Abstract
APAP (N-acetyl-p-aminophenol) overdose induced cellular damage has been linked to oxidative assault by free radicals. Otherwise, constant emergence of resistant virus strains worldwide calls for an urgent need to develop therapeutic agents to improve the treatment of virus infection especially vs Herpes Simplex Virus-type 1 (HSV-1) which is a widespread/ repeated virus with no medical cure. Acrocarpus is a genus in family Fabaceae which is considered as a large economical & medicinal family. Here, we investigated the antioxidant activity of aqueous methanol extract of A. fraxinifolius leaves (AMEAFL) in vitro & in vivo vs APAP-induced organ toxicity & their antiviral/virucidal activity vs HSV 1 in vitro. In vivo, AMEAFL reduced serum cellular toxicity & cellular lipid peroxidation. It enhanced cellular antioxidants in a dose-dependent manner. In vitro, its total phenolic reached 392.4 mg/100 g with 85.3% of its antioxidant activity. It gave strong radical scavenging abilities (DPPH, 90.72%; •NO: 68.16% & O2•−, 79.84%). It was non-toxic to VERO cells & the inhibitory activity to HSV1 was dose dependent. Their MIC, EC50 & SI were 72.6, 51.9, & 2.09 µg/ml. Direct contact between the AMEAFL & virus resulted in varying levels of virucidal action relying on its polyphenolic content. AFL has a dual effect. It has a prophylactic effect on APAP toxicity by increasing the endogenous antioxidant, as well as anti-HSV-1 activity by limiting virus attachment & viral multiplication at the early stages.
1. Introduction
Nonsteroidal anti-inflammatory drugs (NSAIDs) are routinely recommended drugs for management of pain and fever, but they have been limited in clinical situation due to serious hepatic, renal and gastrointestinal problems [Citation1,Citation2]. They belong to a class of non-selective and selective cyclooxygenase (COX) inhibitors. The proportional inhibitory effects of NSAIDs on COX-1 and COX-2 differ [Citation3]. Selective COX-2 inhibitors, which inhibits prostaglandin synthesis, provide some advantages in terms of reducing such side effects, but the risk of cardiovascular adverse events must be considered [Citation4].
N-acetyl-p-aminophenol (APAP), generally referred to as acetaminophen/paracetamol, is a non-prescription analgesic and antipyretic medication that is deemed safe and effective at therapeutic levels over the world [Citation2]. One of the most common causes of poisoning is APAP toxicity. It interacts with a variety of enzyme families, including cytochrome P450, COX, and nitric oxide synthase (NOS), all of which are known to contribute to oxidative stress by generating superoxide radical anions (O2•−), for example. The pathophysiology of APAP-induced tissue injury is complicated by oxidative stress. In APAP toxicity, oxidative stress is caused by a significant decrease in the intracellular amount of GSH and other enzymatic antioxidants [Citation2]. GSH has a crucial role for counteracting free radical mediated cellular damage and is important as an electron donor for certain endogenous antioxidative enzymes such as GPx. GR is a cytosolic enzyme involved in the replenishment of GSH reserves via reducing the oxidized glutathione form (GSSG). Also, Free radical scavenging enzymes such as GSH-Px and CAT represent the first line of cellular defense against oxidative damage.
Excessive usage or overdose of APAP has been linked to organ damage (particularly the liver, which represents the site of toxic metabolite synthesis, and the kidney, which represents the site of toxic metabolite clearance) [Citation2–6].
The activity of APAP’s reactive metabolite (N-acetyl-p-benzoquinoneimine, NAPQI), which is produced in the liver by cytochrome P450, is responsible for its toxicity. The antioxidant actions of intracellular GSH detoxifies NAPQI. As a result, an excessive amount of APAP causes cellular GSH to be depleted [Citation2]. Therefore, there was a decrease in GSH and an increase in NAPQI, which mediates oxidative damage and consequently increases cellular injuries and organs failure [Citation6–8]. We hypothesized that administration of the antioxidants herbal drugs following stress induction by APAP would reduce organs oxidant stress. Antioxidants are defined as any chemicals that considerably slow or prevent the oxidation of an oxidizable substrate when present at low concentrations. As a result, SOD catalyzed the dismutation of superoxide radicals into molecular O2 and H2O2, CAT reduced H2O2, and GR catalyzed NADPH-dependent GSH regeneration from the oxidized form (GSSG) produced by GPX [Citation6–8].
APAP causes acute and/or chronic liver/kidney damage, cardiovascular, gastrointestinal problems, and splenic hyperplasia, according to several studies [Citation1,Citation2,Citation8–13]. Seventy-five percent of blood entering the liver comes directly from the gastrointestinal tract, and portal veins transport these medications as foreign compounds in near-undiluted form to the spleen. After tissue injury, splenic monocytes travel through the bloodstream and migrate to inflammatory areas. Then, these monocytes mature into macrophages, which play a role in inflammatory responses [Citation12]. In dogs, APAP causes liver damage, renal failure, and substantial hematologic abnormalities such as Heinz bodies development and hemoglobin degradation [Citation10,Citation14]. Herpes simplex virus type 1 (HSV-1), on the other hand is a member of the human Herpesviridae family. HSV-1 consists of a large double stranded DNA genome encased within an icosahedral protein cage called the capsid, which is wrapped in the virus envelope. HSV-1 contains about 74 genes within its genome. Fever blisters are caused by the HSV-1 DNA virus. Flu-like symptoms including fever, scratching, or itching, and painful papules are the most common symptoms of HSV-1 infection [Citation15]. The problem with this virus isn’t the painful papules; it’s the way it stays dormant in nerve cells, allowing for recurring infection. Radiation, as well as other linked factors as sunshine, menstruation, and irradiation treatment, have been shown to induce recurrent infection [Citation16,Citation17]. Herpes is difficult to treat since there is no cure; once infected, the HSV remains in the body for the rest of one’s life. The virus remains dormant in neurons until something causes it to reactivate. Treatments, on the other hand, can help to alleviate the symptoms and reduce the pain and the incidence of re-infections. Antiviral medications with no adverse effects produced from medicinal plants, as well as new efficient compounds with varied mechanisms of action vs viral infections, are urgently needed.
Pink cedar, (Acrocarpus fraxinifolius) leaves (AFL), belonging to the Fabaceae family, is a widely distributed tree native to Africa and Asia. It can also be found in tropical areas such as Egypt. Also, Mundani, or Shingle tree are some of its common names [Citation2,Citation18]. Antioxidant, anti–diabetic, anti–inflammatory, anti–proliferative, and hepatoprotective properties of the plant extracts have been observed in experimental animals [Citation2,Citation19–23], as well as anticancer activity in vitro [Citation18]. Antioxidant, cardioprotective, liver/kidney protective, anti–inflammatory, antifungal, antibacterial, analgesic, wound–healing, anxiolytic, anti–arthritic, antiviral, and immune–modulatory are only a few of the biological actions of the Fabaceae family [Citation2,Citation18–21].
The main relevance of phenolic compounds lies in their antioxidant capacity, which enables them to scavenge active oxygen species, block nitrosation and prevent the chelate metal ions, have the tendency for autoxidation, as well as alter the activities of specific cellular enzymes [Citation24]. Antioxidant activity has been demonstrated in AFL extract [Citation19]. The antioxidant effects of phenolic compounds in AMEAFL, as well as their relationship to antiviral efficacy against HSV-1, are little understood.
Furthermore, there is limited evidence that AMEAFL protects against oxidative stress caused by APAP in organs such the heart, the kidney, and the spleen tissues. Thus, the purpose of our study is to examine the antioxidant potential activity of AMEAFL vs APAP-induced tissue damage in rats, as well as to investigate the anti-HSV-1 of AMEAFL in vitro.
2. Materials and methods
2.1. Chemicals
Biochemical and antioxidants kits were measured using a commercially available kit (Bio Diagnostic, Giza, Egypt). N-acetyl-p-aminophenol (APAP) was purchased (Sanofi-Aventis, Cairo, Egypt).
2.2. Plant collection and preparation
AFL was collected in March 2016 at the El-Orman botanical garden (Giza, Egypt). Mrs. Treize Labib, a taxonomy specialist at the same botanical facility, confirmed the AFL. The Pharmacognosy Department (Faculty of Pharmacy, Ain Shams University) received voucher specimens (PHG/P/AF130). The previous procedure was used to make the AFL [Citation18]. The AFL were carefully cleaned with tap water to eliminate dirt & soil. The AFL were then dried in the shade at room temperature and powdered with a mortar & pestle. About two kilograms of AFL was soaking in 80% aqueous methanol for five days then filtered using Whatman grade1 filter paper. The filtrate was thoroughly lyophilized under vacuum at ≈55°C for 48 h till totally dryness to give 522 grams of powder extract.
