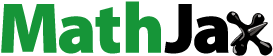
Abstract
The inhibition performance of expired Tamsulosin (TAM) was studied on St52 pipeline steel in 1 M HCl by gravimetric, electrochemical impedance spectroscopy (EIS), potentiodynamic polarization (PDP), scanning electron microscopy (SEM) and energy-dispersive X-ray (EDX) spectroscopic analysis. It was seen that the presence of the studied expired drug decreased the corrosion rate of the pipeline steel. TAM’s efficiency as an inhibitor increased as its concentration increased but decrease with temperature rise. The expired drug showed maximal inhibition efficiency of 98.1% from weight loss measurement at 2.0 × 10−3 M TAM concentration and 303 K. PDP results showed that TAM acted as a mixed-type inhibitor. The adsorption study revealed that Langmuir isotherms gave the best fit. The surface morphological study revealed that TAM formed an adsorbed protective layer on the St52 steel surface in the acid medium. The theoretical study performed using the density functional theory (DFT) allowed correlation with an experimental study.
1. Introduction
Carbon steel with different grades is exploited as pipework steel in the petroleum industry due to its good mechanical properties. The exposure of pipeline steel to aggressive environments as a result of different industrial applications endangers the steel. One of such industrial applications is the use of acids usually hydrochloric acids in oil-well acidizing. This process, as good as it is, leads to acid corrosion of the steel. Therefore, efforts are being made to prevent these unwanted reactions. In the petroleum industry, the corrosion inhibitor is usually introduced to the solution containing the acid in the acidizing process to prevent the dissolution of the steel [Citation1]. The interaction of inhibitor with corroding steel surface significantly modifies the rate of the corrosion process. Immense efforts have been put in by researchers at developing appropriate corrosion inhibitors in various corrosive media [Citation2,Citation3].
Different types of organic compounds comprising π-electrons, aromatic rings and heteroatoms like S, O and N have been applied as inhibitors of metal corrosion in acidic environments [Citation4–7]. The presence of these heteroatoms and the π electrons provides adsorption sites for the inhibitor on steel surfaces, thereby creating an impediment to acid corrosion attack [Citation8]. The adsorption of organic inhibitors onto metal surfaces depends mostly on the various factors which includes the inhibitor molecules' electronic structure, functional groups, aromaticity, steric factors, the donating electrons p-orbital character and electron density at the donor atoms [Citation9]. These inhibitors form complexes when they react with the Fe atom at the steel surface, blocking the steel surface from further deterioration [Citation10].
Several research works have been carried out to ascertain the inhibitive performance of various drugs on various metals [Citation11–20]. Recently, some fresh and expired pharmaceutical compounds have been investigated as potential inhibitors both in our laboratory and by other researchers, including carvedilol [Citation21], glimepiride [Citation22], domperidone [Citation23] and ethambutol [Citation24]. In continuance of our previous research, the evaluation of the performance of expired tamsulosin (TAM) as corrosion inhibitor for St52 pipeline steel in 1M HCl medium was examined using weight loss, EIS, PDP and scanning electron microscopy (SEM) techniques. Tamsulosin is a medication used in the treatment of chronic prostatitis and also to help with kidney stones passage, among others [Citation25]. Considering the high cost of most pharmaceutical drugs, we opted to study the inhibitive performance of the expired drug which is useless as it were. Reports show that above 90% of pharmaceutical drugs are still stable after expiration [Citation26]. TAM has the formula of C20H28N2O5S and a molar mass of 408.51 g/mol. The molecular and 3D ball and stick structures of TAM are shown in Figure . The comparison of the inhibitive performance of TAM with other drugs and with already reported standard corrosion inhibitors are presented in Table .
Table 1. Comparison of the inhibitive performance of TAM with other reported drug corrosion inhibitors.
2. Experimental
2.1. Materials preparation:
St52 steel sheets specimens with composition (weight %), C 0.18, Si 0.50, P 0.05, Mn 1.50, S 0.05 and the balance Fe were used for the experiments (surface morphology, electrochemical and weight loss measurements). The sheets were cut into dimensions of 4 cm × 2 cm × 0.02 cm, and for the electrochemical study, the steel samples were enclosed with epoxy resin exposing 1 cm2 area. The samples were abraded successively with SiC2 paper (800, 1000 and 1200 grades), to secure a smooth surface. The St52 steels were thereafter washed with water, degreased in acetone, and then, finally dried in an oven before weighing and use. The acid test solution (1M HCl) was made by using 37% HCl analytical grade from Merck and distilled water.
2.2. Corrosion inhibitor
The expired Tamsulosin (TAM) drug (Exp. Date: July 2019) was supplied by Naiviv Pharmacy Limited. The drug was crushed into powder and used without further purification. Different concentrations of TAM ranging from 4.9 × 10−4 to 2.0 × 10−3 M were prepared by the dissolution of the weighed amount of the expired drug in 1M HCl.
