Abstract
The aim of this study was to isolate a filamentous nitrogen-fixing cyanobacterium Nostoc sphaeroides (NS) from brackish water of Chilika Lake, Odisha coast, and exploration of its use in the biosynthesis of silver-nanoparticles (AgNPs) against the urinary tract–infecting bacterial strains. The synthesized NS-AgNPs were structurally characterized by analytical techniques such as UV-visible-spectrophotometry, FTIR, XRD, SEM and SEM-EDX. The antibacterial efficacy of synthesized AgNPs had remarkable in vitro activities with the MIC value of 50 mg/ml. Moreover, Nostocine A, the inherent bioactive compound of Nostoc sp. was further investigated for molecular docking studies against the targeted bacterial DNA gyrase (Escherichia coli) and the obtained docking score was −7.9 kCal/mol as compared with the standard drug, Ciprofloxacin of a docking score −7.8 kCal/mol. The concordance between molecular docking and experimental results is excellent. Thus, the biogenic-synthesized “N. sphaeroides-AgNPs” would be used as an antibacterial agent in future.
1. Introduction
Urinary tract infection (UTI) has become a severe problem than imagined, because of the emergence of multidrug resistant (MDR) avatars of UTI-causing bacteria. The clinician has to treat the patient before receiving the urine-culture report. It is the commonplace ailment in women, and a clinician mostly administers empirical therapy antibiotics of some higher generations for the forthwith-control. Here, empirical therapy is the available alternative, as the UTI progresses to cystitis to pyelonephritis and ultimately to blood stream infections or toxic shock syndrome or even to lower chest infections ultimately [Citation1,Citation2]. It has been a commonplace event of antibiotic failures in clinical management of infectious diseases from bacterial multidrug resistance. Consequently, the clinician advises the administration of some antibiotics of higher generations to treat the urgent need of patients with UTI. This practice itself creates a vicious cycle of resistance of bacterial pathogens to the recently applied antibiotics and further introduction of still higher antibiotics against MDR bacterial strains continues [Citation3,Citation4]. Moreover, UTI accounts for nearly a 7 million clinical visits per year in the United States alone costing US$2 billion, annually and almost every woman suffers from UTI at least once in the life-time [Citation5]. UTIs are mainly caused by both Gram-positive and Gram-negative bacteria, although fungi and rarely some viruses could have also been implicated. Distinctively, MDR Escherichia coli strains cause UTI and the designated uropathogenic morbidities.
The problem could be addressed through the use of nanoparticles of non-common phytochemicals or whole plant extracts as reported often before [Citation6]. Several reports have accumulated on cyanobacterial bioactive compounds to address the other serious aliment such as cancer [Citation7,Citation8]. Thus, it is intuitive to imagine some novel phytocompound for a plausible control of UTI routinely. This problem could be addressed with silver-nanoparticles (AgNPs) synthesized with some non-edible natural chemicals. Earlier it had been a common practice to access the antimicrobial efficacy of most flowering plants, now it has been a newer practice to synthesize AgNPs from cyanobacteria [Citation9]. As the plant-synthesized AgNPs have the intrinsic antibacterial property and Ag compounds have antimicrobial activity, some suitable pant-based AgNPs should control the drug-resistant pathogenic bacteria.
Cyanobacteria (blue–green algae) with chlorophyll a similar to the higher plants are the oxygen-evolving photosynthetic bacteria having c-phycocyanin and c-phycoerithrin. Additionally, blue and red pigments enable them a ubiquitous distribution such as in marine waters, freshwaters, damp soils and moistened rocks. These are capable of switching the oxygenic photosynthesis to anoxygenic photosynthesis, in adaptation to the growing environment. Moreover, cyanobacterial morphology ranges from unicellular to filamentous forms; filaments may be either unbranched or branched without or with heterocysts, the sites of dinitrogen-fixation. The unbranched filamentous genus Nostoc sp. is heterocystous and lends itself in bio-fertilization of rice soils in N-deficient Asian rice soils [Citation10]. Indeed, these versatile cyanobacteria have a lot of intrinsic cyanobacterial compounds of curio, which are bioactive against microbial properties, examples shown in other studies [Citation11,Citation12]; however, cyano-compounds have not been seriously pursued for other bioactive therapeutic activities [Citation13]. Besides, Nostoc sp. has noteworthy fascination as the common wellspring of bioactivity with a scope of natural exercises, for example, antimicrobial, antiviral and anti-inflammatory capacities.