2.2.1. In vivo analysis
2.2.1.1. Animals
In the present study, Adult male Rattus norvegicus (Wistar albino rats) weighed 180–200 gm and were 7–8 weeks old. In suitable animal room at Zoology Department (Faculty of Science, Ain Shams University (ASU), Egypt), the rats were kept in appropriate cages and fed pellet diet and tap water on an ad libitum basis. One week before to the start of the research, the rats were given an acclimatization period. The present study was approved by the ASU Research Ethics Committee and gave a permission to carry it out, and all the experiments were carried out according to the National Institutes of Health protocol for the care and use of Laboratory animals (NIH Publications No. 8023, revised 1985).
2.2.1.2. Experimental animals and design
The experimental animals were randomly split into 6 groups (n = 6 per group). Rats in Group I (healthy control group) served as the control group; rats in Groups II and III (AMEAFL 250 and 500, respectively) received just AMEAFL (250 and 500 mg/kg b.w., p.o) for twenty-one days. The dose of AMEAFL chosen was based on previous research [Citation2,Citation23]. Rats in Group IV (APAP+ vehicle) were given APAP (750 mg/kg b.w., p.o.) to generate cellular oxidative stress in the final seven days [Citation8]. Rats in Groups V and VI (AMEAFL 250 + APAP and AMEAFL 500 + APAP, respectively) received orally AMEAFL at dose 250 or 500 mg/kg b.wt, p.o, for twenty-one days before being treated with APAP for the final seven days as Group IV.
2.2.1.3. Collection of blood and tissues samples
Animals were euthanized after an overnight fast following the last administration dose. The blood was drawn into tubes containing EDTA as anticoagulant or EDTA-free. The heart, kidney, liver, and spleen tissues were removed then washed and weighed before being homogenized (5 mL cold buffer/g tissue) then centrifuged (15 min., 4000 rpm, 4°C). The supernatant was separated into aliquots and kept at −80○C till used for determining the antioxidant markers.
2.2.1.4. Estimations of the body & organs weights and their relative weights
The body weight as well as the organs weights including heart, kidney, liver, and spleen tissues were determined by using digital balance. Organ relative weight was computed as follows: [(organ weight in gram per body weight in gram) × 100].
2.2.1.5. Estimations of hematological parameters
Hematological parameters including red blood corpuscles, hemoglobin, hematocrit, white blood cells (RBCs, Hb, HCT, & WBC, respectively), differential granulocytes/agranulocytes counts were measured by automated hematology analyzer (Coulter). Also, blood indices including mean corpuscular volume, mean corpuscular hemoglobin and mean corpuscular hemoglobin concentration (MCV, MCH & MCHC, respectively) were computed using the following equations: MCV (fL/RBC) = [(HCT × 10) /RBCs], MCH (pg/RBC) = [(Hb × 10)/RBCs], and MCHC (gm/dL) = [(Hb × 100)/HCT].
2.2.1.6. Estimations of serum toxicity markers
The activity of the alanine aminotransferase (ALT) enzyme was used to assess hepatotoxicity and nephrotoxicity. The activity of the enzyme aspartate aminotransferase (AST) was also assessed as a marker for more general toxic effects on several organs. In a brief, they were calculated using Reitman & Frankel’s methodology [Citation25], which involved evaluating the quantity of pyruvate or oxalo-acetate produced by the synthesis of 2, 4-di-nitro-phenyl-hydrazine. Lactate dehydrogenase (LDH) was performed as an index of inflammation and cellular toxicity in many different tissues. Serum urea was quantified using a urease method [Citation26], uric acid was assessed using a uricase method [Citation27], and creatinine was assessed using a method previously described [Citation28]. In an alkaline media, creatinine was generated into a colourful complex with picrate.
2.2.1.7. Estimations of nitric oxide and lipid peroxidation (LPO) status
Serum nitric oxide (NO) is formed by the nitric oxide synthase (NOS) which acts on oxygen, arginine, and NADPH to produce NO, citrulline, and NADP+. In brief, the azo dye that results have a strong reddish-purple colour that may be seen at 540 nm. Nitrite and nitrate are the end products of NO in vivo. In serum, heart, kidney, and spleen tissues, thiobarbituric acid reactive substance (as marker for LPO; TBARS) was determined [Citation29]. In an essence, LPO is calculated by forming TBARS by reacting thiobarbituric acid with malondialdehyde in an acidic media at ninety-five °C for thirty minutes in a boiling W.B. The pink product’s absorbance (TBARS) was measured at 534 nm and computed: TBARS (nmol/gram tissue) = [(ASample/Astandard) × (10/gram tissue used)].
2.2.1.8. Estimations of tissues non-enzymatic antioxidant status
In heart, kidney, and spleen tissues, the reduced glutathione (GSH) level was assayed according to previous study [Citation30]. In a nutshell, the method is based on the production of a yellow colour when 5, 5-dithiobtis-2 nitrobenzoic acid (DTNB) is introduced to sulfhydryl-containing molecules (GSH). At 405 nm, the lowered chromogen’s absorbance was assayed. It was computed. GSH level (mg/gram tissue) = [(ASample × 66.66) per gram tissue].
2.2.1.9. Estimations of tissues enzymatic antioxidant status
In Heart, kidney, and spleen tissues, the activity of glutathione peroxidase (GPx) was determined using previous study [Citation31]. The assay measures the activity of GPx in an indirect manner. GR recycles the GSSG generated by the reduction of organic peroxide by GPx to its reduced state. At 340 nm, the absorbance decreases, due to NADPH is converted to NADP. It was computed as follows: [(A340 nm/min/0.00622) × (25/gram tissue)]. The activity of glutathione reductase (GR) was measured [Citation32]. In a nutshell, in the presence of NADPH, GR catalyzes the reduction of GSSG to the sulfhydryl form GSH. When NADPH is converted to NADP+, the absorbance decreases. Finally, at 340 nm, the decrease in absorbance was observed. It was computed as follows: [(4019 × A340 nm/min) × (1/gram tissue)]. The activity of superoxide dismutase (SOD) was measured [Citation33]. This assay is based on the enzyme’s capacity to prevent the nitro blue tetrazolium dye from being reduced by phenazine methosulphate (NBT). At 560 nm, the reaction’s produced blue colour was detected. It was computed as follows: [% inhibition × 3.75 × (1/gram tissue)]. The activity of catalase (CAT) was assayed [Citation34]. CAT combines with a known quantity of hydrogen peroxide (H2O2) and the reaction is stopped after one minute with CAT inhibitor. In the presence of peroxidase, the residual H2O2 combines with 3,5- dichloro-2- hydroxybenzene sulfonic acid and 4- aminophenazone to form a chromophore with a colour intensity that is inversely proportional to the amount of CAT in the sample. At 510 nm, the absorbance was measured. It was computed as follows: [(Astandard − ASample/Astandard) × (0.5) × (1000/gram tissue)].
2.2.2. Phytochemical analysis
2.2.2.1. Estimations of phytochemical screening and total phenolic content
The phytochemical screening was determined by qualitative method (presence/absence) to identify the secondary metabolites (as flavonoids, tannins, glycoside, saponins, terpenoids, phenolics, alkaloids) using standard protocol [Citation35]. Using the Folin–Ciocalteu (FC) method, the total phenolic content was assayed [Citation36]. This test is based on the oxidation of phenolic groups by phosphomolybdic and phosphotungstic acids. FC reagent is used to perform colorimetric oxidation and reduction of phenolic compounds based on previous method [Citation36]. The reaction’s produced blue colour (metal oxide reduction) was detected at 764 nm. The concentration of phenols is proportional to the strength of light absorption at that wavelength. Briefly, a twenty µL of the AMEAFL was mixed to one hundred µL of FC-reagent. Then, add 300 litres of Na carbonate (25%) to the mixture after 8 min. After that, the absorbance was estimated. By using the gallic-acid (GA) solutions, the calibration curve was created. GA solutions were ranging from 0 to 500 mg/L. The results are expressed in GA equivalents (GAE).