2.3. Weight loss studies
Pre-treated and accurately weighed St52 steel samples were submerged in 1M HCl aggressive solutions comprising different TAM concentrations and bare acid at 30°C. The samples being removed after 4 h were washed thoroughly, dried and accurately reweighed. The difference in the steel weights before immersion and after was taken as weight loss. The weight loss tests were repeated thrice, and the mean value was recorded to ensure reproducibility of the results. The tests were repeated at 40, 50 and 60°C. From weight loss data, the inhibition efficiency () and surface coverage (
) of each concentration of TAM were calculated utilizing the equation:
(1)
(1)
(2)
(2) where
and
represent the weight loss without and in presence of TAM, respectively. The corrosion rate (
) with and in the absence of different concentrations of TAM were computed by using the equation:
(3)
(3) where
represents weight loss, A represents an area of St52 steel (cm2) exposed and
stands for time (
) of immersion.
2.4. Electrochemical measurements
Electrochemical studies were carried out in a three-electrode electrochemical cell of 500 mL capacity using VERSASTAT 400 full set DC voltammetry and a potentiodynamics/Galvonstat system with E-chem software. The cell consists of St52 steel with 1 cm2 area exposed as working electrode, platinum counter electrode, and the reference electrode is SCE – saturated calomel electrode. Before the experiment, the St52 steel was immersed in 1M HCl test solution with and in the absence of TAM for sufficient time (3600s) until steadying open circuit potential (OCP) is achieved. For each inhibitor concentration, the experiments were performed three times for reproducibility. EIS tests were performed in a range of 0.1–100 kHz frequency at AC amplitude of 10 mV. The charge-transfer resistance () values were deduced from Nyquist plots. Inhibition efficiency (
) of TAM was computed from the
values utilizing the expression [Citation27]:
(4)
(4) where
and
are the charge-transfer resistances with and without TAM, respectively.
The polarization study was performed by adjusting potential from −0.25 to +0.25 V versus SCE at 1 mV/s scan rate with respect to OCP. The current density () was deduced by the Tafel plots cathodic and anodic linear segments extrapolation. The inhibition efficiency (
) was computed from the
values using the relation [Citation27]:
(5)
(5) where
and
represent corrosion current densities without and with TAM, respectively.
2.5. SEM/EDX studies
Ziess 50 XVP scanning electron microscope was utilized to perform the SEM/EDX study. The pre-treated St52 steel samples were immersed in 1M HCl medium without and with 2.0 × 10−3 M of TAM at 30°C. The samples were removed after 6 h, rinsed in water, dried prior to SEM/EDX analysis.
2.6. Theoretical calculation
Quantum chemical calculations were performed to correlate experimental results with theoretically calculated properties. Quantum chemical parameters were computed utilizing DFT. The geometrical optimizations of the studied TAM molecules (neutral and protonated) were performed with B3LYP along with basis set 631
(d, p), implemented in the Spartan 14.0 package. The following parameters were obtained:
, highest occupied molecular orbital energy;
, lowest unoccupied molecular orbital energy; and
, dipole moment. The obtained
and
were utilized in calculating other quantum chemical parameters which include energy band gap (
), electronegativity (
), global softness (
) and hardness (
) as expressed in the following equations [Citation28,Citation29]:
(6)
(6)
(7)
(7)
(8)
(8)
(9)
(9)
The fraction of transferred electron from TAM molecule to the Fe surface () was computed using the expression [Citation30]:
(10)
(10) where
and
are respectively the Fe and inhibitor electronegativity values, while
and
represent the global hardness for Fe and the inhibitor (TAM). Pearson's scale of electronegativity designate
for
and
for
bulk Fe [Citation31].
3. Results and discussion
3.1. Gravimetric measurements
3.1.1. Effect of TAM concentration
The gravimetric method of monitoring metal corrosion is used due to its reliability and simple application. The variation of and
of TAM with inhibitor concentrations at varying temperatures is shown in Figure (a,b). Figure (a) result clearly shows that the CR declines while increasing TAM’s concentration, and consequently,
increases with inhibitor concentrations (Figure (b)). This observation indicates the effectiveness of the expired drug in the protection of St52 steel in 1 M HCl because of enhanced surface coverage and TAM’s adsorption on the St52 steel surface. This adsorption of TAM molecules on the St52 steel surface reduces the available surface area for corrosion.
3.1.2. Temperature effect and thermodynamics of corrosion process
The and
values deduced from gravimetric measurement without and with optimum concentration (2.0 × 10−3 M) of TAM at various temperatures are given in Table . It shows clearly from the table that
of St52 steel in both absence and presence of TAM increased with temperature rise while
decreased with temperature rise. This could be because the inhibitor molecules get desorbed at a higher temperature from the metal surface [Citation32]. The dependency of CR on temperature is given by Arrhenius and Eyring’s equations (Equations 11 and 12), from which the activation energy and some thermodynamics parameters were deduced [Citation27].
