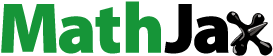
Abstract
Essential oil of Elettaria cardamomum (Green cardamom) is effective in the treatment of various disease conditions. Globally, Guatemala is the leading producer of green cardamom fruits. Green-cardamom fruits from Guatemala were purchased from a Saudi Arabian market. The Guatemala cardamom essential oil (GCEO) was extracted using hydro-distillation method, and analyzed using gaschromatography/ mass-spectrometry (GC-MS). The antioxidant and anti-inflammatory activities were studied using 2,2-diphenyl-1-picrylhydrazyl-hydrate, ferric-chloride, bovine serum-albumin and proteinase inhibitory assays. GC-MS results confirmed the presence of α- terpinyl acetate and 1,8-cineole as major components. The antioxidant and anti-inflammatory activities of GCEO were found to be significant compared with those of standard ascorbic acid and ibuprofen, respectively. The molecular-docking study revealed the considerable binding potential of α-terpinyl acetate and 1,8-cineole with human-peroxiredoxin-5, tyrosine-kinases and Human-5-LOX receptors. The in-silico activity prediction analysis confirmed the antioxidant and anti-inflammatory potential, whereas the pharmacokinetics confirmed the safety of most of the GCEO compounds.
1. Introduction
Elettaria cardamomum Maton (Zingiberaceae), termed as the “queen of spices”, light green or green cardamom, and small or lesser cardamom, is well known for its characteristic aroma and taste, and it is traditionally used in medicine and food [Citation1]. Currently, Guatemala is the foremost commercial manufacturer of lesser cardamom, which is often used in the preparation of “strong cardamom coffee” called “Gahwa” in Middle East Asia. Lesser cardamom is commonly used in Ayurveda and Unani medicines for the treatment of intestinal disorders [Citation2]. Studies have suggested that cardamom is effective against hepatotoxicity and gastric or ulcerogenic ailments [Citation3]. Elguindy et al. reported that lesser cardamom could be used against hepatocellular cancer. Gilani et al. suggested that lesser cardamom is beneficial against sedation and epilepsy [Citation3]. It has been scientifically evaluated for its antioxidant, anti-inflammatory, antimicrobial [Citation4], anticancer [Citation5], antidiarrheal [Citation6] effects. Studies have reported its effect on multiple myeloma [Citation7]; blood pressure, vasodilation and collective heart complaints as well as its anti-cholesterol and anticoagulation properties [Citation8]. Gilani et al. reported that lesser cardamom can lower high blood pressure [Citation3]. Goyal et al. reported its cardioprotective effects [Citation9]. Bhaswant et al. reported that it is effective against hepatotoxicity [Citation10]. Nitasha Bhat et al. (2015) reported its effects on obesity, hypothyroidism, diabetes, hepatomegaly, dyslipidaemia and rapid hyperglycaemia [Citation11]. They also stated that a US-patented antidiabetic formulation contains several plant extracts, including that of cardamom. Among respiratory disorders, it can be used to treat cold and cough, asthma, bronchitis, tuberculosis and sore throat. Nitasha Bhat et al. also reported that lesser cardamom could be used to treat as anti-asthmatic [Citation11]. Khan et al. demonstrated and reported the antihistaminic effects of cardamom extracts on isolated rabbit trachea tissues [Citation12]. Kumar et al. (2016) reported that lesser cardamom helps boost and regain memory and demonstrated its antidepressant properties [Citation13]. E. cardamomum capsules possess a wide array of bioactive components, and the central component is α-terpinyl acetate and 1,8-cineole [Citation6,Citation14]. The composition of essential oil depends on the geographical condition chemotype, growing condition, harvesting time of a plant [Citation15]. International organization for standardization (ISO) developed a code for the cardamom oil (ISO 4733:2004) and data show the variation in the major composition of essential oil in different seeds batches [Citation16] and this may be one of the major regions that reflect precise and accurate doses of cardamom oil. The cardamom volatile oil contains antioxidant components, which primarily contribute to its distinctive odour and role in nutraceutical and medicinal value [Citation17]. Some doses of cardamom essential oil, such as doTERRA [Citation18] and Edens Gardens cardamom essential oil 5 mL [Citation19], are available on the market and are used in traditional medicine to treat inflammatory diseases. The doses 175–280 and 233 L/kg were similarly found to be beneficial for anti-inflammatory and analgesic action in a prior study [Citation20]. Heimesaat et al. (2021) observed that a daily intake of 258 mg per kg bodyweight of cardamom essential oil inhibited mice pro-inflammatory mediator [Citation21].
The oxygenated monoterpenes found in the essential oil of green cardamom, such as α-terpinyl acetate, 1,8-cineole and α-terpineol, among others, exhibit antioxidant, anti-inflammatory, gastroprotective, antiviral and antibacterial properties [Citation22,Citation23]. The smell of the extracted fruit oils is due to the presence of the metabolite α-terpinyl acetate, as well as other substances [Citation24]. Lesser cardamom contains up to 50% α-terpinyl acetate and 1,8-cineole as phytoconstituents [Citation25,Citation26]. E. cardamomum essential oil has been shown to have antioxidant and anti-inflammatory properties in a number of studies [Citation22,Citation27].
In the oxidative stress, reactive oxygen species (ROS) accumulates in the cells, excessive accumulation of ROS trigger sever cellular enzymes, and may affects the growth, metabolism, differentiation and motility of the normal cells [Citation28]. Human peroxiredoxin 5 (PRDX5) located mainly in the mitochondria, peroxisomes and cytosol while protein tyrosine kinases (PTKs) located in the cellular membrane, both the enzymes along with several other functions involved in the antioxidant protection [Citation29,Citation30]. Human 5-LOX a lipoxygenase-enzyme, that is involved in the leukotrienes biosynthesis and participates in the inflammatory responses, its inhibition may be a potential target for evaluation of a new anti-inflammatory agents [Citation31]. Molecular docking, in silico absorption, distribution, metabolism, elimination (ADME) and prediction of activity spectra for substances (PASS) testing by computational studies are used to predict drug targets, drug likening and pharmacological potential of the active compounds [Citation32].
