Abstract
The cyclic voltammetry method was preliminarily used to study the reversibility nature of the electrochemical behaviour of budesonide (BDN) compound. The square wave-adsorptive stripping voltammetry (SW-AdSV) technique was applied to evaluate an electrochemical reduction of BDN in the phosphate buffer pH 2.5. The ultra-trace graphite working electrode was used to accumulate BDN onto its surface. An SW-AdSV reduction peak was observed in the potential of −0.98 V using Ag/AgCl reference electrode and Pt auxiliary electrode. Different parameters ,such as pH, buffer solutions, accumulations time and potential, frequency, amplitude and scan were investigated, and convection rates parameters were studied to acquire a high reduction response. The repeatability, stability, recovery, calibration curve and limit of detection and quantification parameters were studied to evaluate the analytical performance of the SW-AdSV technique. Repeatability and stability were monitored for 20 µM of BDN to give a 0.29% relative standard deviation (RSD%) that was achieved for five measurements and a very stable current for 120 min. The linearity was evaluated by studying the calibration curve over the range of 2 –25 µM (n = 8), given a linear relation between BDN concentrations and SW-AdSV signals. The limits of detection (LOD) and quantification (LOQ) were calculated to become 40 nM (0.017 ppm) and 0.134 µM (0.0577 ppm), respectively. The developed and validated method was successfully applied to the electrochemical study of BDN in pharmaceuticals and biological fluids.
1. Introduction
An adsorption operation is considered the mechanism for the pre-concentration of the studied analyte on the working electrode surface. For the stripping step, square wave voltammetry can be used. The sensitivity and selectivity of the analytical determination would be improved when the AdSV method is coupled with the SWV method as introduced in this research (SW-AdSV).
Square wave-adsorptive stripping voltammetry (SW-AdSV) is one of the fastest and highest sensitive voltammetric techniques. An important advance in the experimental technique (SW-AdSV) for the analysis of drugs and other compounds is due to its simplicity, low cost and relatively short analysis time compared with other analytical techniques. Based on that, the SW-AdSV method has proved to be very sensitive for the determination of pharmaceutical compounds [Citation1–4], pesticides [Citation5,Citation6], creatine [Citation7], metals [Citation8–11] and dyes [Citation12–16]. Budesonide (BDN) belongs to the glucocorticoid molecules (also called a steroid) with a strong anti-inflammatory and anti-allergic activity. It is used to prevent and treat chronic obstructive pulmonary and bronchial asthma diseases [Citation17]. It is given by metered-dose inhaler or nebulizer for prophylactic treatment of asthma, including patients who require oral corticosteroids and those who may benefit from a systemic dose reduction. Budesonide is chemically known as 11,21-dihydroxy-16α,17α-(butylidenebis(oxy))pregna-1,4-diene-3,20-dione[Citation18,Citation19].
It was determined by several analytical methods such as chromatography [Citation17,Citation20–25], liquid chromatography-tandem mass spectrometry (LC-MS/MS) [Citation26], spectrophotometry [Citation27–29] and micellar electrokinetic capillary chromatography [Citation30]
The research aims to develop and validate a simple method for the quantitative determination of BDN in inhalation suspension pharmaceutical doses and human fluids by the selective, sensitive and rapid SW-AdSV technique. The SW-AdSV was used to detect the trace levels of BDN drug by its accumulation on the surface of the UT graphite electrode. Finally, no polarographic and voltammetric methods were previously reported for the determination of BDN, so it determines using the square wave-adsorptive stripping voltammetry method as presented in this research. BDN content is commercially obtained by two different oral formulations that include nasal spray and inhalation powder. The scientific novelty of the present work is that the proposed SW-AdSV method is simple, selective, high sensitivity, low detection limit, less expensive and less time-consuming compared with other published methods and could be used for routine analysis. In addition, the SW-AdSV method was validated by comparing the results with cyclic voltammetry (CV) using a statistical F-test to verify precision and accuracy.