(11)
(11)
(12)
(12) where
stands for pre-exponential factor;
, activation energy;
, gas constant;
, absolute temperature;
stands for Avogadro’s number;
represents Plank’s constant;
represents activation entropy; and
the activation enthalpy. Arrhenius plot (
versus
) and Eyring’s plot (
versus
) are shown in Figures and . Linear curves were gotten with slope
for Arrhenius plot and
for transition state plot, from where
and
were respectively obtained and given in Table . The values of
given in Table were calculated from the intercept of
from Figure . The
in the absence of TAM is 31.32 kJ/mol whereas in the presence of different concentrations of TAM, the values ranged from 36.71 to 45.92 kJ/mol. The higher
values in inhibitor presence when compared with its absence show the difficulty in the dissolution of St52 steel in the presence of TAM due to TAM molecules’ adsorption on the St52 steel surface [Citation32,Citation33]. The calculated
values are positive and follow the pattern of
, which indicates the endothermic character of the St52 steel corrosion and the slow corrosion of the steel in TAM’s presence. The negative values of activation entropy (
) both with and without TAM indicate that activated complexes in the rate deciding step address dissociation, suggesting a diminishing in disorderliness on proceeding from reactants to activated complex [Citation34].
Figure 3. Plot of versus
for St52 steel corrosion in 1M HCl and inhibited solutions at various concentrations of TAM.
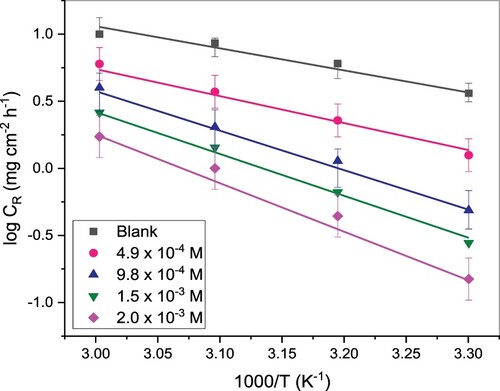
Figure 4. Plot of versus
for corrosion of St52 steel in 1 M HCl and solution and inhibited solutions at different concentrations of TAM.
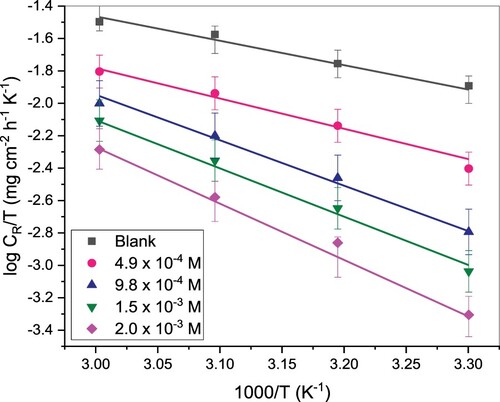
Table 2. Computed values and thermodynamics parameters for St52 steel corrosion in 1 M HCl solution without and with various TAM concentrations.
3.2. EIS measurements
EIS measurements were undertaken to better understand the electrochemical processes that occur at the surface of the corroding steel without and with the addition of TAM. The impedance spectra of St52 steel presented as Nyquist diagrams for TAM in 1M HCl solution without and with low and highest concentrations of the inhibitor are displayed in Figure (a). The spectra possess a single depressed semicircle, indicating the existence of a charge-transfer corrosion process that is not affected by the introduction of TAM. However, the semicircle’s diameter increased with TAM’s concentration, suggesting that the presence of TAM increased resistivity towards charge-transfer at the steel/1M HCl interface and resulted in a protective film of TAM on the St52 steel surface [Citation22,Citation35,Citation36]. Furthermore, the EIS spectra are imperfect semicircles, which show the frequency scattering consequent to the inhomogeneity or roughness of the steel surface [Citation37,Citation38]. More so, phase angle and are displayed for higher values by the higher concentration than the lower concentration (Figure (b,c)). This observation is reflective of better inhibition performance of higher concentration than lower concentration. The inhibition phenomenon could be explained further on the premise that more TAM molecules were adsorbed and a larger surface area covered upon the addition of a higher concentration of TAM to the corrosive media. The EIS data were fitted with an equivalent circuit depicted in Figure (d). In the model,
is the solution resistance and
represents charge-transfer resistance while CPE stands for constant phase element. The use of CPE in place of the pure capacitor is due to the non-ideal activity of steel surfaces and the CPE will give a better fit of the impedance data. The CPE impedance
is computed using the following equation [Citation27,Citation39]:
(13)
(13)
Figure 5. (a) Nyquist, (b) phase angle and (c) Bode modulus plots of St52 steel in 1M HCl without and in different concentrations of TAM.
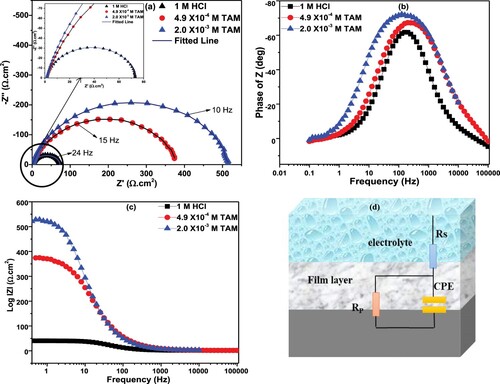
where is the CPE magnitude,
represents angular frequency,
= −1 is an imaginary number and
, related to phase shift, is the CPE exponent which gives information on the unevenness of the steel surface. The double-layer capacitance (
) values were deduced from the CPE parameter
and
using the expression [Citation40]:
(14)
(14) where
is the angular frequency (rad s−1) at maximum imaginary impedance. The impedance parameters including
,
,
,
,
and
are presented in Table . The
values were seen to decrease while the
values and
increase with the increase in TAM concentration.
decrease could be attributed to decreased dielectric constant coupled with increased electric double-layer thickness while
increase could be due to surface coverage increase which propels an inhibition efficiency increase [Citation41,Citation42].