This investigation records the synthesis of AgNPs with a brackish-water cyanobacterium Nostoc sphaeroides using silver nitrate (AgNO3), and exploration of the synthesized AgNPs for antibacterial efficacy after due structural characterizations. The N. sphaeroides-AgNPs were assessed by UV-visible spectrophotometry and characterized by XRD (X-ray diffraction), FTIR (Fourier transform infrared spectroscopy), SEM (scanning electron microscopy) and SEM-EDX(energy-dispersive X-ray). The antibacterial potential of NS-AgNPs was evaluated against pathogenic strains of Staphylococcus aureus and E. coli, isolated from clinical samples. Moreover, the presented bioactive compound Nostocine A (an active chemical of N. sphaeroides) was assessed by the molecular docking studies with bacterial DNA gyrase as the targeted protein.
2. Materials and methods
2.1. Materials
This experimental work was executed by analytical grade procured from Sigma-Aldrich and Hi-media Pvt Ltd (Mumbai, India). The structural analysis of the synthesized nanoparticle was performed by ATR-FTIR (JASCO, 4100), XRD analysis by X-ray powder diffractometer (Rigaku Miniflex, Japan); and two pathogenic bacterial strains, Gram-positive S. aureus and Gram-negative E. coli, isolated from clinical samples collected from women reporting UTI ailment, in IMS &SUM Hospital, Bhubaneswar, Odisha were used.
2.2. Isolation and biomass growth of cyanobacterium
Field water samples with microalgae were collected during December–January 2017, from the brackish region with a large stretch of wetland habitats, Sipakuda, Satapada, Puri, Odisha (Figure ). The collected samples were treated for having a unialgal culture; the N2-fixing filamentous heterocystous blue–green alga (cyanobacterium), N. sphaeroides, family Nostocaceae, order Nostocales was obtained, identified by a research microscope (Magnus, MLXi model, India), and scanning electron microscope (SEM) (Hitachi, S3400N, Japan) for the assessment of surface morphology. Consequently, the obtained rDNA sequence (SRM-DBT Platform for Advanced Life Science Technologies) was analyzed with Finch-tv tool as FASTA format (pairwise sequence alignment). Furthermore, N. sphaeroides was grown in batch cultures in the modified CHU-10 media. The biomass was harvested in 3–4 weeks of continuous growth and each growth cycle was maintained under 24 ± 4°C temperature at a light intensity at 2000 ± 200 lux with the 12-h light/dark cycle. Obviously, the harvested biomass of N. sphaeroides was centrifuged at 400 rpm for 25 min, and the pellets were washed to a condition of moderate aqueous dryness. Moreover, the aqueous N. sphaeroides extract was adjusted to 10 mg wet weight per 10 ml of dry weight for 24 h; after the assorted extract was filtered and stored at 4°C for further analysis.
2.3. AgNP synthesis of N. sphaeroides extracts
AgNPs synthesized with N. sphaeroides (NS-AgNPs)were produced with a volume of 90 ml of 1 mM aqueous AgNO3 mixed with an aliquot of10 ml lot of N. sphaeroides extract in vitro for 24 h at 30°C under 2000 ± 200 lux light intensity, and further under light for 24–48 h, to visibly observe colour change of the developing NS-AgNPs in the conical flask; OD was taken at 200–700 nm. Thereafter, centrifugation of the synthesized AgNPs was performed at 10,000 rpm for 10 min and the supernatant was discarded, while the pellets were pooled and washed thrice at 30°C for further spectral analysis [Citation14].
2.4. Antibacterial activity of N. sphaeroides extracts
First, agar-well diffusion assay was performed using spread plate; a volume of 100 ml molten nutrient agar was poured onto sterile Petri plates that were allowed for drying; 6-mm diameter wells were bored in those Petri plates at two directions. Concomitantly, aliquots of 0.5 ml individual pathogenic strains of S. aureus and E. coli in fresh broth cultures were spread under aseptic conditions. Furthermore, aliquots of 5 ml of each mixture of the cyanobacterial extracts in solvents namely, ethanol, methanol, acetone and water extracts at the concentration (1 mg/ml) were poured; similarly, aliquots of 5 ml of NS-AgNPs were poured at 0.5 and 1.0 mg/ml concentrations. The zones of inhibitions were noted at the third day after immediate incubation at 37°C. Secondly, MIC assay methods were conducted according to the methods elaborated in previous studies [Citation15–17], as followed with serial dilutions of 25, 50, 75, 100, 200 and 500 mg extract and NS-AgNPs with the negative control, DMSO.