2. Experimental
2.1. Instruments
797 VA instrument (Switzerland made) was used to apply the SW-AdSV and CV techniques for the quantitative determination of budesonide (BDN). It was connected with three electrodes system that included a silver/silver chloride (3.0M KCl) reference electrode, UT graphite working electrode (2.0 mm surface area), and Pt auxiliary electrode. A pH-meter model 211 was used to measure pH values in the buffer solutions. The distilled water was obtained by a Millie-Q Plus instrument (USA- made). The biological fluids, spiked human urine and plasma were centrifuged by Labofuge 200 instrument (Germany-made).
2.2. Reagents
Budesonide (BDN) standard material powder was obtained from ACROS, ORGANICS, New Jersey- USA. A stock solution of 10-mM BDN was prepared in a 100-mL volumetric flask by dissolving BDN suitable mass in methanol solvent. For further experiments, the methanol solvent was used to prepare the diluted concentrations of BDN from the stock solution. The phosphate, carbonate, Britton- Robinson (B-R) and acetate buffer solutions were chemically prepared using phosphoric, acetic, boric acids, sodium carbonate and other compounds[Citation31] to study the electrochemical behaviour of the budesonide compound.
2.3. Procedure
The used electrochemical cell was neatly cleaned using distilled H2O and ethanol, and then it was used to inject a buffer solution of 10 mL throughout the analysis period. The SW-AdSV reduction was studied by application of the potential over the range of −0.5 to −1.5 V. The solution was shaken and purged by N2 gas for 120 s to take out O2 gas before each voltammetric measurement. The high sensitivity of electrochemical reduction (SW-AdSV) for analysis of BDN drug was achieved under the optimum parameters of phosphate buffer pH 2.5, 0.0 V Eacc, 30 s tacc, 20 Hz frequency, the scan rate of 200 mVs−1, the amplitude of 50 mV and 2000 rpm convection rate.
2.3.1. Preparation of human biological fluids
Firstly, 20 µM of budesonide was injected into 0.50 mL of human urine and plasma, respectively. Subsequently, 1.0 mL of ethanol, 1.0 mL of ZnSO4 5%. (7H2O) and 0.1 mL of NaOH were added to the biological solution in a centrifuge tube [Citation32]. Herein, the centrifuge instrument was used to centrifuge the mixture at 5000 rpm for 8 min. After that, the resulting solution was filtered to inject the filtrate inside the electrochemical cell to directly validate the SW-AdSV technique to determine the BDN content in the biological fluids by recovery. The SW-AdSV approach was applied to record the five measurements for each reduction scanning of the BDN compound.
2.3.2. Preparation of budesonide doses
The first commercial pharmaceutical is Rhinocort Aqa dose “budesonide” nasal spray (Solna, Sweden made). A 200 µL was directly injected into the electrochemical cell containing phosphate buffer pH 2.5 with the chosen parameters. The SW-AdSV method was applied for the BDN content in the pharmaceutical spray.
The second commercial pharmaceutical is Turbuhaler (budesonide and formoterol) dose “inhalation powder” (packed by Tabuk pharma., KSA, under the license from Astra Zeneca AB, Sweden). The commercial package contained 0.1520 g of inhalation powder that was wholly dissolved in 100 mL of methanol solvent. A 200 µL was directly injected into the electrochemical cell containing phosphate buffer, pH 2.5 and the selected optimum parameters. The SW-AdSV method was applied for the BDN content in the pharmaceutical inhalation powder.
3. Results and discussion
3.1. Cyclic voltammetric observation
The CV technique was carried out to initially evaluate the electrochemical voltammetry behaviour for the 100 µM of budesonide compound, as shown in Figure . The obtained electrochemical peak is probably due to the reduction of the carbonyl functional group to the hydroxyl functional group [Citation33], as proposed in Scheme 1 for the BDN formula. It also clarified the irreversibility nature of the reduction process of BDN. Consequently, the CV electrochemical behaviour confirmed the lack of anodic peak for BDN drug determination.