Table 3. Impedance data for St52 steel in 1M HCl and with various concentrations TAM at 303 K.
3.3. Open circuit potential/ polarization measurement
Figure (a) shows the variation of Eocp with respect to time for St52 steel in 1 M HCl in the absence and presence of TAM. It was observed that the blank solution progressed in a positive direction until a steady-state process was attained. The observed phenomenon is attributed to the corrosion process of pre-exposure, air-formed oxide layer and surface dissolution of the steel. Compared to the Eocp vs time plot of the blank, the Eocp in the inhibited systems is shifted in the anodic direction, which indicates adsorption of TAM molecules on the anodic sites of the steel.
Figure 6. (a) Open circuit potential and (b) polarization curves of St52 steel in1 M HCl and in the presence of different TAM concentrations.
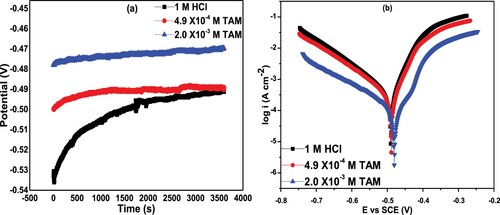
Furthermore, polarization curves of St52 steel in 1 M HCl without and with various concentrations of TAM at 303 K are shown in Figure (b). The polarization parameters like , corrosion potential (
), anodic and cathodic Tafel slopes (
) and inhibition efficiency (
) deduced by extrapolation of the cathodic and anodic current potential curves are given in Table . Figure and Table clearly show that
decreased with increase TAM concentration, implying the suppression of both anodic St52 steel dissolution and cathodic H2 evolution. Table clearly shows that
values decreased from 182.4 µA cm−2 for 1 M HCl without TAM to 26.2 µA cm−2 in the presence of 4.9 × 10−4 M of TAM and continually reduces to 10.8 µA cm−2 as the inhibitor concentration is increased gradually to 2.0 × 10−3 M. This also leads to a significant increase in
as the concentration of TAM increases and reaches a maximum of 94.1% at maximum inhibitor concentration. Again, the
is seen to shift towards a positive direction, suggesting that TAM effectively retarded the dissolution of St52 steel [Citation43]. Based on the maximum shift in
values which is less than 85 mV, the inhibitor TAM drug in the present study behaves as a mixed-type inhibitor [Citation44]. The polarization and EIS techniques complement each other with their results being in good agreement.
Figure 7. SEM/EDX images of St52 steel surface in (a) 1 M HCl without and (b) with 2.0 × 10−3 M of TAM.
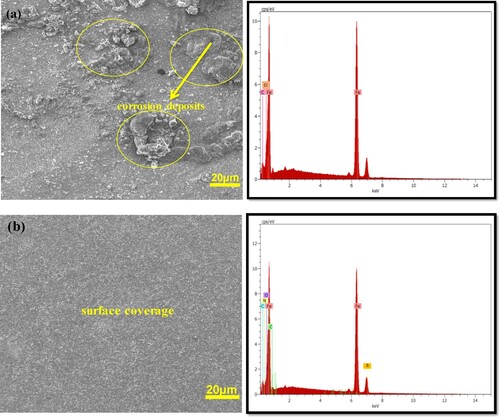
Table 4. Polarization data for St52 steel corrosion in 1.5 M HCl in the absence and presence of TAM.
3.4. SEM/EDX studies
The morphological analysis using SEM/EDX gives useful qualitative evidence about the extent of corrosion attack and adsorption of inhibitor molecules on the steel surface. Figure (a) shows the SEM image of corroded St52 steel surface in 1 M HCl without TAM while Figure (b) represents the SEM image in the presence of 2.0 × 10−3 M of TAM with the corresponding EDX spectrum. The image without TAM reveals a steel surface that is greatly damaged with visible corrosion deposits and cracks. These observations were caused by the aggressive attack of the studied electrolyte (1 M HCl) on the St52 steel surface. On the contrary, the SEM image in the presence of TAM presents a smoother surface that appears less corroded, suggesting adsorption and protective film formation by the molecules of TAM on the steel/acid solution interface [Citation45]. The EDX also shows the adsorption of expected compounds on the steel surface which proves good inhibition action of TAM molecules.
3.5. Adsorption isotherm
To ascertain the best-fitted adsorption isotherm for TAM’s adsorption on the corroding St52 steel surface, various isotherms such as Temkin, Langmuir, Frumkin and Freundlich were tested. The Langmuir isotherm was best fitted for all the tested isotherms and is expressed as follows [Citation27]:
(15)
(15)
where is TAM concentration,
represents surface coverage, and
represents adsorption equilibrium constant. The Langmuir isotherm plot for TAM is shown in Figure . The regression coefficients (
) and the slope of the isotherm are listed in Table . The results clearly show that the
and slope values are very near to unity, implying that TAM’s adsorption obeys Langmuir isotherm. The little drifting of the slopes from one is due to mutual attraction or repulsion between the inhibitor molecules resulting from intermolecular interaction between the adsorbed TAM molecules. From the intercept of the linear graph,
values were deduced. The
is known to represent the adsorption or desorption strength between the metal surface and the adsorbate. Large
values are consistent with effective inhibitor adsorption [Citation46]. The
is related adsorption free energy (
) as:
(16)
(16)
Figure 8. Langmuir isotherm plots for TAM’s adsorption on St52 steel surface at various temperatures.
