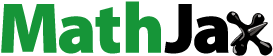
Abstract
Black point disease of wheat contributes to high economic losses every year. The overuse of fungicides resulted in pathogenic fungal resistance, health and environmental hazards that necessitate the formulation of safe biocontrol agents. Dual cultural assay of Trichoderma viride and Trichoderma harzianum strains proved their highest efficiency against Alternaria alternata with inhibition percentages of 75.04 and 67.83%, while the lowest activity was detected against Drechslera halodes with growth inhibition of 51.54 and 43.92%, respectively. Alternaria alternata and D. halodes strains exhibited carbendazim resistance, while Fusarium proliferatum was the most susceptible one. Chemical analysis of T. harzianum and T. viride extracts using gas chromatography-mass spectrometry indicated that 6-pentyl-α-pyrone and cyclooctanol compounds were the main active constituents representing about 26.43 and 32.74% respectively. The tested bioagents were highly effective against the fungal strains causing black point disease of wheat so that these biocontrol agents could be used in formulation of natural fungicides avoiding the harmful impact of the synthetic fungicides.
Abbreviations: FCZ; Fluconazole Antifungal agent, GC-MS; Gas chromatography-Mass spectrometry, MIC; Minimum inhibitory concentration, MFC; Minimum fungicidal concentration, PDA; Potato dextrose agar, T.H; Trichoderma harzianum, 1. T. V; Trichoderma viride
1. Introduction
Wheat (Triticum spp.) is a strategic crop belonging to the Poaceae family [Citation1]. Globally, wheat is the most predominant cereal crop, accounting for over 218 million ha [Citation2]. The annual production yield is evaluated as 735 million tons, with an estimated value of US$ 145 billion [Citation3]. Wheat has a high nutritional value due to its carbohydrate, protein, mineral, fat and vitamin content [Citation4]. Wheat grains are susceptible to infection with black point disease during grain filling or at milking stage [Citation5,Citation6]. Black point disease of wheat contributes to significant economic losses worldwide, with estimated yield losses of 24% [Citation7]. The disease has several harmful effects, such as decreased wheat quality, reduced seed germination and hindered seedling growth [Citation8,Citation9]. Fusarium spp., Alternaria spp., Bipolaris spp. and Drechslera spp. are fungal pathogens frequently associated with black point disease of wheat [Citation10]. However, frequent use of fungicides leads to environmental and health problems and results in the development of fungicide resistance [Citation11]. The use of antagonistic fungi as biological control agents is a potential alternative to fungicides, as it is an eco-friendly way to minimize seed fungal infections [Citation12]. These bioagents exhibited different mechanisms of action against pathogenic fungal strains including antibiosis, competition for nutrients and mycoparasitism [Citation13]. Trichoderma harzianum and T. viride strains were recorded as biological control agents against the concerned phytopathogenic strains and also as plant growth promoters [Citation14]. Furthermore, Trichoderma spp. can produce a wide range of secondary metabolites that protect against phytopathogenic fungi through various mechanisms, including antibiosis and mycoparasitism [Citation15]. These include several volatile compounds, such as ethylene, acetaldehyde and acetone, which account for their antibacterial and antifungal activity [Citation16,Citation17]. Trichoderma spp. exert their antagonistic effects by secreting antibiotics, such as herzianolide, trichodermin and trichodermol. Thus, T. viride and T. harzianum could be used as biological control agents against phytopathogenic fungal strains, such as Fusarium oxysporum, Alternaria alternata and Fusarium solani [Citation18]. The high global incidence of black point disease of wheat and the deleterious effects of chemical fungicides on the environment and human health necessitates the development of natural fungicides. Thus, this study aims to investigate the antagonistic effects of two Trichoderma strains (T. viride and T. harzianum) against four phytopathogenic fungal strains (A. alternata, B. sorokiniana, D. halodes and F. proliferatum) which cause black point disease of wheat.
2. Materials and methods
2.1. Plant fungal pathogens and antagonistic strains
The four phytopathogenic fungal strains (Fusarium proliferatum ATCC 74149, Alternaria alternata ATCC 66981, Bipolaris sorokiniana ATCC 32093, Drechslera halodes ATCC 32198) and two Trichoderma spp. (Trichoderma viride ATCC 18652 and Trichoderma harzianum ATCC 48131) were obtained from the Botany and Microbiology Department, College of Science, King Saud University. The fungal strains were subcultured on potato dextrose agar (PDA) slants, incubated at 25 ± 2°C for 5 days and finally stored in the refrigerator at 4°C until further use.