3.2. SW-AdSV approach
A clear reduction signal was observed at −0.98 V for 20 µM of BDN by using the SW-AdSV method. The UT graphite working electrode was used to study the electrochemical behaviour of the budesonide compound. The SW-AdSV behaviour of BDN was investigated by varying different buffer solutions, values of pH and other analytical conditions, to obtain a sensitive SW-AdSV signal corresponding to the reduction of the carbonyl functional group at −0.98 V potential. A representative cathodic SW-AdS voltammogram for 20 µM of BDN in phosphate buffer and pH 2.5 is illustrated in Figure . Therefore, the SW-AdSV response was indicated the ability of the graphite electrode to deposit the analysed BDN onto the UT graphite surface.
3.3. Study of analytical parameters
There are several parameters that can be affected the sensitivity and shape of the voltammetric peaks. These parameters included pH, buffer solutions, time and potential of accumulations, scan rate, amplitude, frequency and convection rate. Based on the tested runs, the high value of voltammetric currents was selected and reported in the present study.
3.3.1. Effect of buffer solutions and pH
Several buffer solutions have been prepared with various pH values, including acetate pH 3, B-R (Britton Robinson; pH 3, pH7 and pH10), carbonate pH 10 and phosphate pH 3, to determine 20 µM of BDN. Phosphate buffer solution at pH 2.5 has given a high value of voltammetric current, so it was selected for further experiments. In addition, the effect of pH ranging from 2.0 to 5.0 for phosphate buffer was studied to evaluate the electrochemical behaviour of BDN drug. The highest value of SW-AdSV current was achieved at 2.5 pH, as presented in Figure , so a pH value of 2.5 was selected for the following experiments.
On the other hand, the cathodic peak potential (Ep,c) was shifted gradually to more negative values starting from –950 to –1070 mV, by increasing the pH value over the range 2–5. From the relation of Ep,c vs. pH, the slope value was calculated to be 33.33 mV/pH, approximately close to the expected theoretical value of 30 mV/pH [Citation34]. This value indicates that Ep,c depended on pH values and showed the consumption of two protons (two electrons) in the electrochemical process as indicated in the proposed mechanism of BDN.
3.3.2. Effect of potential and time accumulations
The accumulations potential, Eacc, and time, tacc, parameters are considerable important factors affecting the Redox reactions behaviour. The Eacc and tacc were investigated in the range from −0.6 to +0.6 V, and 0.0–90 s (Figures and ), respectively, for 20µM of BDN in phosphate buffer with 2.5 pH. The highest cathodic response potential was achieved at 0.0 V and 30 s time of accumulation. These accumulation values were selected as optimum accumulation parameters for the following determination of BDN.
3.3.3. Effect of frequency and amplitude
The frequency and amplitude voltage step were known as very interesting parameters for studying the sensitivity of voltammetric peaks. Herein, the frequency and amplitude were studied over the range from 10 to 70 Hz and 10 to 70 mV (Figures and ), respectively, for 20 µM of BDN in phosphate buffer, pH 2.5, 0.0 V Eacc and 30 s tacc. The high voltammetric current was achieved by 20 Hz and 50 mV, so they were selected for the subsequent experiments.
3.3.4. Effect of scan and convection rates
The rates of scan and convection are also considered very important factors to obtain the highest voltammetric currents. The scan and convection rates were studied over the ranges from 50 to 300 mVs−1 and 0.0 to 3000 rpm, respectively, for 20 µM of BDN in phosphate buffer, pH 2.5, 0.0 V Eacc, 30 s tacc, 20 Hz and 50 mV amplitude. The high voltammetric readings were obtained at a scan rate of 200 mVs−1 and 2000 rpm convection rate (Figures and ). These optimized factors were selected for the following BDN experiment.
Figure 8. Effect of scan rate (mVs−1) on reduction current for 20 µM of BDN in phosphate buffer pH 2.5, 0.0 V Eacc, 30 s tacc, 20 Hz and 50 mV amplitude.