Considering the enormity of research reports on the pharmacological effects of E. cardamomum essential oil, we aimed to explore the constituents and in vitro antioxidant and anti-inflammatory spectrum of Guatemala cardamom essential oil (GCEO). Further, in silico drug-like prediction of several compounds using ADME, PASS and molecular docking studies of the major chemical constituents using three targets, such as human peroxiredoxin 5 (IHD2), protein tyrosine kinases (3CC6) and human 5-LOX (3V99), are also conducted to account and support for the results of in vitro experimental outcomes.
2. Materials and methods
2.1. Chemicals
All the chemical, namely 2,2-diphenyl-picrylhydrazyl hydrate (DPPH), ferric chloride, potassium ferricyanide (C6N6FeK3), ascorbic acid, bovine serum albumin (BSA), Tris-HCl buffer, casein, perchloric acid (70%), trypsin and trichloroacetic acid (TCA), were purchased from Sigma-Aldrich (Germany).
2.2. Sample used and extraction of essential oils
Fruit sample of Guatemala E. cardamomum (lesser cardamom), labelled as a brand name “AlMEHBAJ”, was obtained from the local market of Al-Kharj (Kingdom of Saudi Arabia, KSA) in January 2020. The sample was deposited in the Department of Pharmacognosy, College of Pharmacy, Prince Sattam Bin Abdulaziz University (PSAU, Al-Kharj, KSA). The fruit was dried and powdered. The essential oil (lower density than water) was extracted using a Clevenger type apparatus (capacity 10 mL) connected onside with 2 L round bottom flask overheating mantle and other side condenser connected with the chiller (Buchi B-741). 100 g of fruit powder was extracted at 60°C for 3 h [Citation6]. The essential oil obtained was collected and dried using anhydrous Na2CO3; after complete drying, it was weighed and kept in an amber-coloured air-tight vial and stored at 4°C for performing in vitro antioxidant and anti-inflammatory activity assays. The experiment was performed in triplicate, and data were expressed as percentage ± standard deviation.
2.3. Characterization of the essential oil composition using gas chromatography-mass spectrometry
GCEO was dissolved in acetone (10 µL/mL) and analysed using gas chromatography (GC) equipped with mass spectrometer detector (MSD) Agilent HP 6890/5977 (Agilent technologies Inc., CA, Santa Clara, USA); the system was operating at 70 eV. 3 µL solution was injected in a split-mode (ratio, 100:1) in a HP-5MS column (Agilent Technologies Inc.) with the following dimensions: length, 30 m; diameter, 0.25 mm; and film-thickness of stationary phase, 0.25 µm. The column oven temperatures, sample injection, separation and identification of compounds were adopted from the previous works [Citation33,Citation34]. In brief, temperatures for the injector and detector were fixed at 270°C and 300°C, respectively. The oven temperature was programmed as follows: 40°C (storage time of 1 min) with a rate of 10°C/min to 110°C (storage time of 1 min), and continued to 300°C (hold time of 5 min), and finally reached to 325°C at the same rate; the total run time was 10 min. Helium constant flow was set at 3 mL/min. The solvent delay was 3 min, and the scan mass/charge range was between 50 and 550 m/z. Agilent MassHunter (NIST14.L) analysis was used for the data analysis. The documentation of compounds was based on the assessment of retention time and spectra of mass with the reference spectra (National Institute of Standards and Technology; NIST, 14.L). Essential oil composition of GCEO was also identified based on the retention indices (RIs) relative to n-alkanes homologous series (C8-C30) and by comparing their mass spectra with those recorded in NIST 05 and Wiley 275 inherent mass spectral libraries and with the literature data [Citation35].
2.4. In vitro antioxidant activities
2.4.1. DPPH free radical scavenging activity
The free radical scavenging (FRS) ability of GCEO was analysed using the DPPH assay, and the method followed by Alam and Majumdar was used, with some modifications [Citation36]. Briefly, 10 mL DPPH-cation solution (0.1 mM) was prepared in methanol. Different dilutions (62.5–1000 μg/mL) of essential oil and standard ascorbic acid were separately prepared in methanol. Then, 2 mL of diluted sample and 2 mL of DPPH were vortexed and incubated at 26°C for 30 min. After incubation, the maximum absorbance (Ab) was recorded at 517 nm against a blank (2 mL DPPH + 2 mL methanol) by using an ultraviolet-visible (Genesys™ 10S UV-Vis, Thermo Scientific, USA) spectrophotometer. The experiment was performed in triplicate. The percentage of FRS was determined using the following equation:
2.4.2. Fecl2 reducing assay
The FeCl2 reducing assay was performed using a previously reported method [Citation37], with minor modifications. In brief, stock solutions (1000 µg/mL) of oils and ascorbic acid were used for preparing different dilutions (200–25 μg/mL). Approximately 1 mL of different concentrations was separately added to 2.5 mL, each of 0.2 mol/L (pH 6.6) phosphate buffer, and 1% C6N6FeK3 solution. These solutions were incubated for 30 min at 50°C. To 2.5 mL of above mixtures, 10% TCA was added. Later, these mixtures were centrifuged for 10 min at 3000 g. Approximately 2.5 mL of supernatant of the centrifuged solution was mixed with double distilled water (2.5 mL) and then, the mixture was shaken with 0.5 mL of freshly prepared 0.1% FeCl3 solution and kept for 10 min. The value of absorbance was noted at 700 nm against blank (n = 3).
