin-induced uveitis in Lewis rats. J Vet Sci. 2016: 16–19.
- Fan X, Du K, Li N, et al. Evaluation of anti-nociceptive and anti-inflammatory effect of luteolin in mice. J Environ Pathol Toxicol Oncol. 2018;37(4):351–364.
- Lodhi S, Vadnere GP, Patil KD, et al. Protective effects of luteolin on injury induced inflammation through reduction of tissue uric acid and pro-inflammatory cytokines in rats. J Tradit Complement Med. 2020;10(1):60–69.
- Lamy S, Moldovan PL, Saad AB, et al. Biphasic effects of luteolin on interleukin-1β-induced cyclooxygenase-2 expression in glioblastoma cells. BBA-Mol Cell Res. 2015;1853(1):126–135.
- Taha EI, Al-Suwayeh SA, El-Badry M. Bioavailability study of indomethacin self-nanoemulsifying oral formulation in rats. Aust J Basic Appl Sci. 2009;3(3):2944–2948.