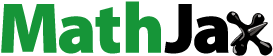
Abstract
The potentiometric method is adopted to determine formation constants of complexes and used with a new numerical scheme. This study deals with the coordination behaviour of L-DOPA ((3,4-dihydroxyphenyl)alanine) in Hg(II)/Pb(II)/Cd(II)-DOPA-L-alanine/glycine/L-phenylalanine (1:1:1) systems. DOPA is recognized as a neurotransmitter, and used in the treatment of Parkinson’s disease. All the selected ligands are biologically active and play a vital role in the physiological processes. All the systems were investigated pH-metrically at 20±1°C and 30±1°C at three different ionic strengths in aqueous medium. Stability Constants of Generalized Species’ computer program was used to obtain refined values of formation constants. Thermodynamic parameters (logkμ→ 0, ΔGo, ΔHo, ΔSo) for biligand systems were calculated. The speciation of different species in mixed coordinated systems was obtained up to a specific pH in particular system. High values of formation constants support the formation of stable ternary complexes. Biligand complexes involving alanine were found to be the most stable, while those of phenylalanine were the least with lowest concentration at a particular pH in specific conditions. Results obtained are helpful in understanding the physiological behaviour of these systems.
1. Introduction
Bioaccumulation and detoxification of heavy metals involve metal chelation [Citation1]. Such complexation reactions in biological relevance have promoted various research investigations [Citation2–5], which are helpful in designing therapeutic methodologies. Few monoligand and biligand systems of DOPA and related neurotransmitters have been investigated by different groups of reseachers [Citation6–9]. Nair et.al. carried out work on the mixed coordinated systems of DOPA with nickel and zinc in an aqueous medium and adopted a computer-aided approach for finding the results [Citation6]. The speciation of ternary complexes of lead(II), cadmium(II) and mercury(II) with L-dopa and phenanthroline in propanediol–water mixtures was obtained by Padmaja et al. [Citation7]. Potentiometric investigations on catecholamine and catechol were reported by R. Nair (Ahuja) et al., in an aqueous medium [Citation8,Citation9]. Interesting results of these researchers prompted computer-simulated investigations on the selected systems in an aqueous medium.
To understand the compatibility and toxic interference of heavy metals in the central nervous system, studies were done on nutrient and toxic metal ions and biologically potential ligands, including DOPA. Results obtained for NiII, CoII and CdII with DOPA, dopamine and tyrosine were reported earlier [Citation10–16]. The present study deals with the thermodynamic stabilities of HgII/PbII/CdII-DOPA-Ala/Gly/Phe complexes (where, DOPA = L-(3,4-dihydroxyphenyl)alanine, Ala = L-alanine, Gly = glycine, Phe = L-phenylalanine).
All the selected ligands are structurally related and directly or indirectly play a vital role in physiology and Central Nervous System (CNS) [Citation17]. These are synthesized in the body according to the following sequence:
DOPA acts as a precursor to DOPM (Dopamine) in CNS and is involved in nerve transduction [Citation18]. Lack of DOPM causes Parkinson’s disease, in which a person loses its ability to move controlled and smooth actions [Citation19]. DOPA is now being used to treat Parkinson’s disease and manganese poisoning [Citation20–22].
2. Material and method
All the reagents used were of the highest purity Merck/Aldrich products. Solutions were prepared in doubly distilled CO2-free water having pH ≈ 6.8 by using a standard method. All the titration mixtures were prepared in the same manner as published elsewhere [Citation11] and titrated against 0.10 M NaOH solution. Studies were carried out at three different ionic strengths (μ = 0.05M, 0.10M, 0.15M (NaNO3)) using an Elico digital pH-meter model LI-127 with the ATC probe and combined electrode type (CL-51B-Glass Body; range 0–14 pH unit; 0–100°C Automatic/Manual) with an accuracy of ±0.01. To find out thermodynamic parameters, titrations were done at two randomly selected temperatures (at 20 ± 1°C and 30 ± 1°C).
For each system pH was plotted against “a” to obtain titration curves (where “a” is the moles of alkali added per mole of metal/ligand). Figure shows representative titration curves at 30 ± 1°C. A Theoretical Composite Curve was obtained by the theoretical addition of metal–ligand B titration curve with a ligand A titration curve.