2.2. Evaluation of fungal antagonistic activity (Dual culture technique)
The antagonistic activity of Trichoderma viride and Trichoderma harzianum against fungal pathogens which cause black point disease of wheat was evaluated using the dual culture technique as previously described [Citation19]. Mycelial discs (6 mm in diameter) of fungal antagonistic strains were inoculated in PDA plates and placed at 1.5 cm from the edge of freshly prepared PDA plates. Similarly, 6 mm mycelial discs of fungal phytopathogens were placed in the opposite direction of the concerned antagonistic fungal strain at 1.5 cm from the edge of the prepared PDA plates. The control plates were inoculated with different fungal pathogens (Alternaria alternata, Bipolaris sorokiniana or Fusarium proliferatum) and incubated with the treated plates at 25 ± 2°C for 5 days. Afterwards, the radial growth of the phytopathogens in the control and treatment plates was measured and inhibition percentages were calculated using the following formula:
where A = colony diameter of the phytopathogen in the control plate, B = colony diameter in the dual culture plate.
2.3. Antifungal potency of culture filtrates of concerned antagonistic fungi
The percentage of growth inhibition (%) of the phytopathogenic fungal strains by the Trichoderma filtrates was evaluated. The two antagonistic fungal strains were grown in 50 mL of potato dextrose broth and incubated at 25 ± 2°C for 7 days in an orbital shaker at 150 rpm. Cell-free filtrates were obtained via filtration using double layers of muslin followed by centrifugation at 9000 rpm for 10 min to remove the fungal spores that may hinder membrane sterilization. Finally, the filtrates were sterilized using Millipore filters (22 µm). PDA medium supplemented with 25% Trichoderma spp. filtrates was poured in sterile Petri dishes and inoculated with 6 mm mycelial discs of the phytopathogenic fungal strains. The control plates were inoculated with 6 mm mycelial discs of different fungal pathogens. The plates were then incubated at 25 ± 2°C for 5 days. The radial growth diameters of the phytopathogens were then measured using a Vernier calliper. The inhibition of mycelial growth index (IMG %) was calculated using the following formula:
where A = radial growth diameter of the phytopathogens in the control plates, B = radial growth diameter of the phytopathogens in the treated plates [Citation20].
2.4. Slide culture technique to detect mycoparasitism relationship
PDA medium was prepared and poured into sterile Petri dishes. The medium was then cut with a sterilized blade, placed over a sterilized glass slide, and inoculated with Trichoderma spp. on one side and a pathogen strain on the opposite side. The slide was then incubated at 25 ± 2°C for 5 days, followed by removal of the PDA medium. The fungal mycelium was then stained with lactophenol cotton blue and a sterile coverslip was placed over the slide. The hyphal interactions between the Trichoderma spp. and fungal pathogens were observed using a light microscope (40×) [Citation21].
2.5. Antimicrobial activity of carbendazim (commonly used fungicide)
The food poisoning technique was used to detect the antifungal efficacy of carbendazim, a commonly used fungicide. Different carbendazim concentrations (50, 100, 150, 200, 250 and 300 ppm) were added to the PDA medium after sterilization. The plates were then inoculated with a 6 mm disc of fungal growth and incubated at 25 ± 2°C for 5 days. The growth diameter of the pathogenic fungi tested was measured using a Vernier calliper. The growth inhibition ratio (%) was calculated using the following equation:
where A = growth diameter in the control plates, B = growth diameter in the treated plates [Citation19].
2.6. Preparation of Trichoderma spp. crude extracts
The ability of Trichoderma strains to produce active metabolites which exhibit antifungal activity against fungal strains causing black point disease of wheat was investigated. The Trichoderma isolates were cultured onto PDA medium and incubated at 25 ± 2°C for 7 days. The fungal growth was then scraped from the agar surface to obtain a mycelial sample of 5 g. Then, the mycelial growth was added to 200 mL of 80% ethanol supplemented with 0.2 M HCl and incubated in a rotatory shaker at 25 ± 2°C for 24 h. The samples were then centrifuged at 9000 rpm to separate the solid components. The extraction procedure was performed again for the solid components, using ethyl acetate as a solvent. Finally, the extracts were combined and partially evaporated using a rotatory evaporator. The yield was calculated using the following equation:
where R = extract residue, S = weight of the raw mycelial sample [Citation22].