2.5. In vitro anti-inflammatory activities
2.5.1. Albumin denaturation assay
In vitro anti-inflammatory potential of GCEO was estimated using the BSA denaturation method, with slight modifications, as described by Alam and Singh [Citation38]. The GCEO and ibuprofen were dissolved in methanol to prepare 1 mg/mL solution. Further, five dilutions of GCEO and ibuprofen ranging from 6.25 to 100 μg/mL were prepared in phosphate buffered saline (PBS; pH = 6.8). Approximately 100 µL of essential oil or standard, 1000 µL of BSA (1%) and 1400 µL of BSA were thoroughly mixed. This mixture was incubated at 37°C for 15 min; then, the reaction mixture was heated at 72°C for 5 min. The reaction mixture was cooled, and the absorbance was determined at 660 nm against a blank (1000 µL of 1% BSA solution and 1500 µL PBS) by using a UV-Vis spectrophotometer (Genesys™ 10S UV-Vis, Thermo Scientific, USA). The percentage of inhibition (protein denaturation) of three repeated experiments was determined using the following equation:
2.5.2. Proteinase inhibitory assay
The proteinase inhibitory activity of GCEO was evaluated using trypsin method with slight modifications [Citation39]. Six dilutions of standard and test samples ranging from 6.25 to 200 μg/mL were prepared in methanol. Approximately 1 mL of test sample, 0.06 mg trypsin and 1 mL Tris-HCl buffer (20 mM) were thoroughly mixed. This mixture was incubated at 37°C for 15 min, and then, 1 mL of casein (0.8% w/v) was added to the mixture. The reaction mixture was further incubated at 37°C for 20 min. Subsequently, 2 mL of per-chloric acid (70%) was added to the mixture. The reaction mixture was centrifuged for 5 min at 3000 rpm. The supernatant was separated, and the absorbance was measured at 210 nm against a blank (reaction mixture without sample and standard solution). The percentage of inhibition (protein denaturation) of three repeated experiments was determined using the following equation:
2.6. In silico studies
2.6.1. Molecular docking studies
In the present study, the first and second (α-terpinyl acetate and 1,8-cineole respectively) major compounds were selected for the molecular docking. AutoDock-4.2.6 tool was applied for creating the file of PDBQT from traditional PDB files [Citation40]. Three-dimensional (3D) structures of 1HD2: peroxiredoxin 5 proteins [Citation41], 3CC6: tyrosine kinase 2 beta [Citation42] and 3V99: lipoxygenase [Citation43] were downloaded from the PDB database. For molecular docking, the structures of α-terpinyl acetate and 1,8-cineole were drawn and then converted into the PDB format by using ACD/ChemSketch (Version 21) and an open Babel software [Citation44], respectively. The ligands were first optimized and then docked into targeted proteins by using the “Ligand fit” model (AutoDock 4.2.6). Then, the 3D structures of the 1HD2-, 3CC6- and 3V99-targeted proteins were docked with the ligands by using AutoDock software. The BIOVIA Discovery Studio Visualizer 21 software [Citation45] was used for the analysis of docking results. The binding energy, residues and length between the target protein and target ligand were calculated.
2.6.2. PASS and ADME prediction studies
For activity and drug-likening prediction, seven significant percentage compounds α-terpinyl acetate, 1,8-cineole, α-terpineol, terpinen-4-ol, β-pinene, linalyl acetate and β-selinene were selected. The structure of the selected compounds was drawn using ChemDraw (ChemDraw® JS) (Figure ) and converted into the SMILES format and then the SMILES format was simulated using PASS online web tool [Citation46]. The prediction of activity spectra for biologically active substances (PASS), which predicts the probable activity (Pa) and probable inactivity (Pi), was used to predict the pharmacological activities of major compounds present in GCEO by using PASS online web tool. ADME properties of the major compounds (α-terpinyl acetate, 1,8-cineole, α-terpineol, terpinen-4-ol, β-pinene, linalyl acetate and β-selinene) were also predicted using SwissADME tool software [Citation47]. The ADME study predicts the lipophilicity, physicochemical properties, drug-likeness and toxicity of compounds.
2.7. Statistical analysis
Data were analysed using GraphPad InStat software and is presented as the mean ± standard deviation. The IC50 value (the essential oil concentration providing 50% inhibition) was calculated from the graph by plotting the percentage inhibition versus concentration of essential oil. Regression equations, correlation coefficient (r), and level of significance (p ≤ 0.05) were used for analysing the antioxidant and anti-inflammatory results.
3. Results and discussion
3.1. Characterization of the essential oil composition by GC–MS
The percentage yield of the extracted essential oils of Guatemala cardamom capsules using the hydrodistillation method was 4.30% ± 0.57%. Figure shows the GC–MS chromatogram of GCEO. Thirty four components were identified using the GC–MS instrumentation (Table ). The major metabolites identified in the essential oil was α-terpinyl acetate (27.14%), 1,8-cineole (15.40%), α-terpineol (7.44%), terpinen-4-ol (5.05%), linalyl acetate (4.49%), β-pinene (3.55%) and β-selinene (3.23%).
Table 1. Chemical composition of the GCEO.
The percentage yield of Guatemala cardamom was found to be similar to that reported in a previous study [Citation48]. The aroma of GCEO is primarily due to the combined effects of α-terpinyl acetate and 1,8-cineole [Citation49]. Several studies have reported that α-terpinyl acetate is a major component of the cardamom essential oil [Citation6,Citation50]. The concentration of α-terpinyl acetate metabolite is higher than that of other metabolites in high-quality cardamom essential oil; therefore, it may be used as an indicator for the identification of superior-quality cardamom fruits. In volatile oil of Guatemala cardamom, the total concentration of monoterpenes was found to be 87.05%, of which the concentration of oxygenated monoterpenes and monoterpene hydrocarbons was found to be approximately 69.99% and 17.06%, respectively. Kumar et al. (2005) analysed the essential oil of Indian E. cardamomum fruits and reported the concentration of oxygenated monoterpenes and monoterpenes hydrocarbons to be approximately 87% and 8.24%, respectively. Their finding is quantitatively and qualitatively different from the findings of the present study [Citation51]. The total sesquiterpenes were found to be 10% in Guatemala cardamom. Abdullah et al. (2017) analysed the essential oil of E. cardamomum fruits collected from the local market in Pakistan and reported a higher concentration of monoterpene than that of sesquiterpenes in the essential oil [Citation22]. Snoussi et al. (2015) studied the essential oil of E. cardamomum obtained from local Tunisian market and demonstrated different concentrations of monoterpenes and sesquiterpenes [Citation49]. Seven major compounds (<3.5%) comprising five oxygenated monoterpenes (α-terpinyl acetate, 1,8-cineole, α-terpineol, terpinen-4-ol and linalyl acetate), one monoterpene hydrocarbons (β-pinene), and one sesquiterpene (β-selinene) were detected in the GCEO. These compounds contribute to approximately 66.3% of total oil composition (97.26%). Similar to an earlier study [Citation24], a diterpene coronarin E was identified in the present study. More recently, Ivanović et al. (2021) compare the essential oils of some Zingiberaceae family including the essential oil of E. cardamomum obtained from local market in Slovenia (Central Europe) and reported that α-Terpinyl acetate and 1,8-Cineole are the major components similar to the present results [Citation52].