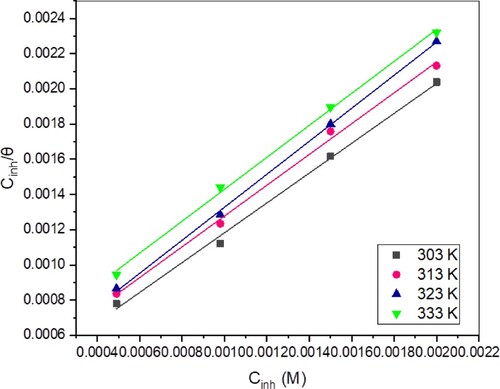
Table 5. Langmuir adsorption isotherm data for TAM at various temperatures.
where stands for temperature,
represents gas constant and 55.5 is
concentration (M) in solution. The computed
and
values are displayed in Table . Negative
suggest that the adsorption of TAM on the St52 steel surface is self-generated and with stable adsorbed film. Literature of previous studies indicates that values of
within −40 kJ mol−1 or negatively higher are consistent with chemisorption [Citation47]. On the other hand, values of
around −20 kJ mol−1 are consisted with physisorption. The values of
as shown in Table from −30.27 to −32.04 kJ mol−1 indicate that the adsorption of TAM on the St52 steel surface is competitive of chemisorption and physisorption [Citation47].
3.6. Proposed inhibition mechanism
The corrosion inhibition mechanism of organic compounds based on literature reports and our present study is generally due to adsorption. TAM displayed a value comprising chemisorption and physisorption mechanism. Chemisorption involves the donor–acceptor interaction between vacant d-orbitals of iron and lone pairs of electrons of heteroatoms together with
electrons of multiple bonds [Citation48]. Physisorption involves the existence of both electrically charged steel surface and charged molecules of the inhibitor. The inhibition mechanism of TAM will depend greatly on its molecular structure and the functional groups present. The molecules of TAM in the acidic solution can be protonated, and the resulting cations exist in equilibrium with its molecular form:
The protonation of amine could improve the solubility of TAM molecules in the aggressive acid solution and the chloride ion, Cl− (counter anion) will enhance the inhibitor adsorption [Citation49]. The protonated amine group will interact with the adsorbed Cl− on the St52 steel surface through electrostatic interaction. The various spatial adsorption interactions of TAM on the St52 steel surface are illustrated in Figure . The molecules of TAM can also adsorb through the interaction of Fe atom vacant d-orbital with -electrons of TAM molecules and non-bonded electrons of heteroatoms [Citation50]. There is also a possibility of TAM forming a smooth homogenous monolayer of protection on the St52 steel surface as shown in Figure . This leads to high surface coverage and effective adsorption [Citation51].
3.7. Theoretical calculations
To have a better understanding of the donor–acceptor interactions between surface iron atoms and the frontiers molecular orbital (FMO) of TAM, quantum chemical calculations were performed. According to the FMO theory of chemical reactivity, the interaction between the LUMO and HOMO of the reacting species results in transition formation [Citation52]. The HOMO energy () is related to the capacity of the molecule to give electrons to the available metal surface vacant d-orbital. Therefore, higher
leads to better adsorption. Then, again, the energy of LUMO (
) provides information on electron acceptance by the molecule. Decreased
shows the capability of TAM molecules to receive an electron from the steel surface and increase inhibition efficiency. Reports have shown that effective inhibitors are those that will not only donate an electron to the corroding metal but can also accept an electron from the metal. The energy gap
is a measure of the reactivity or efficacy of the inhibitor, and small
values suggest good inhibition efficiency of the molecule [Citation53].