2.7. Antifungal efficacy of Trichoderma spp. extracts against fungal pathogens
The disc diffusion method was used to evaluate the antifungal activity of Trichoderma spp. extracts against phytopathogenic fungi which cause black point disease of wheat. Fifteen mL of PDA medium was poured in sterile Petri dishes as a basal medium, followed by 10 mL of seeded medium previously inoculated with a suspension of spores from the fungal phytopathogens tested. Then, filter paper discs (8 mm diameter) were loaded with different concentrations of Trichoderma spp. crude extracts (50, 100, 150, 250, 300 and 400 µg/disc) and placed over the seeded plates [Citation19]. Filter paper discs with 25 µg of fluconazole, a reference antifungal agent, were used as positive controls. The plates were then incubated at 25 ± 2°C for 5 days. The diameters of the inhibition zones were measured using a Vernier calliper and the lowest concentration of Trichoderma spp. extract which exhibited an inhibitory effect against the phytopathogens tested was considered the minimum inhibitory concentration (MIC). The minimum fungicidal concentration (MFC) was obtained by plating an inoculum from the inhibition zones into freshly prepared PDA medium. The lowest concentration with no fungal growth was considered the MFC.
2.8. GC-MS analysis of the T. viride and T. harzianum extracts
Chemical analyses of T. harzianum and T. viride extracts were achieved to detect the active constituents exhibiting antifungal activity. GC-MS was performed using an Agilent 7890 gas chromatograph and an Agilent 5975C Mass Spectrometer, USA. Conditions of analysis were optimized as stated by Yassin et al. Citation2020 [Citation23]. Identification of active components of Trichoderma extracts was achieved by comparison of their mass spectrum with reference standards in NIST database.
2.9. Statistical analysis
Data were statistically analyzed by GraphPad Prism 5.0 (GraphPad Software, Inc., La Jolla, CA, USA) using one-way analysis of variance. The experiments were done in triplicates and the data were presented as a mean of triplicates ± standard error.
3. Results
3.1. Antagonistic antifungal activity of Trichoderma strains
Dual culture results showed that both T. viride and T. harzianum strains limited the growth of different fungal phytopathogens with different growth inhibition patterns as shown in Figure . T. viride strain showed the highest antagonistic activity against different phytopathogenic fungal strains compared to T. harzianum strain. Alternaria alternata was the most susceptible strain to the concerned antagonistic fungal strains (T. viride and T. harzianum) recording inhibition percentages of 75.04 and 67.83%, respectively (Table ). In contrast, the lowest antagonistic activities of T. viride and T. harzianum strains were estimated against D. halodes recording growth inhibition percentages of 51.54 and 43.92%, respectively. Furthermore, the results revealed that T. viride exerted antagonistic action against B. sorokiniana and F. proliferatum with growth inhibition percentages of 62.65 and 64.23%, while T. harzianum showed inhibition percentages of 59.04 and 53.42%, respectively.
Figure 1. Antagonistic activity of T. viride and T. harzianum strains against different phytopathogenic strains.
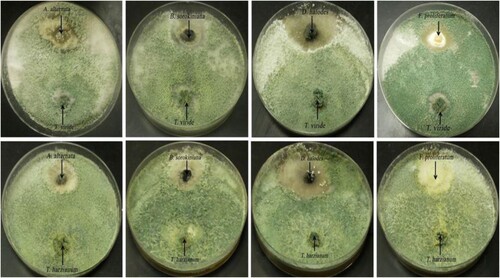
Table 1. Antagonistic activity of T. harzianum and T. viride against the concerned fungal phytopathogens. (T.h, T. harzianum; T.v, T. viride).