3.2. Antioxidant activities
The DPPH scavenging method was used to assess the antioxidant properties of GCEO. At high concentrations, GCEO has been discovered to have a substantial antioxidant action. At a concentration of 1000 µg/mL, the DPPH-induced free radical scavenging activity of GCEO was determined to be around 67.04 ± 0.04% (Figure (A)). Standard ascorbic acid’s antioxidant potential was determined to be 99.1 ± 0.07 percent higher than that of GCEO. The IC50 was 588.6 µg/mL, which was larger than the usual ascorbic acid value of 24.02 µg/mL. GCEO’s reducing ability was found to be lower than that of ordinary ascorbic acid (Figure (B)). In the FeCl2 reduction model, the absorbance value of GCEO (0.448 ± 0.01) of GCEO in the FeCl2 reducing model was found to be lower than that of ascorbic acid (1.254 ± 0.02). A dose-dependent, noteworthy (p < 0.001) increment in the antioxidant potential, along with an increase in concentration effects, was observed.
Figure 2. Antioxidant activity of GCEO using (A) DPPH free radical scavenging assay, (B) Ferric chloride reducing assay. The values were expressed as mean ± SD calculation (n = 3).
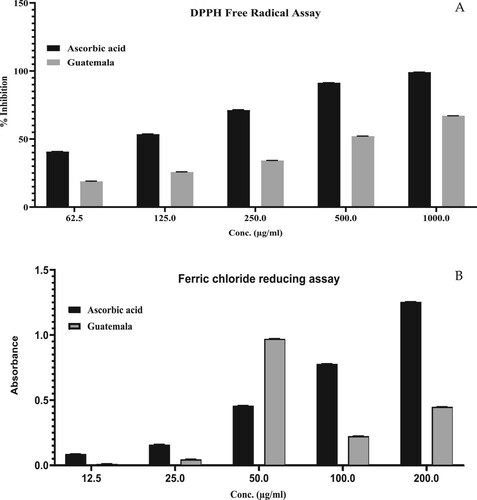
The IC50 value of GCEO indicated a weaker inhibition of the DPPH free radical compared to the standard compounds. The antioxidant effects of essential oils are mainly due to the presence of α-terpinyl acetate, 1,8-cineole, linalyl acetate, β-pinene, α-terpineol and terpinen-4-ol [Citation53–55]. Furthermore, our studies align with a previous study [Citation56]. The FeCl2 assay is dependent on the formation of potassium ferrous cyanide after the reaction between potassium ferric cyanide and tested compounds. Han et al. (2017) reported that the reducing property increases with an increase in the concentration of essential oils [Citation57]. Several studies have revealed that E. cardamomum has a good antioxidant potential against oxidative stress [Citation48,Citation58].
3.3. Anti-inflammatory activity
The BSA- and trypsin-induced inflammation assays were assessed to determine the anti-inflammatory activity, and the outcomes are shown in Figure (A and B), respectively. The inhibition of albumin-induced inflammation by GCEO extended from 16.98% ± 0.18% to 54.16% ± 0.12% at a concentration range of 6.25–100 µg/mL. The inhibition of trypsin-induced inflammation increased from 14.64% ± 0.94% to 54.82% ± 0.23% with an increase in the concentration from 6.25 to 200 µg/mL. The value of anti-inflammatory activity of GCEO was found to be comparable (p < 0.001) to the standard ibuprofen. The IC50 values of GCEO were found to be 83.86 and 159.1 µg/mL in the egg albumin and trypsin assay, respectively, which were higher than those of the standard ibuprofen (25.95 and 32.23 µg/mL in the egg albumin and trypsin assay, respectively). In both methods, a dose-dependent increment in the anti-inflammatory potential, along with an increase in concentration effects, was observed.
Figure 3. Anti-inflammatory activity of GCEO using (A) BSA inhibitory assay (B) Proteinase inhibitory assay. The values were expressed as mean ± SD calculation (n = 3).
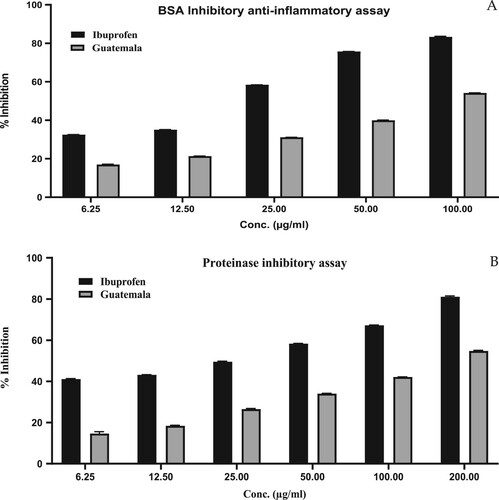
The anti-inflammatory potential of GCEO at similar concentrations of standard (Ibuprofen) exhibited significant (p < 0.001) inhibition of albumin- and trypsin-induced inflammation, which indicated the anti-inflammatory properties of GCEO. Several pharmacological studies have been conducted to determine the activity of cardamom essential oil. The present study indicates that GCEO exhibits moderate anti-inflammatory potential. The anti-inflammatory activity of GCEO may be attributed to the presence of a high percentage of α-terpinyl acetate, 1,8-cineole, linalyl acetate, α-terpineol, β-pinene, terpinen-4-ol, β-selinene and several other components [Citation25,Citation54,Citation59].