2.5. Molecular docking
In bioinformatics, molecular docking is a novel approach for the validation of efficacy properties. Indeed, target-oriented molecule protein could be used to design and bind to the ligand, the targeted protein in the docking process. Nostocine A is an active bioactive compound of Nostoc sp [Citation7]; the Nostocine A was drawn on the ACD/ChemSketch software with a fully geometric optimization with the open label programme. Moreover, E. coli DNA gyrase protein (PDBID:3G7E) was retrieved from protein data bank for docking purposes. After that, the molecular modelling of Nostocine A with E. coli DNA gyrase was carried out by AutoDock software v4.2.6. The receptor grid box size was 60 × 60 × 60 and the Lamarckian genetic algorithm was 1,000,000 for individual run with root mean square at 2.0°Å. The top-ranked docked score was recorded out of 100 docking runs. Then, the binding interaction of ligand–protein was visualized by BioVia-Discovery studiosoftwarev-2017 [Citation18,Citation19].
3. Results and discussion
3.1. Isolation of N. sphaeroides
Morphologically the unbranched filaments of N. sphaeroides were observed as mucilaginous, gelatinous and solid-hollow, free-floating as mass or lump, light-blue green to dark green in colour, with distinct sheath; individual cells were found to be of different stages like, spherical, sub-spherical or cylindrical with intercalary distinct sub-spherical cell small heterocysts. Particularly, N. sphaeroides had filament gelatinous, blue–green to dark, coiled, continuously growing on agar plates as trichome 3–5 µm broad (trichomes with sheath are called as filaments) (Figure ). Concomitantly, the confirmed rDNA sequence was submitted to National Center for Biotechnology Information (https://www.nih.gov/), and the obtained GenBank-accession number is MT974143. Furthermore, the growth of N. sphaeroides was monitored under 25 ± 3°C in the culture room, for 14-h light periods, and after the third cycle the biomass was harvested.
Figure 2. Clockwise representation morphology and surface morphology of N. sphaeroides assessed by scanning electron microscope.
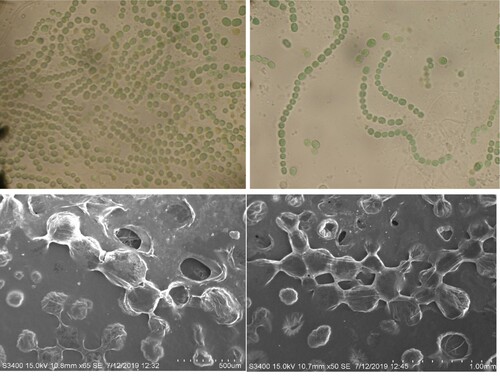
The currently used cyanobacterial sample was from the (meeting point of a river and sea) brackish water at sea-mouth; obviously, the distribution and diversity strongly depend upon the habitats [Citation20]. However, the filaments of the outer layer of colonies had the maximum photosynthetic rate at the highest saturation point; whereas, inner layers had the higher content of chlorophyll a and phycobiliproteins compared to the rate by the outer cell-layers of N. sphaeroides mass, assessed from a single filament to compact macro-colonies, as reported in the previous studies [Citation21,Citation22].
Moreover, in a freshwater rich in dissolved inorganic carbon, the growth rates were recorded as the maximum for N. commune and N. pruniforme with the doubling time 10–14 days. In another freshwater form, N. zetterstedtii with comparatively a lower ambient dissolved inorganic carbon, the growth rate was comparatively slow [Citation23]. N. flagelliforme was cultivated in a 20-L photo-bioreactor for 16 days with the impeller tip speeds at 0.3, 0.8, 1.0, and 1.5 ms−1. Moreover, in a desert, the growth rate of N. flagelliforme on wet sand was faster, and its cells adhered to the surface of sand particles forming a biological crust [Citation24]. Moreover, Nostoc sp. produced rich-bioactive compounds with rapid growth with biomass scaled up to of 400 g (wet weight/l) in another study [Citation25].