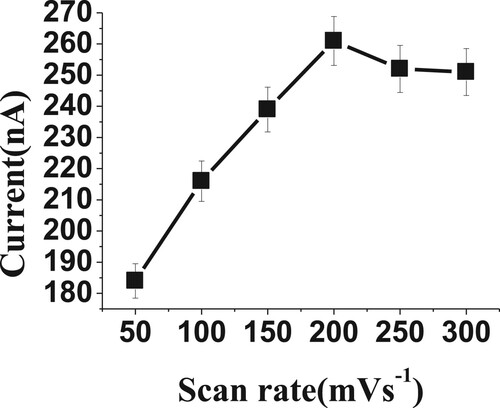
Figure 9. Effect of convection rate (rpm) on reduction current for 20 µM of BDN in phosphate buffer pH 2.5, 0.0 V Eacc, 30 s tacc, 20 Hz, 50 mV amplitude and 200 mVs−1.
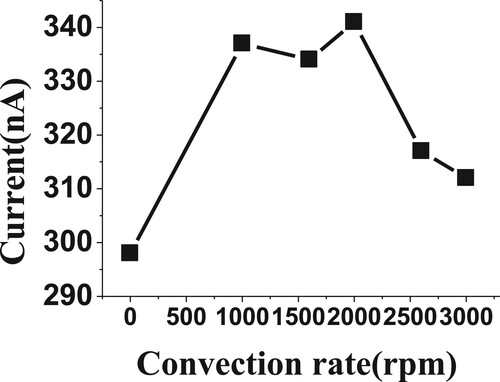
On the other hand, the relation of scan rate logarithm (logv) vs. current logarithm (logi) was given as 0.90 slope value that is closed to 1.0. This value has confirmed the accumulation of BDN onto the UT graphite surface controlled by adsorption [Citation35].
3.3.5. Effect of working electrodes
The working electrodes are a very important factor that affects the electrochemical response and obtains a sensitive SW-AdSV signal. Different electrodes of UT graphite, glassy carbon (GC), gold (Au), silver (Ag) and platinum (Pt) were used for the monitoring of SW-AdSV response for 20 µM of BDN compound. Au, Ag and Pt electrodes did not exhibit SW-AdSV signal at the E1/2 of BDN(−0.98 V), while the GC electrode was decreased in SW-AdSV signal by 50% compared with the graphite UT electrode. According to these results, the UT graphite electrode was selected for all BDN experimental procedures.
3.4. Validation of SW-AdSV method
The SW-AdSV technique was validated for the study of some parameters such as linearity (calibration curve), limits of detection and quantification, repeatability, stability, accuracy and interferences effect.
3.4.1. Linearity
The linearity (calibration curve) was studied over the BDN concentrations ranging from 2 to 25 µM (n = 8) under optimum parameters (Figures and ). The calibration graph of the SW-AdSV method was reported during the studied concentrations range to give 0.95 r2 correlation coefficient.
3.4.2. Limits of detection and quantification
The limits of detection and quantification (LOD, LOQ) were obtained and calculated from the calibration curve and repeatability data. The LOD and LOQ were estimated to become 40 nM (0.017 ppm) and 0.134 µM (0.0577 ppm), respectively. These LOD and LOQ values confirmed the high sensitivity of the SW-AdSV procedure for BDN determination comparing the HPLC method with values of 0.05 ppm LOD and 0.20 ppm LOQ [Citation20], respectively. While, the spectrophotometric methods achieved 0.666 and 0.461 ppm LOD and 2.06 and 1.397 ppm LOQ, respectively [Citation27,Citation28].
Table summarizes the linearity results, LOD and LOQ for the developed SW-AdSV, a reference method (CV) and these published articles.
Table 1. Analytical data for calibration curves, LOD and LOQ for BDN determination under optimum conditions using SW-AdSV, reference method (CV) and some published articles.
3.4.3. Repeatability and stability
Repeatability and stability are extremely important factors that affect the analytical performance of the SW-AdSV method. Herein, they were studied for 20 µM of BDN under the selected optimum conditions. The repeatability was reported for five cathodic measurements to have resulted in 0.29% (RSD%). While the stability was observed for 2 h gave a fully stable SW-AdSV signal for the studied drug.