3.4. Molecular docking studies
The major metabolites, α-terpinyl acetate and 1,8-cineole, were selected for molecular docking studies. Molecular docking was performed against antioxidant [peroxiredoxin-5 proteins (PDB: 1HD2) and tyrosine kinase 2 beta (PDB: 3CC6)] and anti-inflammatory [lipoxygenase proteins (PDB: 3V99)] receptors to identify the critical ligand–protein interactions (Figure ; where 1 and 2 represent α-terpinyl acetate and 1,8-cineole, respectively). Table presents the results of binding energy, docking score, residues and length of hydrogen bonding.
Figure 4. Interaction of 1HD2, 3CC6 and 3V99 protein with α-terpinyl acetate (1) and 1,8-cineole (2) inhibitors.
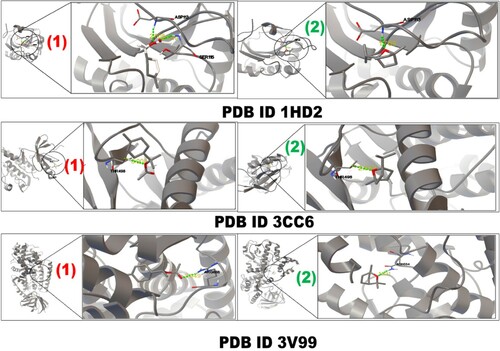
Table 2. The docking score, residues and hydrogen bond length for α-terpinyl acetate and 1,8-cineole with target proteins.
The major metabolite α-terpinyl acetate demonstrated a highest binding-affinity against the lipoxygenase (LO) receptor (PDB ID: 3V99) with the docking score of −6.68 kcal/mol and the lowest affinity against the peroxiredoxin-5 receptor (PDB ID: 1HD2) with a score of −4.96 kcal/mol. The second major compound 1,8-cineole demonstrated similar binding affinities of −5.20 kcal/mol, −5.17 kcal/mol and −5.11 kcal/mol against the 3V99, 3CC6 and 1HD2 receptors, respectively.
Docking (molecular) examinations were employed broadly for the assessment of ligand–target connotation to enhance understanding of the pharmacological properties of natural compounds. It delivers mechanisms of probable action and mode of binding within the protein pockets [Citation60]. Two major components of GCEO (α-terpinyl acetate and 1,8-cineole) were selected for docking studies to support the present in vitro antioxidant and anti-inflammatory activities. The compounds, α-terpinyl acetate and 1,8-cineole, were docked with two antioxidant receptors, peroxiredoxin-5 proteins (PDB: 1HD2) and tyrosine kinase 2 beta (3CC6), and one anti-inflammatory receptor, lipoxygenase LO (PDB: 3V99). The docking scores ranged from −4.96 to −6.68 kcal/mol. α-terpinyl acetate interacted with a few amino acid residues within 3V99, 3CC6 and 1HD2, with docking scores of −6.68, −5.68 and −4.96 kcal/mol, respectively. Similarly, 1,8-cineole interacted with several amino acid residues within 3V99, 3CC6 and 1HD2, with docking scores of −5.20, −5.17 and −5.11 kcal/mol, respectively. These results indicated that both these compounds are responsible for the antioxidant and anti-inflammatory activities of GCEO. Interactions of α-terpinyl acetate with 3V99, 3CC6 and 1HD2 proteins indicated ARG666, THR498, ASP113 and SER115, respectively, whereas the interaction of 1,8-cineole with the 3V99, 3CC6 and 1HD2 proteins indicated ASN554, THR498 and ASP113, respectively. Lipoxygenase LO (PDB: 3V99) is a potential target to predict the anti-inflammatory activity of a compound [Citation61]. Human peroxiredoxin-5 (PDB: 1HD2) and tyrosine kinase 2 beta (3CC6) are a potential target to predict the antioxidant potential of drugs [Citation62].
3.5. PASS and ADME prediction studies
Seven major compounds (Figure ) of GCEO were selected for the antioxidant (Pa) and anti-inflammatory (Pi) prediction studies using the PASS online tool. Table presents the predicted PASS results. The pharmacokinetic features of all major GCEO compounds were also determined using an online tool (SwissADME). The study outcome revealed that all selected compounds fulfil the Lipinski’s rule and strongly support oral bioavailability properties (Table ). The bioavailability radar graph, which represents physicochemical, pharmacokinetics and drug-likeness properties, is shown in Figure (A), and the gastrointestinal (GIT) absorption and blood–brain penetration properties of selected compounds are illustrated in Figure (B).
Table 3. PASS prediction bioactivities of major constituents of GCEO.
Table 4. In silico ADMET profile of GCEO compounds.
To assess the possible antioxidant and anti-inflammatory profile of the major compounds of GCEO, was used PASS. For all seven compounds, the possible-activity (Pa) range of 0.132–0.422 and 0.337–0.751 for antioxidant and anti-inflammatory actions, respectively, support our laboratory investigations suggesting good antioxidant and moderate anti-inflammatory effects. The in vitro GCEO pharmacological effects observed may be due to the combined effect of many compounds. The antioxidant potential among the identified compounds terpinen-4-ol, β-pinene and linalyl acetate – exhibited acceptable Pa values (0.422, 0.399 and 0.384, respectively). However, other compounds exhibited lower antioxidant effects as per the PASS prediction. The overall PASS analysis supported the present in vitro antioxidant potential of GCEO. The Pa value for the anti-inflammatory activities of the identified compounds, linalyl acetate (0.751), β-selinene (0.705), α-terpinyl acetate (0.693), α-terpineol (0.651), β-pinene (0.611), terpinen-4-ol (0.609) and 1,8-cineole (0.327). Overall, the PASS prediction supported the present moderate in vitro anti-inflammatory potential of GCEO compounds. Results of the online prediction software ADME obtained from in silico ADME Swiss software studies clearly indicated that all the selected volatile compounds have drug-like properties with no violation of any of the drug-likeness rules [Citation63,Citation64]. High GI absorption was predicted for most of the selected compounds; most compounds can be easily absorbed by the GI tract. According to the present Swiss-prediction, the major compounds found in GCEO cannot be affected by the P-glycoprotein of the central nervous system. Drug-like properties and GI absorption of the major compounds of GCEO were also predicted using the bioavailability radar graph and BOILED-Egg prediction. The pink area of radar graph represents drug-likeness, and the compounds present in the yellow zone (except β-selinene) can permeate through the blood–brain barrier.