2.2.2.2. Estimations of antioxidant action
The activity of antioxidant was measured [Citation37] based on the of combining the AMEAFL with linoleic acid. In 20 ml chloroform, 6 mg of AMEAFL was dissolved. Then, forty µl linoleic acid and 400µl Tween 20 were mixed with three ml of the solution. To remove the chloroform, the rotary evaporator was utilized (vacuum at 35°C). The AMEAFL emulsion was blended thoroughly with oxygenated distilled water (100 ml). In a test tube, a 3 ml aliquot of the AMEAFL emulsion was mixed with the diluted AMEAFL (0.2 ml). The tubes were put in a W.B. and kept at 50°C right away. At 470 nm, oxidation of AMEAFL emulsion was monitored. After incubation, the AMEAFL absorbance was measured at 10, 20, 30, 40, 50, and 60 min. Instead of the AMEAFL, 0.2 mL distilled water was used as a control. The AMEAFL degradation rate was determined as: Sample degradation rate = [ln (a/b) × (1/t)].
Where: ln, natural log; a, initial absorbance at time 0; b, absorbance at 10, 20, 30, 40, 50 and 60 min; t, time (min). The following equation was used to calculate antioxidant activity (AA) as a percentage inhibition compared to the control: AA (percent) = [(Control Degradation − Sample Degradation) × (100/Control Degradation)].
2.2.2.3. Estimations of free radicals scavenging activity by DPPH, nitric oxide (•NO), and superoxide anion (O2•−) assays
Various concentrations of AMEAFL (20, 40, 60, 80 & 100 µg/ml) were used in vitro free radicals scavenging measurement. The DPPH (1,1– diphenyl– 2–picryl hydrazyl) assay was used to measure the free radical scavenging activity of the AMEAFL according to previous study [Citation25]. Superoxide anion (O2•−) scavenging activity of AMEAFL in phenazine methosulfate (PMS)- nicotinamide adenine dinucleotide (NADH) system was carried out as described in the previous work [Citation26]. Nitric oxide (•NO) scavenging activity of AMEAFL was analyzed [Citation27]. Vitamin C and quercetin were used as the reference standard for standard antioxidant assays like DPPH and O2•−as well as •NO radicals scavenging assays.
2.2.3. In vitro analysis
2.2.3.1. Cells and viruses
HSV 1 (Herpes simplex virus type 1, CATCC-733), African green monkey kidney cells (VERO cells, ATCCCCL-81) were obtained from American type culture collection. African Green Monkey kidney cells (VERO) were cultured in minimal essential medium supplemented with ten percent fetal bovine serum, penicillin, streptomycin, and amphotericin B (100 U/ml, 100 g/ml, & 25 g/ml, respectively) for anti HSV-1 activity screening. The VERO cells were kept at room temperature (37°C) in a humidified five percent CO2 environment. In VERO cells, HSV-1 was propagated. The stock viruses were manufactured as previously described [Citation38]. The fluids were titrated depending on a plaque-forming unit (PFU) count after three cycles of freezing/thawing and stored at −80°C until usage as previously stated. The cytopathic impact in cell culture was used to measure the virus titre indicated by (TCID50%; half percent tissue culture infective dose).
2.2.3.2. Method for cytotoxicity assay
The cytotoxicity of AMEAFL was determined using MTT [3-(4, 5-dimethylthiazol-2-yl)-2,5-diphenyltetrazolium bromide]. This approach is based on the action of mitochondrial succinate dehydrogenase, which converts MTT’s yellow dye to Formosan’s violet dye. To make a stock solution with a concentration of 400 g/ml, 400 mg of the AMEAFL was diluted in DMSO (dimethyl sulfoxide, 200 ml) and 800 ml of PBS (phosphate buffer solution). In minimal essential medium (MEM), two-fold dilutions were made with 2 percent fetal calf serum, sodium bicarbonate, and penicillin/streptomycin/amphotericin b. Confluent VERO cell monolayers were covered with various dilutions of the AMEAFL (3.125, 6.25, 12.5, 25, 50, 100 µg/ml) and cultured for five days at room temperature (37°C) with five percent CO2. The toxicity of the AMEAFL was assessed by comparing the morphology of the cells to a control cell without the AMEAFL. For cell viability confirmation, neutral crystal violet dye of Formosan was introduced after five days of incubation. Microscopically, the cell monolayer was investigated for changes in cell shape or obvious toxic effects [Citation39]. The cells that were cultivated without AMEAFL were used to represent 100% cell survival (incubated with DMSO as negative control). Cell viability (%) was calculated as follows: [Abstreated/AbsControl × 100]. The AMEAFL concentration produced, which lowered cell viability to half, was identified as half maximal inhibitory concentration (IC50).
2.2.3.3. Cytopathic effect reduction assay
The antiviral activity of AMEAFL vs HSV-1 was assessed by using TCID50. The TCID50 assay is a cytopathic effect (CPE)-dependent assay that looks at the integrity of VERO cells monolayers as a measure of viral load. CPE is induced by reproducing viruses, and its suppression indicates antiviral action. VERO cells were cultured in DMEM supplemented with 10% FBS and 1% penicillin/streptomycin and incubated (37°C & 5% CO2). Cells were detached with 2 percent trypsin when they reached 85 percent confluency, and cell counts were performed using an automated cell counter. VERO cells were infected with the HSV-1 strains (0.1 mL/well viral solution). Infected cells were treated with non-cytotoxic doses of the AMEAFL after viral adsorption. Inverted light microscopy was used to look for cellular changes/cytopathic effect (CPE) on VERO cells after 72 h. The reduction of virus multiplication was computed as follows: (CPEtest value/CPEvirus control × 100). As a result, fewer CPEs were used to determine if the extracts were antiviral. As controls, the experiment contained only the virus and cells (positive control) and only the medium and cells (negative control).
2.2.3.4. Method for viral plaque reduction assay
A plaque reduction assay was utilized using monolayer cultures of VERO cells to determine the inhibitory effect (antiherpetic action) of the AMEAFL (at different concentrations: 3.125, 6.25, 12.5, 25, 50, 100 µg/ml) on HSV-1 replication in vitro. It is a method for determining the effect of chemicals on PFU in contrast to a control group. In a twenty-four well plate (5 days; 5 percent CO2; 37°C), monolayers of VERO cells were infected with 100–200 PFU/well with HSV-1 in the absence or presence of the extract (treated). The viral inoculum was removed after 45 min of incubation at 37°C, and the cell monolayers were rinsed with phosphate buffer saline (PBS) and overlaid with plaque medium (PM) supplemented with the extract. In the Control wells, PM was added without the AMEAFL. Cell monolayers were fixed and stained with 0.1% crystal violet, in 1% ethanol, for 15 min, and viral plaques were counted. Viral inhibition (%) after treatment with the AMEAFL was calculated as percentage inhibition compared with untreated viral infected cells control from triplicate experiments. The EC50 values were derived using regression analysis of the data-generated dose–response curves. The CC50/EC50 ratio was used to determine the selectivity index (SI).
2.2.3.5. Method for virucidal action assays
It was tested by inoculating the virus directly into cells after incubating it with AMEAFL. To summarize, six wells were cultured with VERO, and fifty µl of virus diluted to one hundred- two hundred PFU/ml was mixed to AMEAFL dilutions (V/V) that gave the max. viral inhibition, resulting in a total volume of one hundred µl. After 1-hour incubation at 37°C with shaking. The mixture was diluted by ten-fold to obtain a count of virus particles that was acceptable. Then one hundred µl of the mixture was applied to the cell sheet and kept for 1-hour at room temperature (37°C) with shaking, followed by 3 ml of overlay media. The plates were allowed to harden before being incubated at 37°C and monitored daily until the viral plaques appeared. For 2 h, cell sheets were preserved in ten percent formalin and dyed with crystal violet.
As controls, the experiment contained only the virus and cells without the addition of the AMEAFL. The number of viral plaques were counted to determine the number of infectious viruses that remained. Viral inhibition % was calculated [Citation40].
2.2.3.6. Method for antiviral assay
Evaluation of the AMEAFL was performed in 3 distinct stages as follows: Before, during, and after virus inoculation.
2.2.3.4.1. Method for measuring the effect of AMEAFL on pretreated cells
The AMEAFL was examined by exposing it to a monolayer sheet of VERO cells for 2-hours prior to virus inoculation. Briefly, VERO cells were grown in a six-well, and non-cytotoxic different dilutions of AMEAFL were applied to the sheet of VERO cells at conc. that resulted in a high % of HSV-1 suppression, then incubated for 2 h at 37°C. Three times of washing the cells with medium devoid of additives, the AMEAFL was eliminated. After diluting the virus to one hundred- two hundred PFU/ml, fifty µl of the HSV-1 stock was spread to the sheet of VERO cells as well as kept for 1-hour at 37°C with shaking for HSV-1 adsorption. The plates were allowed to harden before being incubated at 37°C and monitored daily until the viral plaques appeared. For 2 h, cell sheets were preserved in ten percent formalin before being dyed with crystal violet. As a control, HSV-1 inoculums were inoculated just to cells only. The number of viral plaques were counted, as well as the viral inhibition % was calculated [Citation41].