3.2. Synthesis and characterization of AgNPs
The N. sphaeroides extract was treated with several concentrations of AgNO3, and the reaction mixture turned to light bud green to deep olive black colour within 24–48 h. The reaction mixture was scanned at 200–700 nm by a spectrophotometer for quantitative estimation of metal ions, organic compounds and a few more analyses (Figure ). The synthesis of NS-AgNPs was confirmed by FTIR for analyses of infrared spectrum of absorbance at 3612, 3652 (O–H str.), 2918(N–H str.), 2320, 2373 (O=C=Ostr.), 1537 (N–O str.) and 1370 cm-1 (Figure ). Furthermore, the XRD spectral pattern of 2θ had shown the formation of crystals at 5–80θ at the ranges 38.5°, 45.3°, 64° and 75.3° (Figure ).
Figure 3. UV-Vis spectra of AgNPs with N. sphaeroides after 48 h for the synthesis at 28°C. colour deviation, at various time intervals 2, 24 and 48 h (A–C).
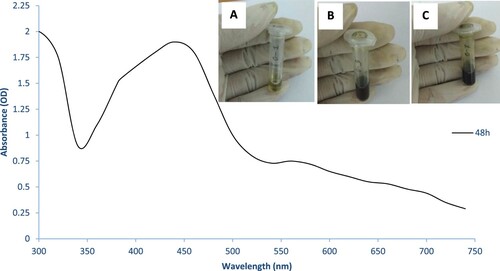
Furthermore, SEM and SEM-EDX (energy-dispersive X-ray) image of synthesized AgNPs were confirmed by surface structure and the presence of Ag nanoparticles (Figure ), and the particle size at ranges 30–90 nm and the average size was calculated at 60 nm (Figure ). However, the maximum rate of absorption of plant-mediated synthesis at 450–460 nm with various time intervals, extended up to 5days [Citation26]. Consequently, the confirmed FTIR spectra at 3323 cm−1 have reported synthesis with AgNPs with citrus fruit peel [Citation27]. Similarly, XRD spectral pattern have reported at various 2θ ranges namely, 38°, 44° and 64° of annual vinca [Citation28].
3.3. MIC and zone of inhibition measurement
Various concentrations of N. sphaeroides solvent extracts were taken against the isolated pathogenic bacteria, S. aureus and E. coli. Ethanol, methanol, acetone and water extracts were used for agar-well diffusion method for monitoring antibacterial efficacy, and the highest zone of inhibition of cell layers was 10–14 mm (Figure ). Whereas, the synthesized NS-AgNPs had significant in vitro antagonistic effect against E. coli only at MIC assay values at 50 mg/ml (Figure ).
Figure 9. MIC assay of NS-AgNPs, against S. aureus (A, B, C) and E. coli (E, F, G) with various concentrations and brick colour represents as bacterial growth inhibition. The insert in the bar-chart is an image of a 96-well MIC plate.
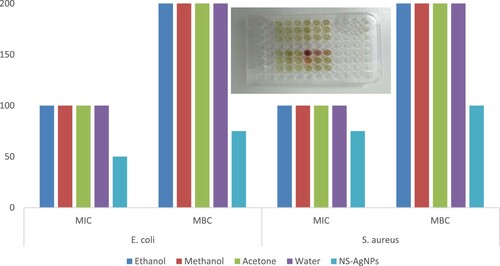
However, cell extracts with solvents, acetone, chloroform, methanol, petroleum aether and water were reported in the literature after monitoring the antibacterial activities with the agar-well diffusion method. The acetone extract had the maximum antibacterial activity against Salmonella typhi with the zone of 15 ± 0.35 and 12.66 ± 0.20, respectively [Citation29], in the he in vitro study of antibacterial activity of several organic extracts of cyanobacterium Anabaena sp. against S. aureus. The methanol extract had the largest zone-size of inhibition, compared to another solvent extract [Citation30]. N. sphaeroides had in vitro antibacterial activity against bacteria E. coli and Bacillus cereus [Citation31]. Further studies are obligatory in locating actual mechanism of biosynthesized NPs and notorious bacterial strains. Moreover, biosynthesis is less toxic, cost effective and feasible preparatory method. Obviously, crude extracts were seen with less side effects [Citation32,Citation33].