Overall, molecular docking study of major compounds (α-terpinyl acetate and 1,8-cineole) and PASS prediction study indicated the strong antioxidant and anti-inflammatory potential of cardamom oil as well as ADME predicts the drug likening properties of majority of the compounds. In the docking, α-terpinyl acetate and 1,8-cineole have shown good binding with the human peroxiredoxin 5 and protein tyrosine kinases receptors. These receptors play an important role in controlling the abnormal growth, metabolism, differentiations and motility of the normal cells through antioxidant mechanism [Citation29,Citation30]. Further, these molecules are studied in PASS prediction to prove the better antioxidant activity. Our in vitro and prediction antioxidant results were strongly supported by several recent studies reporting potent antioxidant effects of cardamom essential oil [Citation25,Citation52]. It is now emphasized that these molecules could be a better antioxidant by PASS predictions.
In addition, the α-terpinyl acetate and 1,8-cineole have been studied for anti-inflammatory activity through inhibition of 5-lipoxygenase enzymes [Citation65,Citation66]. The in vitro anti-inflammatory activity findings are well correlated with the molecular docking and anti-inflammatory activities by PASS. The present findings (in vitro and in silico) are also supported by al-Zuhair et al. [Citation20] and Heimesaat et al. [Citation21]. Based on our findings and correlations with other investigators, we have strongly proven that these molecules might be a better antioxidant and anti-inflammatory properties and a good source of future drugs.
4. Conclusion
Despite the extensive use of essential oils in perfumes, food and pharmaceutical products, modern science has barely explored this treasure scientifically. In the present study, essential oils from cardamom fruits obtained from Guatemala (a large producer) were assessed. GCEO demonstrated strong antioxidant and moderate anti-inflammatory activities. Molecular docking studies of two major compounds (α-terpinyl acetate and 1,8-cineole) of GCEO with the proteins 3V99, 3CC6 and 1HD2 theoretically exhibited activities which are in agreement with the in vitro antioxidant and anti-inflammatory results, as indicated by their significant protein–ligand interaction energy. The antioxidant receptors, human peroxiredoxin 5 and protein tyrosine kinases, as well as the anti-inflammatory receptor 5-lipoxygenase, may be triggered by the essential oil effects of green cardamom. The essential oil of cardamom may be a natural and alternative treatment for oxidative stress and inflammatory reactions. The major compounds present in GCEO exhibited promising in silico docking results, where the PASS prediction supports the in vitro antioxidant and anti-inflammatory outcomes. SwissADME studies clearly indicated that the compounds present in GCEO have drug-like properties. The presence of volatile chemicals in the essential oil of cardamom fruits, such as α-terpinyl acetate, 1,8-cineole, linalyl acetate, α-terpineol, β-pinene, terpinen-4-ol and β-selinene, may be responsible for the antioxidant and anti-inflammatory properties. In the future, animal models will be necessary to validate current in vitro or in silico results.
Author contributions
AA and PA contributed to the design and supervision of this study, AA and MTJ participated in the in vitro studies. AA performed the statistical analysis. The manuscript was written by AA, The editing of the manuscript was done by AA, PA and MTJ. Project administration and funding acquisition were controlled by AA and MTJ. All authors have read and agreed to the published version of the manuscript.
Acknowledgements
The authors are very grateful to Dr Afroz Bakht (PSAU-Al-Kharj, Saudi Arabia) for his help on the GC–MS analyses. The authors equally thank Dr. Piyush Kumar (College of Pharmacy, Integral University, Lucknow, UP, India) for helping in molecular docking study.
Conflict of interest
Authors have no any conflict of interest to report.
Disclosure statement
No potential conflict of interest was reported by the author(s).
References
- Butt MS, Naz A, Sultan MT, et al. Anti-oncogenic perspectives of spices/herbs: a comprehensive review. EXCLI J. 2013;12:1043–1065.
- Jamal A, Javed K, Aslam M, et al. Gastroprotective effect of cardamom, Elettaria cardamomum Maton, fruits in rats. J Ethnopharmacol. 2006;103:149–153.
- Gilani AH, Jabeen Q, Khan AU, et al. Gut modulatory, blood pressure lowering, diuretic and sedative activities of cardamom. J Ethnopharmacol. 2008;115:463–472.
- Radomska-Leśniewska DM, Skopiński P, Niemcewicz M, et al. The effect of anti-inflammatory and antimicrobial herbal remedy PADMA 28 on immunological angiogenesis and granulocytes activity in mice. Mediators Inflamm. 2013;2013:853475.
- Das I, Acharya A, Berry DL, et al. Antioxidative effects of the spice cardamom against non-melanoma skin cancer by modulating nuclear factor erythroid-2-related factor 2 and NF-κB signalling pathways. Br J Nutr. 2012;108(6):984–997.
- Alam A, Rehman NU, Ansari MN, et al. Effects of essential oils of Elettaria cardamomum grown in India and Guatemala on gram-negative bacteria and gastrointestinal disorders. Molecules. 2021;26:9.
- Zhihua Z, Jianping Y, Miaomiao S, et al. Exploration for the multi-effect of cardamom in’s resistance to multiple myeloma. Pak J Pharm Sci. 2014;27:2001–2006.
- Daneshi-Maskooni M, Keshavarz SA, Mansouri S, et al. The effects of green cardamom on blood glucose indices, lipids, inflammatory factors, paraxonase-1, sirtuin-1, and irisin in patients with nonalcoholic fatty liver disease and obesity: study protocol for a randomized controlled trial. Trials. 2017;18:260.
- Goyal SN, Sharma C, Mahajan UB, et al. Protective effects of cardamom in isoproterenol-induced myocardial infarction in rats. Int J Mol Sci. 2015;16(11):27457–27469.