2.2.3.4.2. Method for measuring the effect of AMEAFL on HSV-1 during viral adsorption
Confluent cell monolayers of VERO cells, seeded in twenty-four well, were infected with one hundred- two hundred PFU of the virus. Several non-toxic conc. of the AMEAFL were applied to the monolayers of VERO cells and incubated for 1-hour at 37°C during HSV-1 adsorption. The inoculum was then removed, and infected cells were covered with overlay media and cultured for 5 days at room temperature (37°C) in five percent CO2. For 15 min, the virus plaques were dyed (0.1 percent crystal violet in one percent ethanol). The half max. effective concentration (EC50) was estimated.
2.2.3.4.3. Method for measuring the effect of AMEAFL on virus replication
It was examined by post inoculating VERO cells with AMEAFL after exposing them to HSV-1. VERO cells were grown in a six-well and cultured at 37°C for 1–2 days to create a confluent sheet. After diluting the virus to one hundred- two hundred PFU/ml, fifty µl of the HSV-1 stock was administered to the monolayer of VERO cells and kept for 1-hour with shaking at 37°C. Three times of washing the cells with media without additives, unabsorbed virus particles were eliminated. After virus adsorption, noncytotoxic doses with high anti- HSV-1 efficacy were provided at 1, 4, 8, 24, and 48 h. Three ml of overlay media was applied to the cell monolayer sheet after each time interval. The wells were allowed to solidify before being kept at room temperature (37°C) and monitored daily till the viral plaques appeared. For 2 h, cell sheets were preserved in ten percent of formalin before being dyed with crystal violet. As a control, HSV-1 inoculums were injected just to cells only. The number of viral plaques were counted, as well as the viral inhibition % was calculated [Citation42].
2.3. Data management and analysis
The data were presented as mean values ± standard error of mean (SEM). The results were analyzed using one-way ANOVA, and the differences between groups were assessed by Tukey’s multiple comparison test. P values of less than 0.05 were declared statistically significant. Graph Pad Prism software was used to analyze the data.
3. Results
3.1. In vivo finding
3.1.1. The influence of AMEAFL on body weight, and organs relative weight of rats
The body weight gain and liver/kidney weights & their relatives of the intoxicated rats that received APAP + vehicle significantly decreased (P < 0.05) and increased (P < 0.001), respectively, compared to the control rats (Table ). On the other hand, the intoxicated rats that received either APAP + vehicle or AMEAFL + APAP did not show any changes on the spleen/heart weights and their relatives in this study. The low dose of AMEAFL did not show any modulation on the decrease in the body weight and the increase in liver/kidney weights & their relatives (P < 0.05–P < 0.001 compared to the control rats/P > 0.05 compared with the APAP + vehicle rats) of an intoxicated rat. Otherwise, high dose of AMEAFL completely modulated the loss in body weight (P > 0.05 compared to the control rats/P < 0.001 compared with the APAP + vehicle rats), and partially alleviated the increase in liver/kidney weights & their relatives (P < 0.05 compared to the control rats/P < 0.001 compared to the APAP + vehicle rats).
Table 1. The modulatory effects of AMEAFL on the changes in the body weight and organs weights & their relatives of the intoxicated rats.
3.1.2. The influence of AMEAFL on hematological parameters in rats
The RBC count, Hb content, HCT value were significantly decreased (P < 0.05), but MCV, MCH, MCHC, WBCs, neutrophil, eosinophil, monocyte, and lymphocyte counts were insignificantly affected (P > 0.05) compared with the control rats (Table ). The oral administration of both doses of AMEAFL reversed these changes (P > 0.05) in RBC, Hb, HCT values compared to the control rats (Table ).
Table 2. The modulatory effects of AMEAFL on the changes in the hematological parameters of the intoxicated rats.
3.1.3. The influence of AMEAFL on serum hepatic and renal markers in rats
The AST, ALT, LDH, total cholesterol, total bilirubin, creatinine, uric acid, and urea of the intoxicated rats that received APAP + vehicle significantly increased (P < 0.05–0.001), but total protein of the intoxicated rats that received APAP + vehicle significantly decreased (P < 0.001) compared to the control rats (Table ). The low dose of AMEAFL partially alleviated the increase in the AST, ALT and LDH (P < 0.001 compared to the control rats/P < 0.05 compared to the APAP + vehicle rats) and did not show any modulation on the increase in the total cholesterol, total bilirubin, creatinine, uric acid and urea as well as the decrease in the total protein (P < 0.05 – P < 0.001 compared to the control rats/P > 0.05 compared to the APAP + vehicle rats) of intoxicated rats. Oral administration of the high dose of AMEAFL completely modulated the increase in the AST, ALT, LDH, creatinine, uric acid, and urea (P > 0.05 compared to the control rats/P < 0.001 compared to the APAP + vehicle rats) and partially alleviated the increase in total cholesterol, and total bilirubin as well as the decrease in the total protein (P < 0.05 compared to the control rats/P < 0.001 compared to the APAP + vehicle rats) of intoxicated rats.
Table 3. The modulatory effects of AMEAFL on the changes in the serum toxicity markers of the intoxicated rats.
3.1.4. The influence of AMEAFL on cellular oxidant and antioxidant markers in rats
The TBARs concentration of the intoxicated rats that received APAP + vehicle significantly increased (P < 0.05–0.001) in heart, kidney, and spleen tissues compared to the control rats. On the other hand, GSH level, GPx, GR, SOD, and CAT activities of the intoxicated rats that received APAP + vehicle significantly decreased (P < 0.05–0.001) in the heart, kidney, and spleen tissues compared to the control rats (Figure ).The low dose of AMEAFL partially alleviated the increase in the TBARs concentration (P < 0.001 compared to the control rats/P < 0.05 compared to the APAP + vehicle rats) in kidney, and spleen tissues and completely modulated the increase in the TBARs concentration in heart tissues (P > 0.05 compared to the control rats/P > 0.05 compared to the APAP + vehicle rats). Otherwise, the low dose of AMEAFL partially alleviated the decrease in the GSH level, GPx, GR, SOD, and CAT activities (P < 0.001 compared to the control rats/P < 0.05 compared to the APAP + vehicle rats) in heart, kidney, and spleen tissues, except GPx, GR, SOD, and CAT activities in heart tissues were reverted to near normal concentrations upon oral treatment with low dose of AMEAFL (P > 0.05 compared to the control rats). Oral administration of the high dose of AMEAFL partially alleviated the increase in the TBARs concentration and the decrease in the GSH level, GPx, GR, SOD, and CAT activities (P < 0.05 compared to the control rats/P < 0.001 compared with the APAP + vehicle rats) in the heart, kidney, and spleen tissues, except GPx, GR, SOD, and CAT activities in heart tissues were reverted to near normal concentrations upon oral treatment with high dose of AMEAFL (P > 0.05 compared to the control rats), but the utmost modulation on the changes in SOD activity was shown in the intoxicated rats that received high dose of AMEAFL (P > 0.05 compared to the control rats/P < 0.05 compared to the APAP + vehicle rats).
Figure 1. The modulatory effects of AMEAFL on the changes in the oxidant and antioxidant biomarkers in the kidney, spleen, and liver tissues of the intoxicated rats. (a) TBARS: thiobarbituric acid reactive substance; (b) GSH: Reduced glutathione; (c) GPx: Glutathioneperoxidase; (d) GR: Glutathione reductase; (e) SOD: Superoxide dismutase; (f) CAT: Catalase.
Notes: Values are means ± SEM. AMEAFL: aqueous methanolic extract of A. fraxinifolius leaves; and N-acetyl-p-aminophenol. *symbol = statistically significant compared to the control group; †symbol = statistically significant compared with the APAP-group.