The LUMO and HOMO surfaces of the neutral (TAM) and protonated (TAM-H+) species of the inhibitor are displayed in Figure . It is clear that the LUMO and HOMO orbitals are separately distributed on amino group, phenyl ring and oxygen atoms both in protonated and in species non-protonated. However, the LUMO orbital of TAM-H+ involved sulphur atoms as well. This indicates that the oxygen atoms, nitrogen, sulphur atoms and phenyl rings are among the active adsorption centres. The quantum chemical parameters computed from the DFT are shown in Table . From the table, the values of suggest TAM to be superior over TAM-H+ while values of
suggest TAM-H+ is superior. However, the
which probably is the most important DFT reactivity parameter supports the superior effect of TAM-H+ over TAM. The smaller value of the electronegativity (
) of TAM indicates its higher reactivity and higher ability over TAM-H+ in donating electrons to the metal surface. The total energy of TAM-H+ was more negative than that of TAM, suggesting molecular stability of TAM-H+ when interacting with the St52 steel. Another computed parameter, the dipole moment (
) which shows molecular polarity, was found to be higher in TAM-H+ than in TAM. There are conflicting reports in literature on the influence of
on corrosion inhibition. While some authors suggest that a decrease in
leads to an increase in the efficacy of the inhibitor, others rather think the reverse is the case [Citation54]. Low hardness (
) value for an organic molecule is indicative of an efficient corrosion inhibitor while a higher value of
indicates a less potent inhibitor [Citation55]. The inverse of harness (
) is known as softness (
), and it has a reverse effect on corrosion inhibition with the
values. The values of
and
from the present study also support the superiority of TAM-H+ over TAM. The fraction of electron transfer (
) is a measure of the extent of metal–inhibitor interactions, and studies have shown that values of
greater than 0 and less than 3.6; that is,
indicates increasing inhibition efficiency with an increase in the electron-donating capability of the inhibitor at the steel surface [Citation55]. More so, the computed value of
in the present study is
3.6 for both pronated and neutral species of TAM which confirms the capability of the inhibitor to donate an electron to the steel surface and inhibitive effect of the studied inhibitor.
Table 6. DFT parameters for neutral (TAM) and protonated (TAM-H+) form of the inhibitor.
4. Conclusions
The present study considers the evaluation of the performance of expired tamsulosin (TAM) as a corrosion inhibitor for St52 steel in a 1.5 M HCl medium. The studied drug was found to inhibit the corrosion of the steel in the aggressive acid solution with an increase in its efficiency as TAM concentration increases. Gravimetric studies reveal that TAM’s efficiency decreases with temperature rise. EIS measurement indicates that the presence of TAM in the aggressive acid medium significantly increased due to TAM’s adsorption at the metal–electrolyte interface. PDP studies disclose that TAM is a mixed-type corrosion inhibition. SEM image in the presence of TAM reveals a smoother surface that appears less corroded, suggesting adsorption and protective film formation by the molecules of TAM on the steel/acid solution interface. The inhibitor followed Langmuir isotherm and
values suggest competitive chemical and physical adsorption mechanism. Theoretical calculations support the corrosion inhibitive effect of the inhibitor.
Acknowledgments
Council of Scientific and Industrial Research (CSIR), India, and The World Academy of Sciences (TWAS), Italy, are gratefully acknowledged by Valentine Chikaodili Anadebe for the CSIR-TWAS Postgraduate Fellowship (Award No.22/FF/CSIR-TWAS/2019) to pursue his Ph.D. research programme in CSIR-CECRI, India. In addition, V.C.A is grateful to Alex Ekwueme Federal University Ndufu-Alike Ebonyi State, Nigeria, for the research leave to visit CECRI, India.
Disclosure statement
No potential conflict of interest was reported by the author(s).
References
- Soltani N, Behpour M, Oguzie EE, et al. Corrosion inhibition of mild steel by an imidazolium ionic liquid compound: the effect of pH and surface pre-corrosion. RSC Adv. 2015;5:11145–11162.
- Tazouti A, Galai M, Touir R, et al. Experimental and theoretical studies for mild steel corrosion inhibition in 1.0 M HCl by three new quinoxalinone derivatives. J. Mol. Liq. 2016;221:815–832.
- Abdallah M, Sobhi M, Altass HM. Corrosion inhibition of aluminum in hydrochloric acid by pyrazinamide derivatives. J. Mol. Liq. 2016;223:1143–1150.
- Raviprabha K, Bhat RS. 5-(3-Pryridyl)-4H-1,2,4-triazole-3-thiol as potential corrosion inhibitor for AA6061 Aluminium alloy in 0.1 M Hydrochloric Acid Solution. Surf Eng Appl Electrochem. 2019;55:723–733.
- Kabel KI, Zakaria K, Abbas MA, et al. Assessment of corrosion inhibitive behavior of 2-aminothiophenol derivatives on carbon steel in 1 M HCl. J. Ind. Eng. Chem. 2015;23:57–66.
- Obot IB, Madhankumar A, Umoren SA, et al. Surface protection of mild steel using benzimidazole derivatives: experimental and theoretical approach. J. Adhes Sci Technol. 2015;29:2130–2152.
- Iroha NB, Oguzie EE, Onuoha GN, et al. Inhibition of mild steel corrosion in acidic solution by derivatives of diphenyl glyoxal). 16th international corrosion congress; Beijing, China. 2005
- Ansari KR, Quraishi MA, Singh A. Isatin derivatives as a non-toxic corrosion inhibitor for mild steel in 20% H2SO4. Corros Sci. 2015;95:62–70.
- Verma C, Olasunkanmi LO, Obot IB, et al. 2,4-Diamino-5-(phenylthio)-5H-chromeno [2,3-b] pyridine-3-carbonitriles as green and effective corrosion inhibitors: gravimetric, electrochemical, surface morphology and theoretical studies. RSC Adv. 2016;6:53933–53948.
- Kadhum A, Mohamad AB, Hammed L, et al. Inhibition of mild steel corrosion in Hydrochloric Acid Solution by new coumarin. Materials. 2014;7:4335–4348.