3.4. Molecular docking
The molecular docking study was performed against the bacterial DNA gyrase PDBID:3G7E with algal bioactive compound, Nostocine A and the obtained docking score was −7.9 kCal/mol as compared with the standard drug, Ciprofloxacin of a docking score −7.8 kCal/mol. Moreover, molecular binding interactions of amino acid residues of Nostocine A had interacted with conventional H-bond at Phe 90, whereas Ciprofloxacin had interacted at Phe 90 and Arg 62. Likewise, both these ligands were interacted with π-anion at Glu 36 position of the bacterial gyrase receptor (Figure ). Thus, the antibacterial effectivity of Nostocine A, seen through the docking study was almost comparable to the standard drug. Moreover, cyanobacteria are an important source of novel bioactive secondary metabolites such as aoratoxins, lynbyabellin and curacin. Being photosynthetic prokaryotes, cyanobacteria possess a bandwagon of natural chemicals of structures differing on a particular taxon, based on specific environmental condition(s) and on the diverse inherent metabolism(s), such as oxygenic or anoxygenic photosynthesis, being chlorophyllous even, capability to grow heterotrophically whenever required by shifting of metabolism, N2-fixing or a non-N2-fixing taxon and so on. These novel compounds were used for drug-designing using the computational approach [Citation13]. Marine strains of cyanobacteria produce several bioactive secondary metabolites, some of which are known to be highly cytotoxic compounds, namely, hectochlorin, lyngbyabellins, apratoxins dolastatins and aunilides that are identified as potential-lead compounds for the development of anticancer agents [Citation34]. Indeed, Cyabastacines A and B, isolated from Nostoc sp. were reported as potent antibacterial agents [Citation35]. Thus, the present NS-AgNPs work is an extension or offshoot in the drug-development endeavour with principles of bioinformatics aiding to manipulation of chemicals for locating suitable most hybrid-chemical as possible future antibacterial(s).
4. Conclusion
In this study, biogenic synthesis of AgNPs was described from N. sphaeroides biomass extract acting as reducing agent with an average size of 60 nm and confirmed the synthesis by UV-vis, FTIR, XRD, SEM and SEM-EDX analyses. Furthermore, this study with the synthesized NS-AgNPs and N. sphaeroides biomass, independently proved potencies for antibacterial in vitro activities, against the most frequently infecting uropathogens. Consequently, molecular docking study of the bioactive compound of Nostoc sp., Nostocine A, as a corollary has comparatively greater binding affinity with the bacterial DNA gyrase proving the efficacious capability of Nostoc sp. Having antibacterial property. Thus, the use of secondary metabolites from N. sphaeroides would provide potentiality for enrichments of drugable antibacterials for pharmaceutical industries.
Acknowledgements
C.R. Sahoo was supported by the PhD fellowship grant (No. 1781611002) of Siksha “O” Anusandhan Deemed to be University, Bhubaneswar, Odisha and Indian Council of Medical Research-Senior Research Fellowship grant (No. 45/3/2020-DDI/BMS). The authors are thankful to the central instrumentation facility, OUAT, Bhubaneswar, Odisha. Alexander M. Luke is grateful to the authorities of Ajman University for their encouragements.
Disclosure statement
No potential conflict of interest was reported by the author(s).
Additional information
Funding
References
- Foxman B. The epidemiology of urinary tract infection. Nat Rev Urol. 2010;7:653–660. doi:https://doi.org/10.1038/nrurol.2010.190.
- Kunter U. Urinary tract infections. Nephrologe. 2020;15:119–131. doi:https://doi.org/10.1007/s11560-020-00408-8.
- Baker SJ, Payne DJ, Rappuoli R, et al. Technologies to address antimicrobial resistance. Proc Natl Acad Sci U.S.A. 2018;115:12887–12895. doi:https://doi.org/10.1073/pnas.1717160115.
- Vihta KD, Stoesser N, Llewelyn MJ, et al. Trends over time in Escherichia coli bloodstream infections, urinary tract infections, and antibiotic susceptibilities in Oxfordshire, UK, 1998–2016: a study of electronic health records. Lancet Infect. Dis. 2018;18:1138–1149. doi:https://doi.org/10.1016/S1473-3099(18)30353-0.
- Flores-Mireles AL, Walker JN, Caparon M, et al. Urinary tract infections: epidemiology, mechanisms of infection and treatment options. Nat Rev Microbiol. 2015;13:269–284. doi:https://doi.org/10.1038/nrmicro3432.