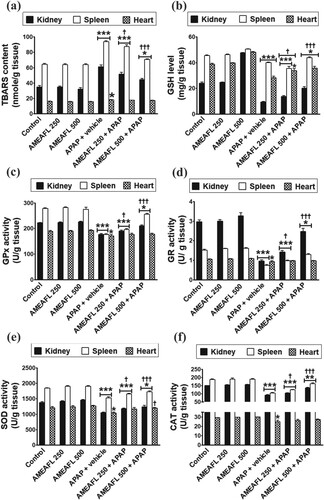
3.1.5. The influence of AMEAFL on healthy normal rats
In healthy-treated rats who received both doses of AMEAFL, none of the following parameters examined in this study were significantly affected (p > 0.05) compared with the control rats (Tables and Figure ).
Furthermore, during the whole research, the death rates (mortality) in all groups that received both doses of AMEAFL were nil. Following that, no negative effects were found for the dose of AMEAFL employed in this investigation.
3.2. Phytochemical finding
3.2.1. Phytochemical screening, total polyphenolic content, antioxidant, and free radicals scavenging activities of AMEAFL
Phytochemical analysis revealed the presence of flavonoids, phenols, glycosides, steroids, tannins, and alkaloids. On the other hand, it gave negative results to oil, phlobatannins, terpenoids, fatty acids, and saponins (Table ). The AMEAFL has a total polyphenol concentration of 392.4 mg/100 g, with 85.3 percent antioxidant activity, according to our findings. Figure shows the dose–response results of DPPH, •NO and O2•−scavenging of AMEAFL. The DPPH radical (90.72%) scavenging abilities of AMEAFL at 100 µg/ml were significantly decreased (p < 0.01) compared with those of positive control (vitamin C; 96.49%). The •NO (68.16%) and O2•− (79.84%) radicals scavenging abilities of AMEAFL at 100 µg/ml were significantly decreased (p < 0.001–0.01) compared with those of quercetin (86.48% and 94.07, respectively).
Figure 2. Free radical scavenging (a), nitric oxide (b), superoxide radicals scavenging (c) potentials effect of AMEAFL In vitro.
Notes: Values are means ± SEM. AMEAFL: aqueous methanolic extract of A. fraxinifolius leaves, Vit. C: vitamin C.
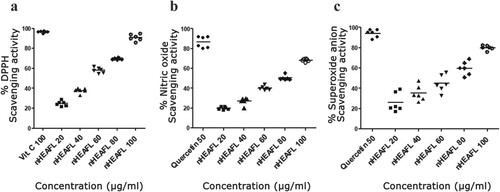
Table 4. Phytochemical analysis of AMEAFL.
3.3. In vitro finding
3.3.1. The influence of AMEAFL on cell viability (cytotoxicity) and cytopathic effect reduction assay
The cytotoxicity effect of the AMEAFL on the proliferation and viability of VERO cells was examined prior to determining their viral inhibitory effect, using the MTT assay. The results showed that AMEAFL exhibited no significant cytotoxicity at the concentrations from 3.125 to 100 µg/ml AMEAFL had a CC50 up to concentration 400 µg/ml. As shown in Table and Figure , the studied concentrations of AMEAFL significantly reduced viral titre in VERO cells by reducing the cytopathic effect assay generated by virus infection. All dilutions of AMEAFL (especially on higher amount) revealed varying levels of the cytopathic action, but the lower amount reported no cytopathic action on the VERO cells (IC50 about 108.9 µg/ml as well as the level of cell-safe about ≤72.6 µg/ml).
Figure 3. Cytopathic effect % of AMEAFL.
Notes: AMEAFL: aqueous methanolic extract of A. fraxinifolius leaves.
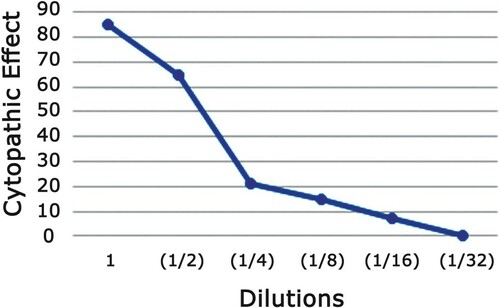
Table 5. Cytopathic effect % of AMEAFL.
3.3.2. The influence of the AMEAFL on viral plaque number reduction assay
Safest concentrations of the extract obtained from cytotoxicity experiment were used in all following experiments. Following experiments were conducted to evaluate the antiviral activity of the extract and to study the AMEAFL effect on life cycle of the virus. EC50 was calculated as well as the SI was determined as the IC50/EC50 and measures the actual effectiveness of the AMEAFL as a therapeutic agent in terms of effective does as a ratio to its cytotoxicity against the normal cells. Results in Table , and Figure show that the AMEAFL started with a moderate anti-HSV-1 character of 68% at dilution 1/4 (25 µg/ml) which is comparable to72.6 µg/ml. At dilution 1/8 (12.5 g/ml), the anti-HSV-1 character practically disappeared, yielding 39.2% anti-HSV-1 activity; the AMEAFL had an EC50 of 51.9 µg/ml, SI of 2.09, and MIC of 72.6 µg/ml.
Figure 4. Plaque reduction assay of each cell-safe dilution of AMEAFL extract.
Notes: AMEAFL: aqueous methanolic extract of A. fraxinifolius leaves.
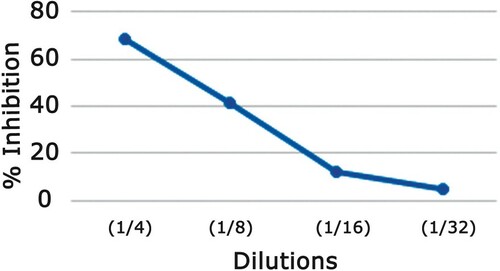
Table 6. Plaque reduction assay of AMEAFL.
3.3.3. The influence of the AMEAFL on virucidal action
After evaluation of the toxic and the safe levels of AMEAFL on VERO cell, minimum inhibitory concentration (MIC) was incubated with the virus in cell free culture medium for 1-hr. The HSV-1 virus was found to be completely inhibited in 87% of the HSV-1 virus.
3.3.5. The influence of the AMEAFL on HSV-1 adsorption (before, during, & after)
Before, during, and after the virus adsorption, the AMEAFL was applied to the VERO cells at various time intervals, to discover the mode of action to its antiviral effect. Before HSV-1 inoculation, AMEAFL was applied to VERO cells for 1 h and then washed away entirely. The extract had a relatively modest level of viral inhibition, around 8.4%, according to the plaque reduction assay. AMEAFL was given at the same time of HSV-1 inoculation. Our finding reported the percent of virus infection inhibition was about 71.2%. After virus inoculation, the effect of AMEAFL on the viral replication was observed at various time-intervals (one, four, eight, twenty-four and forty-eight hrs). Results are shown in Table and Figure .
Figure 5. Antiviral effect of AMEAFL at various time intervals after virus inoculation.
Notes: AMEAFL: aqueous methanolic extract of A. fraxinifolius leaves.
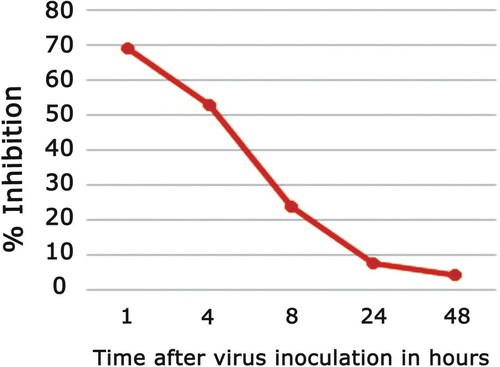
Table 7. Antiviral activity of AMEAFL after the viral inoculation.
4. Discussion
Toxicity testing is paramount in the screening of newly developed drugs before it can be used on humans. The essence of toxicity testing is not just to check how safe a test substance is; but to characterize the possible toxic effects it can produce. The importance of this study is that it examines the APAP-induced organ toxicity in vivo and proposes a natural replacement after carefully investigating its cytotoxic effect, and its prophylactic/therapeutic potential not just as an antioxidant agent but also as an antiviral agent against one of the most resistant viruses that affect humans. In this study, the ameliorative effects of the AMEAFL showed a dose-dependent reduction of serum cellular toxicity (AST, ALT, LDH, total cholesterol, total bilirubin, total protein, creatinine, uric acid, and urea), hematotoxicity, cellular lipid peroxidation (TBARS) and inflammatory markers (NO) in male rats.
LDH is a cytoplasmic enzyme that can be found in almost every tissue. It is a toxicity marker for common injuries and is released into the bloodstream when bodily tissue is injured.