3.2. Antifungal potency of culture filtrates of concerned antagonistic fungi
Cultural filtrates of the antagonistic fungal strains (T. harzianum and T. viride) exhibited antifungal potency against different pathogenic fungal strains. T. viride filtrates demonstrated the maximum growth inhibition against B. sorokiniana (57.97%) followed by D. halodes (42.90%), A. alternata (30.46%) and F. proliferatum (19.02%). On the other hand, the cultural filtrates of T. harzianum exhibited the highest anti-phytopathogenic activity against B. sorokiniana followed by D. halodes, A. alternata and F. proliferatum recording growth inhibition percentages of 53.06, 40.81, 28.72 and 6.97%, respectively, as seen in Table .
Table 2. Inhibitory effect of the culture filtrates of antagonistic strains (T. harzianum andT. viride) against wheat fungal phytopathogens.
3.3. Slide culture technique to detect mycoparasitism relationship
T. harzianum strain exhibited mycoparasitism against the tested phytopathogenic fungal isolates except D. halodes strain, while T. viride showed no mycoparasitic action. The antagonistic T. harzianum isolate exhibited parasitic action against A. alternata, B. sorokiniana and F. proliferatum strains through different mechanisms of action as seen in Figure . The mycoparasitic behaviour included coiling of Trichoderma hyphae around the phytopathogenic hyphae, formation of penetration peg and disintegration of pathogenic hyphae.
3.4. Fungicidal activity of carbendazim (reference fungicide)
Carbendazim as a reference fungicide showed highly fungicidal activity against F. proliferatum strain at all tested concentrations, while D. halodes and A. alternata exhibited carbendazim resistance. Furthermore, carbendazim was moderately effective against B. sorokiniana strain with inhibition percentage of 69.08% at 1.00 ppm concentration. In addition, the MIC of carbendazim against B. sorokiniana was 0.50 ppm and the mycelial growth was completely suppressed at MFC of 2.00 ppm (Table ).
Table 3. Effect of different concentrations of fungicide (carbendazim) on mycelial growth of wheat phytopathogenic fungi. (A. a, Alternaria alternata; B.s, Bipolaris sorokiniana; D. h, Drechslera halodes; F. p, Fusarium proliferatum).
3.5. Antifungal efficacy of Trichoderma spp. extracts against fungal pathogens using disc diffusion method
T. viride extract exerted the highest antifungal activity against different phytopathogenic strains compared to T. harzianum extract. B. sorokiniana was the most sensitive strain to T. harzianum and T. viride extracts recording suppressive zone diameters of 11.36 and 12.29 mm, respectively at a concentration of 200 µg/disc as seen in Table . In contrast, A. alternata exhibited the lowest susceptibility to T. harzianum and T. viride strains with MIC values of 300 µg/disc, respectively. The MFC of T. viride extract against different phytopathogenic strains was 300 µg/disc. Moreover, T. harzianum extract showed fungicidal activity against B. sorokiniana and D. halodes with MFC value of 300 µg/disc, while it was 400 µg/disc against A. alternata and D. halodes strains.
Table 4. Antifungal activity of T. viride and T. harzianum extracts against different phytopathogenic fungal strains.
3.6. GC-MS analysis of the T. viride and T. harzianum extracts
GS-MS of T. viride and T. harzianum was performed to detect different active ingredients exhibiting anti-phytopathogenic activity. 6-pentyl-α-pyrone (26.43%) was the predominant active component of T. harzianum extract followed by hexadecanoic acid (15.98%), acetic acid (12.84%), 2-phenylethyl alcohol (11.36%), 2-butoxyethyl acetate (8.53%), 1-methoy-2-propanone (7.29%), 1-hexadecanol (6.45%), isopentyl acetate (4.65%), dioctyl ester, hexanedioic acid (4.24%) and 9-eicosane (2.23%) as demonstrated in Table . In contrast, the major active component of T. viride was cyclooctanol (32.74%) followed by propyl-benzene (21.65%), cadinene (11.23%), epizonaren (9.34%), d- limonene (8.49%), α-bisabolol (7.32%), β-farnesene (6.78%) and propanoic acid (2.45%) as seen in Table 6.
Table 5. GC-MS analysis of the active components of T. harzianum extract.
Table 6. GC-MS analysis of the active components of T. viride extract.