- Abdallah M, Gad EAM, Sobhi M, et al. Performance of tramadol drug as a safe inhibitor for aluminum corrosion in 1.0 M HCl solution and understanding mechanism of inhibition using DFT. Egypt. J. Pet. 2019;28:173–181.
- Ayoola AA, Fayomi OSI, Ogunkanmbi SO. Data on inhibitive performance of chloraphenicol drug on A315 mild steel in acidic medium. Data Br. 2018;19:804–809.
- EL-Haddad ME, Fouda AS, Hassan AF. Data from chemical, electrochemical and quantum chemical studies for interaction between Cephapirin drug as an eco-friendly corrosion inhibitor and carbon steel surface in acidic medium. Chem. Data Collect. 2019;22:100251.
- Singh P, Chauhan DS, Chauhan SS, et al. Chemically modified expired Dapsone drug as environmentally benign corrosion inhibitor for mild steel in sulphuric acid useful for industrial pickling process. J Mol Liq. 2019;286:110903.
- Srivastava M, Tiwari P, Srivastava SK, et al. Electrochemical investigation of Irbesartan drug molecules as an inhibitor of mild steel corrosion in 1M HCl and 0.5 M H2SO4 solutions. J Mol. Liq. 2017;236:184–197.
- Anadebe VC, Onukwuli OD, Abeng FE, et al. Electrochemical-kinetics, MD-simulation and multi-input single-output (MISO) modeling using adaptive neuro-fuzzy inference system (ANFIS) prediction for dexamethasone drug as eco-friendly corrosion inhibitor for mild steel in 2M HCl electrolyte. J Taiwan Inst. Chem. Eng. 2020;115:251–265.
- Hameed RS A. Expired Ranitidine drugs as corrosion inhibitor for aluminum in 1M HCl. Al-Azhar Bull Sci. 2009;20:151–163.
- Naqvi I, Saleemi AR, Naveed S. Cefixime: A drug as efficient corrosion inhibitor for mild steel in Acidic Media: electrochemical and thermodynamic studies. Int J Electrochem Sci. 2011;6:146–161.
- Singh AK, Quraishi MA. Effect of Cefazolin on the corrosion of mild steel in HCl solution. Corros. Sci. 2010;52:152–160.
- Ebenso EE, Eddy NO, Odiongenyi AO. Corrosion inhibition and adsorption properties of Methocarbamol on mild steel in acidic medium. Port. Electrochim. Acta. 2009;27:13–22.
- Fouda AS, El Morsi MA, El Mogy T. Studies on the inhibition of carbon steel corrosion in hydrochloric acid solution by expired carvedilol drug. Green Chem Lett Rev. 2017;10:336–345.
- Iroha NB, Ukpe RA. Investigation of the inhibition of the corrosion of carbon steel in solution of HCl by glimepiride. Commun Phys Sci. 2020;5:246–256.
- Fouda AS, El Morsi MA, El-Mogy T. Investigation of the inhibition of carbon steel corrosion in Hydrochloric Acid Solutions by domperidone drug. J Anal Pharm Res. 2017;5(5):00153.
- Dahiya S, Saini N, Dahiya N, et al. Corrosion inhibition activity of an expired antibacterial drug in Acidic Media amid elucidate DFT and MD simulations port. Electrochim Acta. 2018;36:213–230.
- Wang RC, Smith-Bindman R, Whitaker E, et al. Effect of Tamsulosin on stone passage for ureteral stones: a systematic review and meta-analysis. Ann Emerg Med. 2016;69(3):353–361.
- Gupta NK, Gopal CSA, Srivastava V, et al. Application of expired drugs in corrosion inhibition of mild steel. Int J Pharm Chem Anal. 2017;4:8–12.
- Iroha NB, Akaranta O. Experimental and surface morphological study of corrosion inhibition of N80 carbon steel in HCl stimulated acidizing solution using gum exudate from terminalia mentaly. SN Appl Sci. 2020;2:1514.
- Xia G, Jiang X, Zhou L, et al. Synergic effect of methyl acrylate and N-cetylpyridinium bromide in N-cetyl-3-(2-methoxy carbonylvinyl) pyridinium bromide molecule for X70 steel protection. Corros. Sci. 2015;94:224–236.
- Iroha NB, Madueke NA, Mkpenie V, et al. Experimental, adsorption, quantum chemical and molecular dynamics simulation studies on the corrosion inhibition performance of vincamine on J55 steel in acidic medium. J Mol. Struct. 2021;1227:129533.
- Yadav M, Kumar S, Purkait T, et al. Electrochemical, thermodynamic and quantum chemical studies of synthesized benzimidazole derivatives as corrosion inhibitors for N80 steel in hydrochloric acid. J Mol Liq. 2016;213:122–138.
- Verma C, Sorour AA, Ebenso EE, et al. Inhibition performance of three naphthyridine derivatives for mild steel corrosion in 1M HCl: computation and experimental analyses. Results Phys. 2018;10:504–511.
- Geethamani P, Narmatha M, Dhanalakshmi R, et al. Corrosion inhibition and adsorption properties of mild steel in 1 M hydrochloric acid medium by expired ambroxol drug. J Bio- Tribo-Corros. 2019;5:16.