- Mishra MP, Padhy RN. Antibacterial activity of green silver nanoparticles synthesized from Anogeissus acuminata against multidrug resistant urinary tract infecting bacteria in vitro and host-toxicity testing. J Appl Biomed. 2018;16:120–125. doi:https://doi.org/10.1016/j.jab.2017.11.003.
- Sahoo CR, Paidesetty SK, Padhy RN. Nornostocine congeners as potential anticancer drugs: an overview. Drug Dev Res. 2019;80:878–892. doi:https://doi.org/10.1002/ddr.21577.
- Sahoo CR, Paidesetty SK, Padhy RN. Norharmane as a potential chemical entity for development of anticancer drugs. Eur J Med Chem. 2019;162:752–764. doi:https://doi.org/10.1016/j.ejmech.2018.11.024.
- Sahoo CR, Maharana S, Mandhata CP, et al. Biogenic silver nanoparticle synthesis with cyanobacterium Chroococcus minutus isolated from Baliharachandi sea-mouth, Odisha, and in vitro antibacterial activity. Saudi J Biol Sci. 2020;27:1580–1586. doi:https://doi.org/10.1016/j.sjbs.2020.03.020.
- Padhy RN, Nayak N, Dash-Mohini RR, et al. Growth, metabolism and yield of rice cultivated in soils amended with fly ash and cyanobacteria and metal loads in plant parts. Rice Sci. 2016;23:22–32. doi:https://doi.org/10.1016/j.rsci.2016.01.003.
- Nayak N, Padhy RN, Singh PK. Evaluation of antibacterial and antioxidant efficacy of the fern Azolla caroliniana symbiotic with the Cyanobacterium Anabaena azollae. Proc Natl Acad Sci India Sect B - Biol Sci. 2015;85:555–569. doi:https://doi.org/10.1007/s40011-014-0370-3.
- Najdenski HM, Gigova LG, Iliev II, et al. Antibacterial and antifungal activities of selected microalgae and cyanobacteria. Int J Food Sci Technol. 2013;48:1533–1540. doi:https://doi.org/10.1111/ijfs.12122.
- Vijayakumar S, Menakha M. Pharmaceutical applications of cyanobacteria – a review. J Acute Med. 2015;5:15–23. doi:https://doi.org/10.1016/j.jacme.2015.02.004.
- Kathiraven T, Sundaramanickam A, Shanmugam N, et al. Green synthesis of silver nanoparticles using marine algae Caulerpa racemosa and their antibacterial activity against some human pathogens. Appl Nanosci. 2015;5:499–504. doi:https://doi.org/10.1007/s13204-014-0341-2.
- Mishra MP, Rath S, Swain SS, et al. In vitro antibacterial activity of crude extracts of 9 selected medicinal plants against UTI causing MDR bacteria. J King Saud Univ - Sci. 2017;29:84–95. doi:https://doi.org/10.1016/j.jksus.2015.05.007.
- Baral N, Mohapatra S, Raiguru BP, et al. Microwave-assisted rapid and efficient synthesis of new series of chromene-based 1,2,4-oxadiazole derivatives and evaluation of antibacterial activity with molecular docking investigation. J Heterocycl Chem. 2019;56:552–565. doi:https://doi.org/10.1002/jhet.3430.
- Mishra NP, Mohapatra S, Sahoo CR, et al. Design, one-pot synthesis, molecular docking study, and antibacterial evaluation of novel 2H-chromene based imidazo[1,2-a]pyridine derivatives as potent peptide deformylase inhibitors. J Mol Struct 2021;1246:131183. doi:https://doi.org/10.1016/j.molstruc.2021.131183.
- Sahoo CR, Paidesetty SK, Dehury B, et al. Molecular dynamics and computational study of Mannich-based coumarin derivatives: potent tyrosine kinase inhibitor. J Biomol Struct Dyn. 2020;38:5419–5428. doi:https://doi.org/10.1080/07391102.2019.1701554.
- Sahoo CR, Paidesetty SK, Sarathbabu S, et al. Molecular dynamics simulation, synthesis and topoisomerase inhibitory actions of vanillin derivatives: a systematic computational structural integument. J Biomol Struct Dyn. 2021; 1–11. doi:https://doi.org/10.1080/07391102.2021.1961867
- Lin CS, Chou TL, Wu JT. Biodiversity of soil algae in the farmlands of mid-Taiwan. Bot Stud. 2013;54. doi:https://doi.org/10.1186/1999-3110-54-41.