4. Discussion
Trichoderma spp. showed a potential biocontrol efficiency against fungal phytopathogens either through direct mechanisms of mycoparasitism and antibiosis or by indirect mechanisms as competition for nutrients, enhancing plant defence responses and exhilarating plant growth [Citation24]. In this regard, Trichoderma bioagents suppress the growth of fungal phytopathogens by a mixed action through their metabolites inhibitory effect and lytic enzymes which disintegrate the fungal cell wall [Citation25]. Trichoderma spp. have been ascertained as biological control agents against several phytopathogenic fungal strains [Citation26]. The current study confirmed the potent activity of T. viride and T. harzianum against fungal pathogenic strains causing black point disease of wheat. Dual cultural assay indicated that T. viride strain exerted the highest antagonistic potency against A. alternata (75.04%) followed by F. proliferatum (64.23%), B. sorokiniana (62.65%) and D. halodes (51.54%), while T. harzianum exhibited a moderate efficacy against A. alternata followed by B. sorokiniana, F. proliferatum and D. halodes with corresponding inhibition percentages of 67.83%, 59.04%, 53.42% and 43.92%, respectively. The proved antagonistic efficacies of T. viride and T. harzianum against fungal strains causing black point disease were coincident with that reported by Ghorbanpour et al., Citation2018 and Adnan et al., Citation2019 [Citation27,Citation28]. Moreover, the metabolites produced by Trichoderma spp. may play a major role in suppressing the growth of phytopathogenic fungi as the hyphae of pathogens obviously collapsed between the interaction zones as clearly presented by dual culture assay. Several researchers attributed the formation of clear interaction zone of hypha disappearance of pathogenic fungi to the action of secondary metabolites as gliotoxin produced by Trichoderma spp which was believed to play an important role in the antibiosis process [Citation29,Citation30]. On the other hand, the potent biological activities of Trichoderma strains may be also assigned to their high colonization rates resulting in suppression of the competitive microorganisms [Citation28].
In contrast, resistance of A. alternata and D. halodes to carbendazim fungicide was detected in the current study at different concentrations. The previous result was coincident with that of Yang et al., Citation2019 who reported the resistance of A. alternata to a number of agricultural fungicides [Citation31]. The development of fungicides resistance may be due to alteration of fungicides target sites in fungal cell or to the fungal enzymatic breakdown of these fungicides [Citation32].
In our current study, T. harzianum exhibited mycoparasitism against A. alternata, F. proliferatum and B. sorokiniana, while no mycoparasitic action was detected against D. halodes. In this regard, the mycoparasitic activity of T. harzianum against B. sorokiniana was demonstrated by coiling of the Trichoderma hyphae around the hyphae of pathogenic fungus. Furthermore, hyphal penetration of T. harzianum into A. alternata, B. sorokiniana and F. proliferatum hyphae was proven as another mycoparasitic mode of action [Citation33,Citation34]. On the other hand, the mycoparasitic action may be powered by number of extracellular enzymes that disintegrate fungal hyphae of phytopathogenic fungi as chitinases and cellulases facilitating hyphal penetration of Trichoderma bioagents [Citation35,Citation36]. The same explanations exposing these modes of fungal parasitism against the concerned phytopathogenic fungi were also documented by Keswani et al., Citation2019 and Sornakili et al., Citation2020 [Citation37,Citation38].
GC-MS analysis showed that 6-pentyl-α-pyrone (26.43%) was the main active ingredient of T. harzianum extract which was recorded to possess antimycotic activity [Citation39]. The previous concept was ascertained by Pandey et al., Citation2019 who reported the antimycotic activity of the previous compound against fungal pathogens causing plant diseases [Citation40]. The antifungal volatile constituents as 6-pentyl-α-pyrone produced by Trichoderma harzianum were found to suppress the fungal growth of phytopathogenic fungi through mycofumigation avoiding side effects of using chemical fungicides [Citation41,Citation42]. Moreover, 6-pentyl-α-pyrone has the capability of inhibiting fusaric acid which is a fungal mycotoxin produced by fusarial phytopathogens [Citation43]. Other volatile active components of T. harzianum extract including hexadecanoic acid, acetic acid, 2-phenylethanol, isopentyl acetate, 1-hexadecanol and eicosane were mentioned to exert antifungal activity in previous literature [Citation44–48].