Figure 1. Representative pH vs. “a” curves, where Curve 1 represents ligand “A” (DOPA) titration curve, Curve 2 represents ligand “B” (Ala/Gly/Phe) titration curve, Curve 3 represents metal–ligand “A” (1:1) titration curve, Curve 4 represents metal–ligand “B” (1:1) titration curve, Curve 5 represents mixed-ligand (1:1:1) titration curve, and Curve “T” represents theoretical composite curve.
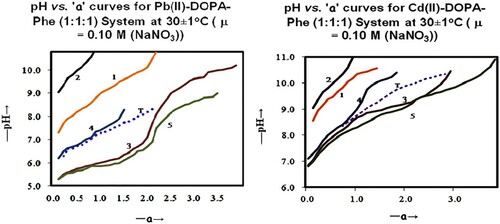
Experimental data were subjected to the algebraic method of Chaberek and Martell [Citation23,Citation24] as modified by Dey et al. [Citation25] to calculate formation constants. These values were refined by the SCOGS computer program [Citation26–28] extrapolated to zero ionic strength in log K and (square root of the ionic strength) plot to obtain thermodynamic formation constants. Values of ΔG˚, ΔH˚ and ΔS˚ for mixed coordinated complexes were calculated using Gibb’s Helmholtz equation, van’t Hoff isotherm and van’t Hoff isochore. All these results are depicted in Tables and .
Table 1. Protonation/dissociation constants of Ligands (log βμ→0) and thermodynamic formation constants of binary complexes in equimolar systems.
Table 2. Thermodynamic parameters for biprotonated M(II)-DOPA-ligand 'B' biligand complexes in equimolar systems.
3. Results and discussion
Protonation constants of ligands and the thermodynamic formation constants of binary complexes are given in Table .
The plot of experimental data is given in Figure . Titration curves for HgII and PbII follow the same trend (Figure ), and the pattern of curves for CdII systems is similar to those of CoII-DOPA-Ala/Phe systems [Citation11], as shown in Figure .
The nature of titration curves supports the formation of all the mixed coordinated complexes simultaneously (Scheme 1), as was predicted using the method of Carey and Martell [Citation29]. Furthermore, the predictions are supported by quantitative results obtained in the form of speciation curves (Figures and ).
Figure 2. Representative speciation curves for HgII/PbII-DOPA-Ala/Gly/Phe systems, where Curve 1: [M]; 2: [DOPA]; 3: [ligand B]; 4: [MAH2]; 5: [MB]; and 6: [MABH2].
![Figure 2. Representative speciation curves for HgII/PbII-DOPA-Ala/Gly/Phe systems, where Curve 1: [M]; 2: [DOPA]; 3: [ligand B]; 4: [MAH2]; 5: [MB]; and 6: [MABH2].](/cms/asset/c909a156-3b59-4fc8-bb9c-03252d831927/tusc_a_2007635_f0002_oc.jpg)
Figure 3. Representative speciation curves for CdII-DOPA-Ala/Gly/Phe systems, where Curve 1: [M]; 2: [DOPA]; 3: [ligand B]; 4: [MAH2]; 5: [MB]; and 6: [MABH2].
![Figure 3. Representative speciation curves for CdII-DOPA-Ala/Gly/Phe systems, where Curve 1: [M]; 2: [DOPA]; 3: [ligand B]; 4: [MAH2]; 5: [MB]; and 6: [MABH2].](/cms/asset/16ae6c6a-4edb-4bec-8cb7-2b6c20f39016/tusc_a_2007635_f0003_oc.jpg)
Thermodynamic formation constants for biligand systems and other thermodynamic parameters are given in Table .
HgII and PbII coordinate with ligands at a lower pH (near pH 6.0), while CdII coordinates above pH 7.0. A buffer region is observed near pH 6.5 in HgII/PbII-DOPA systems and at pH ≈ 9.0 in the systems of CdII-DOPA (curves 3 and 5 in Figure ), representing a change in the coordination mode of DOPA from aminocarboxylic to catecholic mode.
The complexation behaviour of DOPA is supported by spectroscopic studies done by Boggess and Martin [Citation30]. Boggess et.al. explained the displacement of bound glycinate site (aminocarboxylic end) to catecholate locus above pH 8.0 on the basis of absorption spectra. At a higher pH, DOPA is likely to form polymeric species [Citation30,Citation31]. Hence, the calculations for the monoligand and biligand complexes of DOPA were confined below pH 7.0 in the systems involving HgII and PbII and up to pH 9.0 in the systems of CdII, to avoid errors. The speciation of various monoligand and biligand species is also given to the confined pH in the respective systems (Figures and ).