3.4.4. Accuracy
The accuracy of the developed SW-AdSV method was evaluated using the calculation of the recovery of an unknown amount of BDN (10 µM) added to phosphate buffer pH 2.5 solution and analysed via the optimized SW-AdSV procedure. The value of the recovery obtained by the standard addition method is to be recorded as 111% ± 0.89.
Table summarizes the repeatability results for the developed SW-AdSV and CV reference method measurements of BDN drug and SW-AdSV accuracy (recovery).
Table 2. SW-AdSV and CV reference method repeatability results for BDN determination under optimum conditions and SW-AdSV recovery data.
3.4.5. Influence of interferences on SW-AdSV signal
The starch, sucrose and lactose substances and other materials are already packed inside the commercial pharmaceuticals. These substances may be considered as interferences that could compete with the studied drug on the surface of the working electrode (UT graphite). The 5, 10 and 25 folds of these interferences were added to the 20 µM BDN at phosphate buffer solution and pH 2.5 under optimum conditions. The SW-AdSV procedure was applied for these substances to study their effect on the cathodic current. Starch was negatively affected on the SW-AdSV signal of 20 µM BDN solution by 30.8%, 50% and 58%, respectively. In addition, lactose was negatively affected on the SW-AdSV signal of 20 µM BDN solution by 16%, 20.5% and 23%, respectively. Finally, sucrose also negatively affected the reduction signal of 20 µM BDN solution by 4.6%, 8.5% and 12%, respectively.
3.5. SW-AdSV application
The validation of the developed SW-AdSV technique was tested in the evaluation via the electrochemical analysis of budesonide compounds in commercial formulations and biological fluids.
3.5.1. Determination of BDN in pharmaceutical formulations
The SW-AdSV was directly applied to determine budesonide in its commercial doses. For each doses sample, a 200 µL was directly injected into the electrochemical cell that contained phosphate buffer of pH 2.5 under optimum parameters to give 310 and 232 nA cathodic currents at the BDN reduction potential (−0.98 V) for Rhinocort Aqa “nasal spray” and Turbuhaler “inhalation powder”, respectively, as shown in Figures and . After the needed dissolving and filtration procedures, the budesonide content of commercially available inhalation powder (Turbuhaler) was used for the SW-AdSV analysis. The SW-AdSV approach was evaluated by repeating the voltammetric scan five times for each doses samples, resulting 2.16 µM ± 0.185 and 6.6 µM ± 0.34 for nasal spray and inhalation powder, respectively.
Figure 12. Square wave-AdS voltammogram of 200 µL of commercial Rhinocort Aqa dose “nasal spray” sample using UT electrode under optimum conditions.
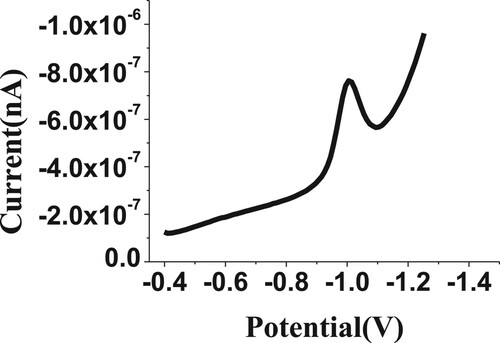
Figure 13. Square wave-AdS voltammograms of commercial Turbuhaler dose “0.1520 g of inhalation powder” sample using UT electrode under optimum conditions (A: in 100 mL MeOH, B: in 25 mL MeOH).
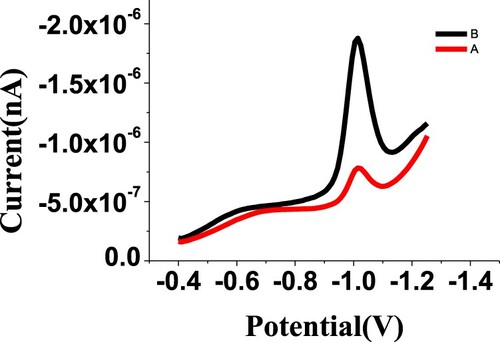
In this research, CV was used as a reference method to determine BDN in the same pharmaceutical doses.