On the other hand, the other components as cyclooctanol, propyl-benzene and cadinene were the main chemical constituents of T. viride extract with relative percentages of 32.74, 21.65 and 11.23%, respectively. GC-MS results were in accordance with the previous study reported by Awad et al., Citation2018 which proved that cyclooctanol and propyl-benzene were the main active compounds of T. viride extract recording 8.48% and 41.75%, respectively. Furthermore, the previous constituents were proved to exert antifungal efficiency against fungal phytopathogens [Citation19]. The sesquiterpene compounds of T. viride extract as d- limonene, β-farnesene, α-bisabolol and cadinene were also reported to possess antifungal activity [Citation49–52]. Due to the presence of many bioactive constituents, the antifungal activities of Trichoderma strains cannot be referred to one bioactive component only, but also to the synergism among several bioactive constituents of Trichoderma extracts [Citation53].
5. Conclusion
Fungal strains (T. viride and T. harzianum) exhibited strong antagonistic activities against fungal phytopathogens causing black point disease of wheat. T. viride exhibited a higher antagonistic activity than T. harzianum strain against the carbendazim resistant phytopathogenic fungi. The high antagonistic activity of Trichoderma strains against fungal pathogens causing black point disease of wheat encourages performing further in vivo studies for the application of these bioagents as natural fungicides avoiding the harmful impacts of synthetic fungicides.
Availability of data and materials
The datasets used and/or analyzed during the current study are available from the corresponding author on reasonable request.
Acknowledgement
The authors extend their appreciation to the Researchers Supporting Project number (RSP-2021/362), King Saud University, Riyadh, Saudi Arabia.quest.
Disclosure statement
No potential conflict of interest was reported by the author(s). quest.
Additional information
Funding
References
- Mourad AM, Alomari DZ, Alqudah AM, et al. Recent advances in wheat (Triticum spp.) breeding. In: Al-Khayri M, Jain SM, Johnson DV, editors. Advances in Plant Breeding Strategies: Cereals Vol. 5. New York (NY): Springer International Publishing; 2019. p. 559–593.
- Giraldo P, Benavente E, Manzano-Agugliaro F, et al. Worldwide research trends on wheat and barley: a bibliometric comparative analysis. Agronomy. 2019;9:352.
- FAO. FAOSTAT database collections. Rome: Food and Agriculture Organization of the United Nations; 2019.
- Carcea M. Nutritional value of grain-based foods. Foods. 2020;9:504.
- Xu K-G, Jiang Y-M, Li Y-K, et al. Identification and pathogenicity of fungal pathogens causing black point in wheat on the North China plain. Indian J Microbiol. 2018;58:159–164.
- Bashyal B, Rawat K, Sharma S, et al. Major seed-borne diseases in important cereals: symptomatology, aetiology and economic importance seed-borne diseases of agricultural crops: detection, diagnosis & management. Vol. 1. New York (NY): Springer International Publishing; 2020. p. 371–426.
- Figueroa M, Hammond-Kosack KE, Solomon PS. A review of wheat diseases – a field perspective. Mol Plant Pathol. 2018;19:1523–1536.
- Abdullah M, Zulkiffal M, Din A, et al. Discrepancy in germination behavior and physico-chemical quality traits during wheat storage. J Food Process Pres. 2019;43:e14109.
- Li Q-Y, Xu Q-Q, Jiang Y-M, et al. The correlation between wheat black point and agronomic traits in the North China plain. Crop Prot. 2019;119:17–23.
- El-Gremi SM, Draz IS, Youssef WAE. Biological control of pathogens associated with kernel black point disease of wheat. Crop Prot. 2017;91:13–19.
- Brauer VS, Rezende CP, Pessoni AM, et al. Antifungal agents in agriculture: friends and foes of public health. Biomolecules. 2019;9:521.
- Köhl J, Kolnaar R, Ravensberg WJ. Mode of action of microbial biological control agents against plant diseases: relevance beyond efficacy. Front Plant Sci. 2019;10:845.
- Sharma PK, Gothalwal R. Trichoderma: a potent fungus as biological control agent agro-environmental sustainability. Vol. 1. New York (NY): Springer International Publishing; 2017. p. 113–125.
- Meena M, Swapnil P, Zehra A, et al. Antagonistic assessment of Trichoderma spp. by producing volatile and non-volatile compounds against different fungal pathogens. Arch Phytopathology Plant Protect. 2017;50:629–648.