Speciation curves obtained for PbII/HgII-DOPA systems show the formation of the mixed coordinated complex above pH 5.0 (Figure ). The concentration of monoligand species is low in the systems of lead. While in HgII and CdII systems, the monoligand and biligand species exist at the initial pH of complex formation. Both these complexes are formed up to pH 5.5 in HgII systems and up to pH 7.0 in the systems of CdII. Above this pH in respective systems, a biligand complex is formed by simultaneous coordination of metal and ligands as well as by the combination of monoligand complexes with other ligands (Scheme 1).
where M = Metal ion, A = L-DOPA, B = L-alanine/glycine/L-phenylalanine.
Species distribution of metal in the systems of phenylalanine show the percentage of maximal formation of MAH2B complex is lower than other systems due to the repulsion between bulky phenyl rings of DOPA and phenylalanine.
Results obtained for biligand systems are incorporated in Table . These results show that the order of stability of complexes is
This can be explained on account of the structure of these ligands. The highest stability of complexes of alanine is due to the positive inductive effect of the methylene group of the ligand. While a lower stability of biligand complexes of phenylalanine is attributed to the electron withdrawing nature of phenyl ring of a particular ligand and to the staric hindrance between two bulky ligands.
The stability order of complexes with respect to metal ions is in the sequence of their hydrolysis, which is given as follows:
This order can be explained on the basis of the charge to radius ratio.
4. Conclusion
Results show that the ternary complexes of alanine are most stable, and those of phenylalanine are least stable. As far as metal ions are concerned, complexes of mercury are most stable, and those of the cadmium are least stable.
The present work is helpful in understanding the in vivo stability and biochemical behaviour of selected ligands with the selected metal ions. All the selected ligands play a vital role in the Central Nervous System. Hence this study is helpful for biochemists to understand the compatibility and toxic interference of selected heavy metal ions in the Central Nervous System. These data are useful in designing complexo-therapeutic treatment for such metal toxicity. An alternative model for the species distributions from potentiometric data, in thermodynamic equilibrium, based on the models used for HySS or Hyperquad, and titration curve has also a bottle neck [Citation32] and is expected to be recognized shortly.
Acknowledgements
Dr. Neerja Upadhyaya is thankful to Jiwaji University, Gwalior, M.P., where the work was carried out. Thanks are also due to Dr. Neelima Kulkarni, Prof., Deptt. of Chemistry, M.S. University, Baroda, Gujarat, for extending her help.
Disclosure statement
No potential conflict of interest was reported by the author(s).
References
- Chauhan ZH, Mohammad SI, Syed KA, et al. Antibacterial dimeric copper(II) complexes with chromone-derived compounds. Inhib Med Chem. 2012;27(2):223.
- Al-Mohaimeed AM, Alothman AA. Characterization by potentiometric procedures of the stability constants of the binary and ternary complexes of Cu(II) and duloxetine drug with amino acids. J Chem. 2019;2019:1064942.
- Devi M, Nair (Ahuja) R. Speciation studies of dimethyltin (IV) and trimethyltin (IV) - carboxylates systems. Int J Sci Res. 2015;4(2):1359.
- Fedorov Yu V, Fedorov OA, Kalmykov SN, et al. Potentiometric studies of complex formation of amidopyridine macrocycles bearing pendant arms with proton and heavy metal ions in aqueous solution. Polyhedron. 2017;124:229.
- Al-Rashdi1 AA, Naggar AH, Farghaly OA, et al. Potentiometric and conductometric studies of sulfathiazole: glycine binary and ternary complexes. Int J Electrochem Sci. 2019;14:1132–1146.
- Nair MS, Arasu PT, Neelkantan MA., et al. Stability and structure of copper (II), nickel (II) and zinc (II) heterobinuclear complexes containing some biologically important ligands . Ind J Chem A. 1995;34(A):365.
- Padmaja N, Rao GN. Speciation of ternary complexes of lead(II), cadmium(II) and mercury(II) with L-dopa and phenanthroline in propanediol-water mixtures. Chem Speciation Bioavailability. 2012;24(4):234.
- Gupta J, Nair(Ahuja) R, Dwivedi K. Solution studies of Cd(II) and Gd(III) involving malonic acid and some catecholamines. Int J Sci Res. 2015;4(6):1266.