- Bhaswant M, Poudyal H, Mathai ML, et al. Green and black cardamom in a diet-induced rat model of metabolic syndrome. Nutrients. 2015;7:7691–7707.
- Bhat GM N, Nayak N, Vinodraj K, et al. Comparison of the efficacy of cardamom (Elettaria cardamomum) with pioglitazone on dexamethasone-induced hepatic steatosis, dyslipidemia, and hyperglycemia in albino rats. J Adv Pharm Technol Res. 2015;6(3):136.
- Khan A, Khan QJ, Gilan AH. Pharmacological basis for the medicinal use of cardamom in asthma. Bangladesh J Pharmacol. 2011;6:34–37.
- Kumar KS, Unnisa A, Sushmitha KS, et al. Antidepressant activity of cardamom oil by marble burying test in rats. Der Pharmacia Lettre. 2016;8(3):279–282.
- Amma K, Rani MP, Sasidharan I, et al. Chemical composition, flavonoid – phenolic contents and radical scavenging activity of four major varieties of cardamom. Int J Biol Med Res. 2010;1:20–24.
- Figueiredo AC, Barroso JG, Pedro LG, et al. Factors affecting secondary metabolite production in plants: volatile components and essential oils. Flavour Fragr J. 2008;23:213–226.
- EFSA. (European Food Safety Authority). Safety and efficacy of an essential oil from Elettaria cardamomum (L.) Maton when used as a sensory additive in feed for all animal species. EFSA J. 2019;17:e05721.
- Hamzaa RG, Osman NN. Using of coffee and cardamom mixture to ameliorate oxidative stress induced in γ-irradiated rats. Biochem Anal Biochem. 2012;1:2161–1009.
- https://www.doterra.com/US/en/p/cardamom-oil (Excess on 23-10-2021).
- https://www.amazon.com/Edens-Garden-Essential-Therapeutic-Aromatherapy/dp/B0046ZTJH0?th=1 (Excess on 23-10-2021).
- al-Zuhair H, el-Sayeh B, Ameen HA, et al. Pharmacological studies of cardamom oil in animals. Pharmacol Res. 1996;34(1–2):79–82.
- Heimesaat MM, Mousavi S, Weschka D, et al. Anti-pathogenic and immune-modulatory effects of peroral treatment with cardamom essential oil in acute murine campylobacteriosis. Microorganisms. 2021;9(1):169.
- Abdullah A, Butt MS, Shahid M, et al. Evaluating the antimicrobial potential of Green cardamom essential oil focusing on quorum sensing inhibition of chromobacterium violaceum. J Food Sci Technol. 2017;54:2306–2315.
- Ashokkumar K, Vellaikumar S, Murugan M, et al. Essential oil profile diversity in cardamom accessions from southern India. Front Sustain Food Syst. 2021;5:137.
- Singh G, Kiran S, Marimuthu P, et al. Antioxidant and antimicrobial activities of essential oil and various oleoresins of Elettaria cardamomum (seeds and pods). J Sci Food Agric. 2008;88:280–289.
- Chowdhury S, Kumar S. Alpha-terpinyl acetate: A natural monoterpenoid from Elettaria cardamomum as multi-target directed ligand in Alzheimer’s disease. J Funct Foods. 2020;68:103892.
- Alam A, Majumdar RS, Alam P. Systematics evaluations of morphological traits, chemical composition, and antimicrobial properties of selected varieties of Elettaria cardamomum (L.) Maton. Nat Prod Commun. 2019;14(12):1–7.
- Kandikattu HK, Rachitha P, Jayashree GV, et al. Anti-inflammatory and anti-oxidant effects of cardamom (Elettaria repens (Sonn.) Baill) and its phytochemical analysis by 4D GCXGC TOF-MS. Biomed Pharmacother. 2017;91:191–201.
- Pizzino G, Irrera N, Cucinotta M, et al. Oxidative stress: harms and benefits for human health. Oxid Med Cell Longev. 2017;2017:8416763.
- Declercq JP, Evrard C, Clippe A, et al. Crystal structure of human peroxiredoxin 5, a novel type of mammalian peroxiredoxin at 1.5 A resolution. J Mol Biol. 2001;311(4):751–759.
- Li J, Pang Q. Oxidative stress-associated protein tyrosine kinases and phosphatases in Fanconi anemia. Antioxid Redox Signal. 2014;20(14):2290–2301.
- Hu C, Ma S. Recent development of lipoxygenase inhibitors as anti-inflammatory agents. Medchemcomm. 2017;9(2):212–225.
- Khan FM, Kader FB, Arman M, et al. Pharmacological insights and prediction of lead bioactive isolates of Dita bark through experimental and computer-aided mechanism. Biomed Pharmacother. 2020;131:110774.
- Dehsheikh A B, Sourestani M M, Dehsheikh P B, et al. A comparative study of essential oil constituents and phenolic compounds of Arabian Lilac (Vitex Trifolia var. Purpurea): An evidence of season effects. Foods. 2019;8(2):52.
- Wesołowska A, Jadczak P, Kulpa D, et al. Gas chromatography-mass spectrometry (GC-MS) analysis of essential oils from AgNPs and AuNPs elicited lavandula angustifolia in vitro cultures. Molecules. 2019;24(3):606.
- Adams RP. Identification of essential oil components by gas chromatography/quadrupole mass spectrometry. J Am Soc Mass Spectrom. 2007;16:1902–1903.
- Alam A, Rita SM. Antioxidant activity of essential oil of three cultivars of Amomum subulatum and standardization of high-performance thin layer chromatography (HPTLC) method for the estimation of 1,8-cineole. Afr J Biotechnol. 2018;17:1129–1137.
- Huang HC, Wang HF, Yih KH, et al. Dual bioactivities of essential oil extracted from the leaves of Artemisia argyi as an antimelanogenic versus antioxidant agent and chemical composition analysis by GC/MS. Int J Mol Sci. 2012;13(11):14679–14697.