AMEAFL enhanced the synthesis of cellular enzymatic/non-enzymatic antioxidants (GPx, GR, SOD, CAT, and GSH) in kidney, spleen, and heart tissues of rats. Hence it can be used as prophylactic medicinal plant against drug induced toxicity. Many of AFL’s bioactive compounds have been correlated to their antioxidant and radical scavenging activities [Citation2,Citation18,Citation22,Citation23] including flavonoids, phenols, glycosides, steroids, tannins, and alkaloids as was proved in this study. The total phenolic content in the AMEAFL reached 392.4 mg/100 g with 85.3% of antioxidant activity. It displayed strong DPPH radical scavenging abilities (90.72%) as well as •NO (68.16%) & O2•− (79.84%) scavenging abilities compared with vit. C.
Also, AMEAFL was non-toxic to VERO cells up to concentration 400µg/ml and the inhibitory action of HSV1 was dose-dependent on the AMEAFL polyphenol content. The MIC, EC50 and the SI for the AMEAFL were 72.6, 51.9, & 2.09 µg/ml, respectively. Hence, SI showed promising value for the AMEAFL, and therefore it can be used as therapeutic medication for HSV1. Direct contact between the HSV1 and AMEAFL reported various degrees of virucidal action relying on the phytochemical content of AMEAFL.
The discovery of natural substances to activate antioxidant defense system and minimize free radicals’ overproduction has become a new research priority for the treatment of several human diseases. APAP is recently considered the most utilized medicine in self-poisoning, with a high risk of morbidity and fatality. Only when the GSH level is decreased by APAP it is assumed that the hepatic tissues generate a dangerous product. APAP on the other hand converts to NAPQI, which are extremely reactive free radicals that reduce the body’s natural antioxidant GSH and can bind covalently to cellular proteins and unsaturated fatty acids in cell membranes. APAP may also cause organs toxicity by generation of reactive oxygen/nitrogen (ROS/RNS) species such as O2•−, hydrogen peroxide (H2O2) and hydroxyl radical (•OH), nitric oxide (NO) and peroxynitrite (ONOO−) [Citation43]. O2•− plays significant role in the formation of oxidative damage to cellular components. NO toxicity elevates when it reacts with O2• − to form the peroxynitrite anion, which is unstable strong oxidant that decomposed to generate hydroxyl radicals (•OH) and nitrogen dioxide (NO2) [Citation44]. As a result, systemic inflammation can be caused by oxidative stress, which is defined as an imbalance between free radicals and antioxidants. NO generation by the vasculature increases dramatically, contributing to oxidative stress when combined with other ROS.
As revealed in this study, this damage by APAP overdose causes body weight loss, anemia, lipid peroxidation, inflammation, changes in protein function, loss of cell membrane functional integrity, and an increase in cellular injury markers. Sulfhydryl groups are depleted by APAP, which interferes with NO generation, resulting in cellular toxicity [Citation13]. One hypothesis for the direct toxic effect on the heart suggests that myocardial injury occurs due to a similar mechanism which causes hepatic damage, with APAP partly converted to a toxic NAPQI, which acts as a direct toxin on the myocardium [Citation45,Citation46]. APAP caused considerable changes in hematological markers in rats in the current investigation. APAP overdose resulted in a considerable drop in the number of RBC, Hb, and HCT values [Citation45,Citation46]. Lipid peroxidation induced by free radicals were discovered to be the primary causes of cellular RBC destruction [Citation45].
Phenolic compounds are a group of antioxidants which serve as free radical terminators [Citation47,Citation48]. The presence of polyphenolic compounds in AMEAFL may give reliance to its use in the treatment of disorders caused by oxidative stress. Results also showed that when polyphenols content rises, the antioxidant activity rises as well. Donglin and Yasunori [Citation49] reported similar findings.
In comparison to the APAP-only group, oral pretreatment of rats with AMEAFL provided partial protection against overdose APAP-induced tissue damage, as evidenced by significant decreases in serum toxicity markers, serum/cellular lipid peroxidation, and serum NO, as well as significant increases in RBC, Hb, HCT, and cellular antioxidants [Citation22,Citation23]. Furthermore, AMEAFL’s ability to reverse elevated serum cellular toxicity markers in APAP-induced organ injury may be attributable to its membrane-stabilizing effect, which prevents intracellular enzyme leakage [Citation2,Citation22,Citation23]. Furthermore, these modulations alleviated the body weight change [Citation2] and relative liver/kidney weights, especially when AMEAFL was given at a high dose. This could be attributed to the antioxidant and free radical chelator and scavenger activities of phenolic acids in AMEAFL, which have a particular effect on hydroxyl radical (•OH), and peroxyl radicals (ROO•), superoxide anions (O2−), and peroxynitrites (ONOO−) [Citation18]. In APAP-intoxicated rats, AFL has been demonstrated to have an important role in boosting GSH status and total antioxidant capacity [Citation2,Citation23].
AFL extracts contain bioactive phenolics as gallic acid, ellagic acid, brevifolin carboxylic acid, and methyl-gallate [Citation18,Citation20], which may be responsible for its radical-scavenging activity [Citation50].
In addition, the presence of flavonoids, terpinols, and alpha tocopherol (Vit. E), which has a substantial antioxidant effect in the detoxification of free radicals, stabilizing the cell membrane, and restoring structure, may explain the higher preventive efficacy in AMEAFL [Citation2]. Alpha tocopherol may have blocked or scavenged APAP–generated free radical chain reactions before they reached their targets organs. In addition, alpha–tocopherol increased the amount of cytochrome-P3, which converts APAP to NAPQI [Citation2,Citation51].
According to El-Nashar et al. [Citation18], A. fraxinifolius leaves contained high amount of gallic acid (phenolic acids) that may be a reason for ameliorating tissue injuries induced by oxidative stress in rat model of gamma irradiation and CCl4 at dose 500 mg/kg. Also, Abd El-Ghffar & Shehata [Citation22] reported that 500 mg/kg, po, of AMEAFL has great antioxidant and anti-inflammatory activities on liver and kidney dysfunctions of diabetic rat model.
Because of their numerous phenolic hydroxyl groups, anthocyanins, flavonoids, and polyphenols are known to have antioxidant or free radical scavenging effects [Citation52]. The phenolic characteristic of polyphenolic extract can also donate electrons to H2O2 and hence neutralize it to water, resulting in H2O2 scavenging [Citation52]. Previous study [Citation20] showed that AFL have a considerable antioxidant activity due to flavonoids as quercetin, and myricetin.
The phytochemical screening of AFL hexane extract revealed polyphenolic components such as triterpenoids, flavonoids, lupeol, phytol, and squalene. All of these compounds have been found to have significant antioxidant activity and may be responsible for AFL’s ability to protect cells against drug-induced toxicity. Also, gallic acid and alpha tocopherol are powerful antioxidants, according to several studies [Citation2,Citation48,Citation53], that preserve tissues from oxidative stress [Citation54]. Another study [Citation55] found that lupeol (derived from Ficus pseudopalma) possesses antioxidant, and cellular protective properties vs APAP-induced oxidative damage in rats. Also, the promising protective benefits of squalene (derived from Albiziaprocera) vs APAP– and CCl4–induced cellular damage in rats and mice, respectively, were reported by previous studies [Citation56,Citation57].
Phytol has been found to work as a source for vitamin E and also vitamin K1, as well as having antioxidant and anticancer properties [Citation2,Citation58]. Taking this effect into consideration, we can deduce that as the amount of exogenous polyphenolic diets increases, so does the amount of endogenous antioxidant system. As a result, pharmaceutical antioxidant therapeutics could be a potential strategy for preventing and/or treating diseases caused by excessive oxidative stress. In general, we can report that AMEAFL has important antioxidant effects on heart kidney, and spleen, such as the capacity to scavenge ROS, suppress LPO, and improve antioxidant defense system, which we attribute to its active pharmaceutical ingredients.
On the other hand, before any experiments on the AMEAFL to investigate its effect on HSV-1, the cytotoxicity of the AMEAFL on VERO cells must first be investigated. The biological activity of AMEAFL dilutions was tested against the viability of the VERO cell line.
Because efficient SI values should have very little cytopathic effect on cells while having a very high anti- HSV-1 activity, it can be concluded from plague reduction findings that the AMEAFL has a very promising result as a therapeutic agent of HSV-1, because almost all of its dilutions used were safe, and thus a high concentration can be used to give a high anti- HSV-1 action.