- Sood M, Kapoor D, Kumar V, et al. Trichoderma: The “secrets” of a multitalented biocontrol agent. Plants. 2020;9:762.
- Piechulla B, Lemfack MC, Kai M. Effects of discrete bioactive microbial volatiles on plants and fungi. Plant Cell Environ. 2017;40:2042–2067.
- Ghorbanpour M, Omidvari M, Abbaszadeh-Dahaji P, et al. Mechanisms underlying the protective effects of beneficial fungi against plant diseases. Biol Control. 2018;117:147–157.
- Ramírez-Cariño HF, Guadarrama-Mendoza PC, Sánchez-López V, et al. Biocontrol of Alternaria alternata and Fusarium oxysporum by Trichoderma asperelloides and bacillus paralicheniformis in tomato plants. Antonie van Leeuwenhoek. 2020;113:1247–1261.
- Awad NE, Kassem HA, Hamed MA, et al. Isolation and characterization of the bioactive metabolites from the soil derived fungus Trichoderma viride. Mycology. 2018;9:70–80.
- Bunbury-Blanchette AL, Walker AK. Trichoderma species show biocontrol potential in dual culture and greenhouse bioassays against Fusarium basal rot of onion. Biol Control. 2019;130:127–135.
- Naglot A, Goswami S, Rahman I, et al. Antagonistic potential of native T. viride strain against potent tea fungal pathogens in North east India. Plant Pathol J. 2015;31:278.
- Synytsya A, Monkai J, Bleha R, et al. Antimicrobial activity of crude extracts prepared from fungal mycelia. Asian Pac J Trop Biomed. 2017;7:257–261.
- Yassin MT, Mostafa AA, Al-Askar AA. In vitro anticandidal potency of syzygium aromaticum (clove) extracts against vaginal candidiasis. BMC Complement Altern Med. 2020;20:25.
- Bhardwaj N, Kumar J. Characterization of volatile secondary metabolites from Trichoderma asperellum. J Appl Nat Sci. 2017;9:954–959.
- Dukare AS, Singh RK, Jangra RK, et al. Non-fungicides-based promising technologies for managing post-production penicillium induced spoilage in horticultural commodities: a comprehensive review. Food Rev Int. 2020: 1–41.
- Mazrou YS, Makhlouf AH, Elseehy MM, et al. Antagonistic activity and molecular characterization of biological control agent Trichoderma harzianum from Saudi arabia. Egypt J Biol Pest Co. 2020;30:4.
- Adnan M, Islam W, Shabbir A, et al. Plant defense against fungal pathogens by antagonistic fungi with Trichoderma in focus. Microb Pathog. 2019;129:7–18.
- Bizos G, Papatheodorou EM, Chatzistathis T, et al. The role of microbial inoculants on plant protection, growth stimulation, and crop productivity of the olive tree (Olea europea L). Plants. 2020;9(6):743.
- Tomah AA, Abd Alamer IS, Li B, et al. A new species of Trichoderma and gliotoxin role: a new observation in enhancing biocontrol potential of T. virens against phytophthora capsici on chili pepper. Biol Control. 2020;145:104261.
- Khan RAA, Najeeb S, Hussain S, et al. Bioactive secondary metabolites from Trichoderma spp. against phytopathogenic fungi. Microorganisms. 2020;8(6):817.
- Yang L-N, He M-H, Ouyang H-B, et al. Cross-resistance of the pathogenic fungus Alternaria alternata to fungicides with different modes of action. BMC Microbiol. 2019;19:205.
- Baibakova EV, Nefedjeva EE, Suska-Malawska M, et al. Modern fungicides: mechanisms of action, fungal resistance and phytotoxic effects. Annu Res Rev Biol. 2019;32(3):1–16.
- Parulekar-Berde CV, Joshi SA, Berde VB. Fungal communities as biological control agents for different phytopathogenic organisms Recent Trends in mycological Research. New York (NY): Springer; 2020. p. 189–201.
- Asad SA, Tabassum A, Hameed A, et al. Determination of lytic enzyme activities of indigenous Trichoderma isolates from Pakistan. Braz J Microbiol. 2015;46:1053–1064.