- Deng Z, Hu Q, Lu F, et al. Colony development and physiological characterization of the edible blue-green alga, Nostoc sphaeroides (Nostocaceae, Cyanophyta). Prog Nat Sci. 2008;18:1475–1483. doi:https://doi.org/10.1016/j.pnsc.2008.03.031.
- Mollenhauer D, Bengtsson R, Lindstrøm EA. Macroscopic cyanobacteria of the genus nostoc: a neglected and endangered constituent of European inland aquatic biodiversity. Eur J Phycol. 1999;34:349–360. doi:https://doi.org/10.1080/09670269910001736412.
- Sand-Jensen K. Ecophysiology of gelatinous Nostoc colonies: unprecedented slow growth and survival in resource-poor and harsh environments. Ann Bot. 2014;114:17–33. doi:https://doi.org/10.1093/aob/mcu085.
- Yue S, Liu J, Zheng R, et al. The growth of Nostoc flagelliforme cells in desert soil and its ecological effects. Adv Mater Res. 2012, 518–523: 5500–5505. doi:https://doi.org/10.4028/www.scientific.net/AMR.518-523.5500.
- Guljamow A, Kreische M, Ishida K, et al. High-density cultivation of terrestrial Nostoc strains leads to reprogramming of secondary metabolome. Appl Environ Microbiol. 2017;83; doi:https://doi.org/10.1128/AEM.01510-17.
- Kumar V, Singh S, Srivastava B, et al. Green synthesis of silver nanoparticles using leaf extract of Holoptelea integrifolia and preliminary investigation of its antioxidant, anti-inflammatory, antidiabetic and antibacterial activities. J Environ Chem Eng. 2019;7; 103094. doi:https://doi.org/10.1016/j.jece.2019.103094.
- Dutta T, Ghosh NN, Das M, et al. Green synthesis of antibacterial and antifungal silver nanoparticles using citrus limetta peel extract: experimental and theoretical studies. J Environ Chem Eng. 2020;8; 4, 104019. doi:https://doi.org/10.1016/j.jece.2020.104019.
- Rohaizad A, Shahabuddin S, Shahid MM, et al. Green synthesis of silver nanoparticles from catharanthus roseus dried bark extract deposited on graphene oxide for effective adsorption of methylene blue dye. J Environ Chem Eng. 2020;8; 4, 103955 doi:https://doi.org/10.1016/j.jece.2020.103955.
- Rao BD. (2015). Antibacterial activity of fresh water Cyanobacteria.
- Bhattacharyya S, Deep R, Nayak B, et al. Antimicrobial activity of two diazotropic cyanobacteria against Staphylococcus aureus. Int J Med Arom Plants. 2013;3:2249–4340. http://www.openaccessscience.com.
- Shaieb FA, Issa AA-S, Meragaa A. Antimicrobial activity of crude extracts of cyanobacteria Nostoc commune and Spirulina platensis, Arch. Biomed Sci. 2014;2:34–41. http://www.journals.tmkarpinski.com/index.php/abms/article/view/221.
- Hamida RS, Abdelmeguid NE, Ali MA, et al. Synthesis of silver nanoparticles using a novel cyanobacteria desertifilum sp. extract: their antibacterial and cytotoxicity effects. Int J Nanomedicine. 2020;15:49–63. doi:https://doi.org/10.2147/IJN.S238575.
- Hamida RS, Ali MA, Goda DA, et al. Cytotoxic effect of green silver nanoparticles against ampicillin-resistantKlebsiella pneumoniae. RSC Adv. 2020;10:21136–21146. doi:https://doi.org/10.1039/d0ra03580g.
- Tan LT. Bioactive natural products from marine cyanobacteria for drug discovery. Phytochemistry. 2007;68:954–979. doi:https://doi.org/10.1016/j.phytochem.2007.01.012.
- Cabanillas AH, Tena Pérez V, Maderuelo Corral S, et al. Cybastacines A and B: antibiotic sesterterpenes from a nostoc sp. cyanobacterium. J Nat Prod. 2018;81:410–413. doi:https://doi.org/10.1021/acs.jnatprod.7b00638.