The AMEAFL’s virucidal activity against the HSV-1 revealed that a significant amount of the HSV-1 inhibition was attributable to direct virus inactivation caused by direct contact with virus particles. The concentration of polyphenol in the AMEAFL is directly proportional to the degree of viral inhibition. These findings are consistent with [Citation40,Citation59–61], who found that exposing cell-free HSV-1 to Rubus alleghaniensis (black berry) for 15 min at 37°C exhibited virucidal action.
In terms of the mode of action investigations, the fact that AMEAFL pretreated VERO cells showed very little viral suppression can lead to the conclusion that pretreatment of tissue culture cells with the investigated AMEAFL before virus inoculation had no influence on virus infectivity. Raenu et al. [Citation62] reported similar findings, concluding that the extracts they employed had no effect on HSV-1 infectivity when administered on VERO cells prior to HSV-1 inoculation.
On the other hand, our results showed that the AMEAFL inhibited virus replication to varying degrees depending on the concentration, by blocking virus adsorption either by deactivating adhesion of the surface glycoprotein of the viral envelope to the receptor sites on the cells, preventing viral cell fusion in the initial replication stage, and finally, inhibiting the early adsorption. Previous studies [Citation59,Citation63,Citation64] reported similar results, stating that the medicinal plant extracts they utilized immediately inactivated HSV-1 particles, resulting in early infection failure, including viral attachment and penetration [Citation65]. When the antiviral activity of AMEAFL vs HSV-1 was measured at various time intervals following HSV-1 inoculation, the findings revealed that the AMEAFL inhibited the virus to varying degrees depending on the time of addition. In addition, VERO cells treated 1–4 h after HSV-1 inoculation showed the greatest HSV-1 inhibition.
In vitro findings reveal that when the period between virus adsorption and AMEAFL treatment increases, the reduction of virus activity decreases considerably after four hours, eventually eliminating. These findings show that the viral suppression observed quickly after virus inoculation is mediated by the studied extract interfering with viral protein formation and transcription during viral replication’s early phases. Similar findings were observed by Sayed et al. [Citation66].
5. Conclusion
According to the findings, oxidative-stress plays a role in the pathophysiology of APAP-induced tissue damage. AMEAFL has been shown to protect against oxidative damage caused by an overdose of APAP. AFL’s protective impact could be attributed to its phytoconstituents, which have strong antioxidant and free radical scavenging activities. As a result, it is recommended that these medicinal plants be used as a dietary supplement in the diet to reduce the deleterious effects of APAP. As a result, more research is required in the future to establish the particular pathways through which our results exert their effects. Furthermore, in vitro experiments revealed that the AMEAFL has strong antioxidant and anti-herpetic properties, as well as a non-cytotoxic effect on VERO cells. As a result, the AMEAFL can be employed as adjuvants in synthetic antiviral treatments.
Abbreviations
AFL, Acrocarpus fraxinifolius leaves; ALT, Alanine aminotransferase; AMEAFL, aqueous methanol extract of AFL; APAP,N-acetyl-p-aminophenol; AST, Aspartate aminotransferase; CAT, Catalase; COX, Cyclooxygenases; GPx, Glutathione peroxidase; GR, Glutathione reductase; GSH, Reduced glutathione; LDH, Lactate dehydrogenase; NAPQI,N-acetyl-p-benzoquinoneimine; NO, nitric oxide; NSAIDs, Nonsteroidal anti-inflammatory drugs; TBARS: thiobarbituric acid reactive substance.
Availability of data and materials
The datasets supporting the conclusions of this article are included within the article and its additional files.
Disclosure statement
No potential conflict of interest was reported by the author(s).
References
- Garcia RLA, Hernandez-Diaz S. The risk of upper gastrointestinal complications associated with non-steroidal antiinflammatory drugs, glucocorticoids, acetaminophen, and combinations of these agents. Arthritis Res. 2001;3:98–101.
- El-Ghffar EA A, El-Nashar HAS, Eldahshan O, et al. GC-MS analysis and hepatoprotective activity of the n-hexane extract of Acrocarpus fraxinifolius leaves against paracetamol-induced hepatotoxicity in male albino rats. Pharm Biol. 2016;55:44–449.
- Howard PA, Delafontaine P. Nonsteroidal anti-Inflammatory drugs and cardiovascular risk. J Am Coll Cardiol. 2004;43:519–525.
- Ukwueze CS, Ukwueze CO, Nweze EC. Comparative study on the toxic effects of some non-steroidal anti-inflammatory drugs (NSAIDs) in rats. J Vet Adv. 2014;4:767–771.
- Hinson JA, Roberts DW, James LP. Mechanisms of acetaminophen-induced liver necrosis. Handb Exp Pharmacol. 2010;196:369–405.
- McGill MR, Williams CD, Xie Y, et al. Acetaminophen-induced liver injury in rats and mice: Comparison of protein adducts, mitochondrial dysfunction, and oxidative stress in the mechanism of toxicity. Toxicol. Appl. Pharmacol. 2012;264:387–394.
- Boutis K, Shannon M. Nephrotoxicity after acute severe acetaminophen poisoning in adolescents. Clin. Toxicol. 2001;39:441–445.
- Hamid Z A, Budin SB, Hamid A, et al. Nephroprotective effects of Zingiberzerumbet Smith ethyl acetate extract against paracetamol-induced nephrotoxicity and oxidative stress in rats. J Zhejiang Univ-Sci B (Biomed &Biotechnol. 2012;13:176–185.
- Jacob S, Cherian P, Preusz C, et al. Heart breaking case of acetaminophen poisoning. Circulation. 2008;118:e513–e514.
- Salem SI, Elgayed SSA, El-Kelany WM, et al. Diagnostic studies on acetaminophen toxicosis in dogs. Global Veterinaria. 2010;5:72–83.
- Mehrpour O, Afshari R, Delshard P, et al. Cardiotoxicity due to paracetamol overdose-A case study and review of the literature. Indian J Forensic Med Toxicol. 2011;5:31–34.
- Mandal M, Gardner CR, Sun R, et al. The spleen as an extramedullary source of inflammatory cells responding to acetaminophen-induced liver injury. Toxicol Appl Pharmacol. 2016;304:110–120.
- Ralapanawa U, Jayawickreme KP, Ekanayake EMM, et al. A study on paracetamol cardiotoxicity. BMC Pharmacol Toxicol. 2016;17:30–38.
- Satirapoj BMD, Lohachit PMD, Ruamvang TMD. Therapeutic dose of acetaminophen with fatal hepatic necrosis and acute renal failure. J Med Assoc Thai. 2017;90:1244–1247.
- Khan MT, Ather A, Thomson KD, et al. Extracts and molecules from medicinal plants against herpes simplex viruses. Antiviral Res. 2005;67:107–119.
- Collier L, Oxford J, Kellam P. The herpesviruses: general properties. In: Human virology, 2nd ed. United Kingdom: Oxford University Press; 2000. p. 131–140.
- Wagner EK, Hewlett MJ, Bloom DC, et al. Replication of some nuclear-replicating eukaryotic DNA viruses with large genomes. In: Basic virology, 3rd ed. United States: Blackwell Publishing Ltd; 2008. p. 331–351.
- El-Nashar HAS, Eldahshan O, Elshawi OE, et al. Phytochemical investigation, antitumor activity, and hepatoprotective effects of Acrocarpus fraxinifolius leaf extract. Drug Dev Res. 2017;78:210–226.
- AbouZeid AH, Mohammed R, Soliman FM, et al. Galloyle derivatives and biological activities of acrocarpusfraxinifolius weight and arn leaves. Planta Med. 2012;78:213–219.
- El-Kashak WA, Hamed AR, El-Raey M, et al. Antiproliferative, antioxidant and antimicrobial activities of phenolic compounds from Acrocarpus fraxinifolius. J Chem Pharm Res. 2016;8:520–528.
- El-Rafie HM, AbouZeid AH, Mohammed RS, et al. Bioactivities and phytochemical studies of Acrocarpus fraxinifolius Bark Wight and Arn. Int J Pharmacol Pharmac Sci. 2018;12:793.
- El-Ghffar EA A, Shehata SM. Antioxidant and anti-inflammatory effects of Acrocarpus fraxinifolius onhyperglycemia, hyperlipidemia and liver/kidney dysfunctions against alloxaninduced type 1 diabetes in rats. Indian J Tradknowl. 2018;17:223–232.
- Alaa B. Ameliorative effects of Acrocarpus fraxinifolius leaves extract against liver damage induced in wistar rats by acetaminophen. RJPBCS. 2018;9:153–161.
- Rice ECA, Miller NJ, Bolwell PG, et al. The relative antioxidant activities of plant-de-rived polyphenolic flavonoids. Free Radical Res. 1995;22:375–383.