- Alam A, Sing V. Composition and pharmacological activity of essential oils from two imported Amomum subulatum fruit samples. J Taibah Univ Med Sci. 2021;16:231–239.
- Gunathilake KDPP, Ranaweera KKDS, Rupasinghe HPV. In vitro anti-inflammatory Properties of selected green leafy vegetables. Biomedicines. 2018;6:107.
- Rizvi SM, Shakil S, Haneef M. A simple click by click protocol to perform docking: AutoDock 4.2 made easy for non-bioinformaticians. EXCLI J. 2013;12:831–857.
- Declercq JP, Evrard C, Clippe A, et al. Crystal structure of human peroxiredoxin 5, a novel type of mammalian peroxiredoxin at 1.5 A resolution. J Mol Biol. 2001;311(4):751–759.
- Busam RD, Lehtio L, Karlberg T, et al. Crystal structure of kinase domain of protein tyrosine kinase 2 beta (PTK2B). 2008. doi:https://doi.org/10.2210/pdb3CC6/pdb.
- Gilbert NC, Rui Z, Neau DB, et al. S663D Stable-5-LOX in complex with Arachidonic Acid. 2012. doi:https://doi.org/10.2210/pdb3V99/pdb.
- O’Boyle NM, Banck M, James CA, et al. Open babel: an open chemical toolbox. J Cheminform. 2011;3:33. https://doi.org/https://doi.org/10.1186/1758-2946-3-33.
- BIOVIA. Dassault Systèmes. In Discovery Studio Visualizer. v21.1.0.20298; Dassault Systèmes: San Diego, CA, USA; 2021.
- Filimonov DA, Lagunin AA, Gloriozova TA, et al. Prediction of the biological activity spectra of organic compounds using the PASS online web resource. Chem Heterocycl Compd. 2014;50(3):444–457.
- Daina A, Michielin O, Zoete V. SwissADME: a free web tool to evaluate pharmacokinetics, drug-likeness and medicinal chemistry friendliness of small molecules. Sci Rep. 2017;7:42717.
- Amma KPP, Sasidharan I, Sreekumar MM, et al. Total antioxidant capacity and change in phytochemicals of four major varieties of cardamom oils during decortication. Int J Food Prop. 2015;18:1317–1325.
- Snoussi M, Noumi E, Dehmani A, et al. Chemical composition and antimicrobial activities of Elettaria Cardamomum L. (Manton) essential oil: a high activity against a wide range of food borne and medically important bacteria and fungi. J Chem Bio Phy Sci. 2016;6:248–259.
- Leela NK, Prasath D, Venugopal MN. Essential oil composition of selected cardamom genotypes at different maturity levels. Indian J Hortic. 2008;65:366–369.
- Kumar A, Tandon S, Ahmad J, et al. Essential oil composition of seed and fruit coat of Elettaria cardamomum from South India. J Essent Oil-Bear Plants. 2005;8:204–207.
- Ivanović M, Makoter K, Islamčević Razboršek M. Comparative study of chemical composition and antioxidant activity of essential oils and crude extracts of four characteristic Zingiberaceae herbs. Plants (Basel). 2021;10(3):501.
- Ciftci O, Ozdemir I, Tanyildizi S, et al. Antioxidative effects of curcumin, β-myrcene and 1,8-cineole against 2,3,7,8-tetrachlorodibenzo-p-dioxin-induced oxidative stress in rats liver. Toxicol Ind Health. 2011;27:447–453.
- Bicas JL, Neri-Numa IA, Ruiz ALTG, et al. Evaluation of the antioxidant and antiproliferative potential of bioflavors. Food Chem Toxicol. 2011;49:1610–1615.
- Salehi B, Upadhyay S, Erdogan OI, et al. Therapeutic potential of α- and β-pinene: a miracle gift of nature. Biomolecules. 2019;9:738.
- Sharopov FS, Wink M, Setzer WN. Radical scavenging and antioxidant activities of essential oil components-an experimental and computational investigation. Nat Prod Commun. 2015;10:153–156.
- Han F, Ma GQ, Yang M, et al. Chemical composition and antioxidant activities of essential oils from different parts of the oregano. J Zhejiang Univ Sci B. 2017;18(1):79–84.
- Abu-Taweel GM. Cardamom (Elettaria cardamomum) perinatal exposure effects on the development, behavior and biochemical parameters in mice offspring. Saudi J Biol Sci. 2018;25(1):186–193.
- Kim MG, Kim SM, Min JH, et al. Anti-inflammatory effects of linalool on ovalbumin-induced pulmonary inflammation. Int Immunopharmacol. 2019;74:105706.
- Mahmud S, Uddin MAR, Zaman M, et al. Molecular docking and dynamics study of natural compound for potential inhibition of main protease of SARS-CoV-2. J Biomol Struct Dyn. 2020;24:1–9.
- Hsu KC, Huang Fu WC, Lin TE. A site-moiety map and virtual screening approach for discovery of novel 5-LOX inhibitors. Sci Rep. 2020;10:10510.
- Alminderej F, Bakari S, Almundarij TI, et al. Antioxidant activities of a new chemotype of Piper cubeba L. fruit essential oil (methyleugenol/eugenol): in silico molecular docking and ADMET studies. Plants (Basel). 2020;9(11):1534.
- Lipinski CA, Lombardo F, Dominy BW, et al. Experimental and computational approaches to estimate solubility and permeability in drug discovery and development settings. Adv Drug Deliv Rev. 2001;46(1–3):3–26.
- Bojarska J, Remko M, Breza M, et al. A supramolecular approach to structure-based design with a focus on synthons hierarchy in Ornithine-derived ligands: review, synthesis, experimental and in silico studies. Molecules. 2020;25(5):1135.
- Santos FA. 1,8-Cineol, a food flavoring agent, prevents ethanol-induced gastric injury in rats. Dig Dis Sci. 2001;46:331–337.
- Cutillas AB, Carrasco A, Martinez-Gutierrez R, et al. Thymus mastichina L. essential oils from Murcia (Spain): composition and antioxidant, antienzymatic and antimicrobial bioactivities. PLoS One. 2018;13(1):e0190790.