- Devi M, Nair (Ahuja) R, Gupta J, et al. Interaction of Me2Sn (IV) 2+ and Me3Sn (IV) + cations with catecholamines in aqueous media. IOSR J Appl Chem. 2015;8(3):52.
- Dwivedi N, Dwivedi K, Nair(Ahuja) R. Speciation of protonated and nonprotonated complex species in biologically important mixed-ligand systems of dopamine (DOPM). Orient J Chem. 2008;24(2):521.
- Dwivedi(Upadhyaya) N, Dwivedi K, Nair(Ahuja) R. Complexation behavior of (3,4 – dihydroxyphenyl) alanine in some mixed coordinated systems: equilibrium studies. J Indian Chem Soc. 2009;86:352.
- Yadav SK, Upadhyaya(Dwivedi) N, Nair(Ahuja) R, et al. Solution study of ternary systems involving transition metals, dipeptide and catecholic ligands. J Indian Chem Soc. 2011;88:1599.
- Upadhyaya N, Dwivedi K, Nair(Ahuja) R. Computer simulated distribution of co(II) in protonated and nonprotonated biligand species involving some neurotransmitters. Int J Innov Res Sci Eng Technol (IJIRSET). 2016;5(7):12202.
- Upadhyaya N, Dwivedi K, Nair(Ahuja) R. Speciation of Cd(II) as a function of pH in ternary systems involving some potential ligands. Der Pharma Chem. 2016;8(10):32.
- Upadhyaya N, Dwivedi K, Nair(Ahuja) R. Solution studies on biologically relevant biligand systems involving L-tyrosine. J Chem Chem Sci. 2017;7(7):532.
- Upadhyaya N, Nair(Ahuja) R. Percentage distribution of Ni(II) in biligand systems involving some biologically significant ligands. Asian J Res Chem. 2017;10(5):193–197.
- Smith C.U.M., Elements of molecular biology. 2nd ed. Chichester: John Wiley & Sons; 1996, p. 324.
- Balasubramanian D. High speed knockout in the brain. ‘Everyman’s Sci. 2006;XLI(1):68.
- Lemke MR, Brecht HM, Koester J, et al. Anhedonia, depression, and motor functioning in Parkinson’s disease during treatment with pramipexole. J Neuropsychiatry Clin Neurosci. 2005;17(2):214–220.
- Vanden HDMA, Pasterkamp RJ. Getting connected in the dopamine system. Prog Neurobiol. 2008;85:75.
- Recalcati S, Invernizzi P, Arosio P, et al. New functions for an iron storage protein: the role of ferritin in immunity and autoimmunity. J Autoimmun. 2008;30(1-2):84.
- Reaneyand SH and Smith DR, Manganese oxidation state mediates toxicity in PC12 cells. Toxicol Appl Pharmacol. 2005;205:271–281.
- Chaberek S, Martell AE. Stability of metal chelates. I. Iminodiacetic and iminodipropionic acids. J Am Chem Soc. 1952;74:5052.
- Chaberek S, Martell AE. Interaction of divalent metal ions with N-hydroxyethylethylenediaminetriacetic acid. J Am Chem Soc. 1955;77:1477.
- Ram N, Dey AK. Metal-ligand equilibria in solution: a generalized approach. Indian J Chem A. 1976;14(A):892.
- Sayce IG. Computer calculation of equilibrium constants of species present in mixtures of metal ions and complexing agents. Talanta. 1968;15:1397.
- Sayce IG. Computer calculation of equilibrium constants by use of the program SCOGS; a correction. Talanta. 1971;18:653.
- Sayce IG, Sharma VS. Computer calculation of equilibrium constants using programme SCOGS: a further modification. Talanta. 1972;19:831.
- Carey GH, Martell AE. Mixed ligand Chelates of uranium (IV)1,2. J Am Chem Soc. 1967;89:9895.
- Boggess RK, Martin RB. Copper(II) chelation by dopa, epinephrine, and other catechols. J Am Chem Soc. 1975;97:3076–3081.
- Bhattacharya PK, Vadapadapatri M. Interligand interaction in mixed amino acid complexes. Dalton Trans. 1989;4:567–568.
- Stephan DS, Dominik H, Thomas M, et al. Stabilization of the computation of stability constants and species distributions from titration curves. Computation. 2021;9:55.