Table summarizes the BDN results using the SW-AdSV method and the reference CV technique using least square equations.
Table 3. The validated and developed SW-AdSV results for the determination of BDN in pharmaceutical doses and by a reference method (CV).
As it seems from Table , the results achieved by the developed SW-AdSV technique were in good agreement with those obtained by the CV procedure to determine BDN in the same pharmaceutical formulations. According to the statistical evaluation (F-test approach) for these results, there is no significant difference between the results obtained by the developed SW-AdSV technique and obtained by the CV reference method. Comparing SW-AdSV with the reference method (CV) results, the calculated F-test values were 5.82 and 4.7 for nasal spray and inhalation powder samples, respectively. These F-test values were less than the critical value (6.59) at the 95% confidence level. There is no statistical evidence that the variance of the proposed SW-AdSV method differs significantly from the variance of the CV reference method.
Accordingly, there is no significant difference between these two methods (SW-AdSV and CV) in precision for the BDN determination.
3.5.2. Determination of BDN in biological fluids
The validation of the electrochemical method (SW-AdSV) was also successfully evaluated through the determination of BDN in the spiked biological fluids (urine and plasma) by recovery of 0.5 µM of BDN standard. The SW-AdSV procedure was carried out by standard addition method for BDN pharmaceutical in the biological samples (five measurements for each addition) by recovery of 20 µM using the addition of 0.5 µM of BDN five times, to give the recovery means of 113% and 104% with STD (standard deviations) of ±1.67% and ±1.26% for spiked urine and plasma solutions, respectively, as listed in Table .
Table 4. The validated and developed SW-AdSV results for determination of BDN in biological fluids.
4. Conclusion
The SW-AdSV method has been developed and validated to determine budesonide (BDN) in pharmaceutical formulations and human biological fluids (urine and plasma). Among the advantages of this method is the linear lower detection limit within a range of 2–25 µM (n = 8) under selected conditions. This study gives a very good feature of easy procedures to analyse the biological fluids and introduces many benefits for the validated SW-AdSV technique. In addition, the voltammetric results gave significant advantages that included rapid, high sensitivity and simplicity of the used SW-AdSV approach. The detection and quantification data limits also confirmed a high sensitivity of the SW-AdSV technique for BDN determination. The UT graphite electrode gave more advantages for the BDN analysis in pharmaceutical formulations and human samples. The CV method was used as a reference method for this BDN determination.
Acknowledgement
The author likes to thank A. Althafiry and Sameh, academic members in Taibah University, Medina –KSA, for their support to get the pharmaceuticals for completing this research.
Disclosure statement
No potential conflict of interest was reported by the author(s).
References
- Al-Ghamdi AF, Hefnawy MM, Al-Majed AA, et al. Development of square-wave adsorptive stripping voltammetric method for determination of acebutolol in pharmaceutical formulations and biological fluids. Chem Cent J. 2012;6:15.
- Stępniowska A, Sztanke M, Tuzimski T, et al. Square-wave adsorptive stripping voltammetric approaches at two in situ modified electrodes as first analytical methods for the quantitative determination of a new anticancer drug candidate. J Iran Chem Soc. 2019;16:2755–2763.
- Ardila JA, Oliveira GG, Medeiros RA, et al. Square-wave adsorptive stripping voltammetric determination of nanomolar levels of bezafibrate using a glassy carbon electrode modified with multi-walled carbon nanotubes within a dihexadecyl hydrogen phosphate film. Analyst. 2014;139:1762–1768.
- Levent A, Altun A, Süleyman Taş S, et al. Voltammetric behavior of testosterone on bismuth film electrode: highly sensitive determination in pharmaceuticals and human urine by square-wave adsorptive stripping voltammetry. Electroanal. 2015;27:1219–1228.
- Melo LC, Julião MSS, Milhome MAL, et al. Square wave adsorptive stripping voltammetry determination of chlorpyriphos in irrigation agricultural water. J Anal Chem. 2018;73:695–704.