- Bhat K. A New agar plate assisted slide culture technique to study mycoparasitism of Trichoderma spp. on Rhizoctonia solani and Fusarium oxysporium. Int J Curr Microbiol Appl Sci. 2017;6:3176–3180.
- Rajani P, Rajasekaran C, Vasanthakumari M, et al. Inhibition of plant pathogenic fungi by endophytic Trichoderma spp. through mycoparasitism and volatile organic compounds. Microbiol Res. 2021;242:126595.
- Keswani C, Singh HB, Hermosa R, et al. Antimicrobial secondary metabolites from agriculturally important fungi as next biocontrol agents. Appl Microbiol Biotechnol. 2019;103:9287–9303.
- Sornakili A, Thankappan S, Sridharan A, et al. Antagonistic fungal endophytes and their metabolite-mediated interactions against phytopathogens in rice. Physiol Mol Plant Path. 2020;112:101525.
- Ismaiel AA, Ali DM. Antimicrobial properties of 6-pentyl-α-pyrone produced by endophytic strains of Trichoderma koningii and its effect on aflatoxin B1 production. Biologia. 2017;72(12):1403–1415.
- Pandey M, Ahmad S, John SA, et al. Antifungal potential of native Trichoderma isolates against pythium aphanidermatum causing chilli damping-off. Ann Plant Prot Sci. 2019;27:102–106.
- Alfiky A, Weisskopf L. Deciphering trichoderma–plant–pathogen interactions for better development of biocontrol applications. Journal of Fungi. 2021;7(1):61.
- Degani O, Khatib S, Becher P, et al. Trichoderma asperellum secreted 6-pentyl-α-pyrone to control magnaporthiopsis maydis, the Maize late wilt disease agent. Biology (Basel). 2021;10(9):897.
- Sharma VS, Sharma PR. The comparative mechanistic aspects of Trichoderma and probiotics: scope for future research. Physiol Mol Plant Pathol. 2017;100:84–96.
- Ahsan T, Chen J, Zhao X, et al. Extraction and identification of bioactive compounds (eicosane and dibutyl phthalate) produced by streptomyces strain KX852460 for the biological control of Rhizoctonia solani AG-3 strain KX852461 to control target spot disease in tobacco leaf. AMB Express. 2017;7:54.
- Mookherjee A, Bera P, Mitra A, et al. Characterization and synergistic effect of antifungal volatile organic compounds emitted by the geotrichum candidum PF005, an endophytic fungus from the eggplant. Microb Ecol. 2018;75:647–661.
- Liu Q, Yin G, Zhang J. The suitable condition for lactobacillus parafarraginis ZH1 producing hexadecanoic acid and inhibiting pathogenic and spoilage yeasts. Biol Control. 2020;149:104318.
- Debonne E, Vermeulen A, Bouboutiefski N, et al. Modelling and validation of the antifungal activity of DL-3-phenyllactic acid and acetic acid on bread spoilage moulds. Food Microbiol. 2020;88:103407.
- Zhang H, Du H, Xu Y. Volatile organic compound-mediated antifungal activity of pichia spp. and Its effect on the metabolic profiles of fermentation communities. Appl Environ Microbiol. 2021;87(9):e02992–e02920.
- Wang H, Yang Z, Ying G, et al. Antifungal evaluation of plant essential oils and their major components against toxigenic fungi. Ind Crops Prod. 2018;120:180–186.
- He X, Zhang L, Chen J, et al. Correlation between Chemical composition and antifungal activity of clausena lansium essential Oil against candida spp. Molecules. 2019;24:1394.
- Cai R, Hu M, Zhang Y, et al. Antifungal activity and mechanism of citral, limonene and eugenol against zygosaccharomyces rouxii. Lwt. 2019;106:50–56.
- de Medeiros CA, ÂdV P, de Oliveira JC, et al. Evaluating the antifungal activity of α-bisabolol in association with NaCl on Fusarium oxysporum in Maize grains. Curr Microbiol. 2021;78(2):604–610.
- Khruengsai S, Pripdeevech P, D’Souza PE, et al. Biofumigation activities of volatile compounds from two Trichoderma afroharzianum strains against Fusarium infections in fresh chilies. J Sci Food Agric. 2021;101:5861–5871.