- Tong Z. X, Wang R J, Huang J, et al. Hierarchical self-assembly, photo-responsive phase behavior and variable tensile property of azobenzene-containing ABA triblock copolymers. RSC Adv. 2015;5:4030–4040.
- Essien EA, Kavaz D, Ituen EB, et al. Synthesis, characterization and anticorrosion property of olive leaves extract-titanium. J Adhes Sci Technol. 2018;32(16):1773–1794.
- James AO, Iroha NB. An investigation on the inhibitory action of modified almond extract on the corrosion of Q235 mild steel in acid environment. IOSR J Appl Chem. 2019;12:1–10.
- Ansari KR, Quraishi MA. Experimental and quantum chemical evaluation of dihydropyridine derivative as environmental benign corrosion inhibitor for mild steel in 15% HCl. Anal Bioanal Electrochem. 2015;7:509–522.
- Iroha NB, Nnanna LA. Leucas Martinicensis as an inhibitor of carbon steel corrosion in acidic medium. Int J Res. 2020;7:19–26.
- Bostan R, Varvara S, Gaina L, et al. Evaluation of some phenothiazine derivatives as corrosion inhibitors for bronze in weakly acidic solution. Corros Sci. 2012;63:275–286.
- Roy P, Karfa P, Adhikari U, et al. Corrosion inhibition of mild steel in acidic medium by polyacrylamide grafted guar gum with various grafting percentage: effect of intramolecular synergism. Corros Sci. 2014;88:246–253.
- Alaoui K, El Kacimi Y, Galai M, et al. New triazepine carboxylate derivatives: correlation between corrosion inhibition property and chemical structure. Int J Ind Chem. 2020;11:23–42.
- Iroha NB, Maduelosi NJ. Stem extract of Dissotis theifolia (Melastomataceae) as a green corrosion inhibitor for N80 steel in 1 M HCl solution. IOSR J Appl Chem. 2020;13:30–38.
- Doner A, Sahin EA, Kardas G, et al. Investigation of corrosion inhibition effect of 3-[(2-hydroxy-benzylidene)-amino]-2-thioxo-thiazolidin-4-one on corrosion of mild steel in the acidic medium. Corros Sci. 2013;66:278–284.
- Abeng FE, Anadebe VC, Idim VD, et al. Anti-corrosion behavior of expired tobramycin drug on carbon steel in acidic medium. S. Afr. J. Chem. 2020;73:125–130.
- Abeng FE, Ikpi ME, Ushie OA, et al. Insight into the corrosion inhibition mechanism of carbon steel in 2 M HCl electrolyte by eco-friendly based pharmaceutical drugs. Chem Data Collect. 2021;34:100722.
- Popova A, Christov M. Inhibitive properties of quaternary ammonium bromides of Ncontaining heterocycles on acid mild steel corrosion. J Univ Chem Technol Metall. 2008;43:37–47.
- Amin MA. Ibrahim MM corrosion and corrosion control of mild steel in concentrated H2SO4 solutions by a newly synthesized glycine derivative. Corros Sci. 2011;53:873–885.
- Saha SK, Dutta A, Ghosh P, et al. Novel Schiff-base molecules as efficient corrosion inhibitors for mild steel surface in 1 M HCl medium: experimental and theoretical approach. Phys Chem Chem Phys. 2016;18:17898–17911.
- Asadi N, Ramezanzadeh M, Bahlakeh G, et al. Utilizing lemon balm extract as an effective green corrosion inhibitor for mild steel in 1M HCl solution: A detailed experimental, molecular dynamics. Monte Carlo and Quantum Mechanics Study. J Taiwan Inst Chem Eng. 2019;95:252–272.
- Faiz M, Zahari A, Awang K, et al. Corrosion inhibition on mild steel in 1 M HCl solution by cryptocarya nigra extracts and three of its constituents (alkaloids). RSC Adv. 2020;10:6547–6562.
- Sulaiman KO, Onawole AT, Faye O, et al. Understanding the corrosion inhibition of mild steel by selected green compounds using chemical quantum based assessments and molecular dynamics simulations. J Mol Liq. 2019;279:342–350.
- Gece G. Bilgiç S quantum chemical study of some cyclic nitrogen compounds as corrosion inhibitors of steel in NaCl media. Corros Sci. 2009;51:1876–1878.
- Ammal PR, Anupama RP, Joseph A. Comparative studies on the electrochemical and physicochemical behaviour of three different benzimidazole motifs as corrosion inhibitor for mild steel in hydrochloric acid. Egypt J Petrol. 2018;27:1067–1076.
- Guo L, Dong W, Zhang S. Theoretical challenges in understanding the inhibition mechanism of copper corrosion in acid media in the presence of three triazole derivatives. RSC Adv. 2014;4:41956–41967.
- Madkour LH, Kaya S, Kaya C, et al. Quantum chemical calculations, molecular dynamics simulation and experimental studies of using some azo dyes as corrosion inhibitors for iron. part 1: mono-azo dye derivatives. J Taiwan Inst Chem. 2016;68:461–480.
- Pavithra MK, Venkatesha TV, Kumar MKP, et al. Investigation of the inhibition effect of ibuprofen triazole against mild steel corrosion in an acidic environment. Res Chem Intermed. 2014;41:7163–7177.