- Gupta M, Mazumdar UK, Gomathi P, et al. Antioxidant and free radical scavenging activities of ervatamia coronaria Stapf. Leaves. Ira J Phan Res. 2004;2:119–126.
- Sreejayan N, Rao MNA. Nitric oxide scavenging bycurcuminoids. J Pharm Pharmacol. 1997;49:105–107.
- Marcocci L, Maguire JJ, Droy-Lafaix MT, et al. The nitric oxide scavenging property of Ginkgo biloba extracts EGb 761. Biochem Biophys Res Comm. 1994;201:748–755.
- Reitman S, Frankel SA. Colorimetric method determination of serum GOT (glutamic oxalacetic transaminase) GPT (glutamic pyruvic transaminase) activity. Amer J ClinPathol. 1975;28:56–63.
- Vassault A, Grafmeyer D, Naudin C, et al. Protocol for the validation of methods. Ann Biol Clin. 1986;44:686–645.
- Young DS, Pestaner LC, Gibberman V. Effects of drugs on clinical laboratory tests. Clin Chem. 1975;21:1D–432D.
- Fossati P, Prencipe L, Berti G. Use of 3,5-dichloro-2-hydroxybenzenesulfonic acid/4-aminophenazone chromogenic system in direct enzymic assay of uric acid in serum and urine. Clin Chem. 1980;26:227–231.
- Ohkawa H, Ohishi N, Yagi K. Assay for lipid peroxides in animal tissues by thiobarbituric acid reaction. Anal Biochem. 1979;95:351–358.
- Beutler E, Duron O, Kelly BM. Improved method for the determination of blood glutathione. J Lab Clin Med. 1963;61:882–888.
- Paglia DE, Valentine WN. Studies on the quantitative and qualitative characterization of erythrocyte glutathione peroxidase. J Lab Clin Med. 1967;70:158–169.
- Trease G, Evans SM. Pharmacognosy. 15th ed. Bailer Tindal, London: Elsevier Publisher; 2002. p. 23–67.
- Singleton VL, Rossi JA. Colorimetry of total phenolics with Phosphomolybdic-Phosphotungstic acid reagents. Am J Enol Vitic. 1965;16:144–158.
- Taga MS, Miller EE, Pratt DE. Chia seed as a source of natural lipid antioxidants. JAOCS. 1984;61:928–931.
- Goldberg DM, Spooner RJ. In Methods of enzymatic analysis (Bergmeyen, H.V. Ed.). 3rd ed., VerlogChemie, Deerfield beach, FI. 1983; Vol. 3, p. 258–265.
- Nishikimi M, Appaji N, Yagi K. The occurrence of superoxide anion in the reaction of reduced phenazine methosulfate and molecular oxygen. Biochem Biophys Res Commun. 1972;46:849–854.
- Aebi H. Catalase in vitro. Meth Enzymol. 1984;105:121–126.
- Simões CM, Amoros M, Girre L. Mechanism of antiviral activity of triterpenoidsaponins. Phytother Res. 1999;13:323–328.
- Ojo OO, Oluyege JO, Famurewa O. Antiviral properties of two Nigerian plants. African J Plant Sci. 2009;3(7):157–159.
- Abraham P. Oxidative stress in paracetamol-induced pathogenesis: (I) renal damage. Indian J Biochem Biophys. 2005;42:59–62.
- Shahidi F, Wanasundara PK. Phenolic antioxidants. Crit Rev Food Sci Nutr. 1992; 32:67e103.
- El-Menyiy N, Al-Waili N, El-Ghouizi A, et al. Evaluation of antiproteinuric and hepato-renal protective activities of propolis in paracetamol toxicity in rats. Nutr Res Pract. 2018;12(6):535–540.
- Yousef MI, Omar SA, El-Guendi MI, et al. Potential protective effects of quercetin and curcumin on paracetamol induced histological changes, oxidative stress, impaired liver, and kidney functions and haematotoxicity in rat. Food Chem Toxicol. 2010;48:3246–3261.
- Pacher P, Beckman JS, Liaudet L. Nitric oxide and peroxynitrite: in health and disease. Physiol Rev. 2007;87:315–424.
- Trouong VD, Mcfeeters RF, Thompson RT, et al. Phenolic acid content and composition in leaves and roots of common commercial sweet potato (Ipomea batatas L.) cultivars in the United States. J Food Sci. 2007;72(6):C343–C349.
- Donglin Z, Yasunori H. Phenolic compounds, and their antioxidant properties in different tissues of carrots (Daucuscarota L.). J Food Agric Environ. 2004;2:95–100.
- Rosales-Castro M, Honorato-Salazar JA, Gonz-alez-Laredo RF. Antioxidant phenolic compounds of ethanolic and aqueous extracts from pink cedar (Acrocarpus fraxinifolius Whight&Arn.) bark at two tree ages. J Wood Chem Technol. 2015;35:270–279.
- Landes N, Pfluger P, Kluth D, et al. Vitamin E activates gene expression via the pregnane X receptor. Biochem Pharmacol. 2003;65:269–273.
- Bendary E, Francis RR, Ali HMG, et al. Antioxidant and structure–activity relationships (SARs) of some phenolic and anilines compounds. Annal Agricul Sci. 2013;58:173–181.
- Gülçin I, Sat IG, Beydemir S, et al. Comparison of antioxidant activity of clove (Eugenia caryophylata Thunb) buds and lavender (Lavandulastoechas L.) J. Food Chem. 2004;87:393–400.
- El-Gendy AM. The beneficial effect of Trifolium flower extracts on paracetamol-intoxicated male rats. Egyptian J Hospit Med. 2012;49:771–780.
- Arimado J, Santiago L. The antioxidant and hepatoprotective activities of the ethyl acetate leaf extract of Ficuspseudopalma Blanco. Int J Pharmacog Phytochem Res. 2015;7:1179–1189.
- Sivakrishnan S, Muthu AK. Evaluation of hepatoprotective activity of squalene isolated from Albiziaprocera against paracetamol induced hepatotoxicity on Wistar rats. World J Pharm Pharmaceut Sci. 2014;3:1351–1362.
- Zuhan J, Jie J, Peijiang M, et al. Study of the antioxidative and hepatoprotective effects of squalene on acute liver injury mice induced by CCl4. J Zhejiang Tradit Chin Med. 2015;9:666–670.
- Oyugi DA, Ayorinde FO, Gugssa A, et al. Biological activity and mass spectrometric analysis of Vernoniaamygdalina fractions. J Biosci Tech. 2011;2:287–304.
- Kratz JM, Andrighetti-Fröhner CR, Kolling DJ, et al. Anti-HSV-1 and anti-HIV-1 activity of gallic acid and pentylgallate. MemInst Oswaldo Cruz. Rio de Janeiro. 2008;103(5):437–442.
- Raenu Y, Songyot A, Yingmanee T. Anti-herpes simplex virus activity of extracts from the culinary herpsOcimum sanctum L., Ocimumbascilicum L. African J Biotechnol. 2010;10(5):860–866.
- Danaher RJ, Wang C, Daj J, et al. Antiviral effects of blackberry extract against herpes simplex virus type 1. Oral Surg. 2011;112(3):31–35.
- Raenu Y, Sunee C, Yingmanee T. Inhibitory effect of aromatic herbs, lavender, sage and chamomile against herpes simplex virus infection. J Biotechnol. 2011;10(68):15394–15401.
- Pujol CA, Estevez JM, Carlucci MJ, et al. Novel DL-galactan hybrids from the red seaweed Gymnogongrustorulosusare potent inhibitors of herpes simplex virus and dengue virus. Antimicrob Agents Chemother. 2002;13(2):83–89.
- Xiang Y, Pei Y, Chang Q, et al. In vitro anti-herpes simplex virus activity of 1, 2, 4, 6-tetra- O-galloyl-β-D-glucose from Phyllamthusemblica L. (Euphorbiaceae). Phytotherapy Res. 2011;25:975–982.
- Arthanari SK, Vanitha J, Ganesh M, et al. Evaluation of antiviral and cytotoxic activities of methanolic extract of S. grandiflora (Fabaceae) flowers. Asian Pac J Trop Biomed. 2012;2(2):S855–S858.
- Sayed HM, Mahmoud SS, Hossein K, et al. Evaluation of in vitro antiviral activity of ChelidoniummajusL. against herpes simplex virus type-1. African J Microbiol Res. 2012;6(20):4360–4364.