- Guiberteau A, Galeano T, Mora N, et al. Study and determination of the pesticide imidacloprid by square wave adsorptive stripping voltammetry. Talanta. 2001;53:943–949.
- Stojanova K, Gulaboski R, Mirečeski V, et al. Adsorptive stripping square-wave voltammetry of creatine. Anal Let. 1999;32:2937–2950.
- Neto MM, Rocha MM, Brett CM. Square wave adsorptive stripping voltammetry of molybdenum(VI) in continuous flow at a wall-jet mercury film electrode sensor. Talanta. 1994;41:1597–1601.
- Morfobos M, Economou A, Voulgaropoulos A. Simultaneous determination of nickel(II) and cobalt(II) by square wave adsorptive stripping voltammetry on a rotating-disc bismuth-film electrode. Anal Chim Acta. 2004;519:57–64.
- Chatzitheodorou E, Economou A, Voulgaropoulos A. Trace determination of chromium by square-wave adsorptive stripping voltammetry on bismuth film electrodes. Electroanal. 2004;16:1745–1754.
- Farghaly OA, Ghandour MA. Square-wave stripping voltammetry for direct determination of eight heavy metals in soil and indoor-airborne particulate matter. Environ Res. 2005;97:229–235.
- Alghamdi AH. A square-wave adsorptive Stripping Voltammetric Method for the determination of amaranth, a food additive dye. J AOAC Inter. 2005;88:788–793.
- Alghamdi AF. A square-wave adsorptive stripping voltammetric method for determination of fast green dye. J. AOAC Inter. 2009;92:1714–1719.
- Alghamdi AF, Kooli F. A sensitive procedure for the rapid electrochemical determination of eosin-Y dye using voltammetric techniques onto a mercury electrode surface. J Mater Environ Sci. 2013;4:762–769.
- Berzas JJ, Rodríguez NJ, Flores MJ, et al. Square wave adsorptive voltammetric determination of sunset yellow. Talanta. 1997;44(3):467–474.
- Nagles E, García-Beltrán O. Determination of allura Red in the presence of cetylpyridinium bromide by square-wave adsorptive stripping voltammetry on a glassy carbon electrode. Anal Sci. 2018;34(10):1171–1175.
- Hryniewicka M, Starczewska B, biewska AG. Determination of Budesonide and sulfasalazine in water and wastewater samples using DLLME-SFO-HPLC-UV method. Water (Basel). 2019;11:1581.
- Elks J. The dictionary of drugs: Chemical Data: Chemical Data, structures and bibliographies, 2014;186.
- Lemke TL, Williams DA. Foye's. principles of medicinal chemistry. Philadelphia: Lippincott Williams & Wilkins. 2008. p. 1253.
- Varshosaz J, Emami J, Tavakoli N, et al. Development and validation of a rapid HPLC method for simultaneous analysis of budesonide and its novel synthesized hemiesters in colon specific formulations. Res Pharm Sci. 2011;6(2):107–116.
- Gupta M, Bhargava HN. Development and validation of a high-performance liquid chromatographic method for the analysis of budesonide. J Pharm Biomed Anal. 2006;40(2):423–428.
- Demurtas A, Pescina S, Nicoli S, et al. Validation of a HPLC-UV method for the quantification of budesonide in skin layers. J Chromatogr B. 2021;1164:122512.
- Kotak RK, Pandya CV, Pandya AC. Validation of novel analytical RP-HPLC method for determination of Formoterol Fumarate and Budesonide in inhalation suspension pharmaceutical dosage form. Res J Pharm Tech. 2021;14(8):4383–4390.
- Szeitz A, Manji J, Riggs KW, et al. Validated assay for the simultaneous determination of cortisol and budesonide in human plasma using ultra high performance liquid chromatography-tandem mass spectrometry. J Pharm Biomed Anal. 2014;90:198–206.
- Salem YA, Shaldam MA, El-Sherbiny DT DT, et al. Simultaneous determination of Formoterol Fumarate and budesonide epimers in metered dose inhaler using Ion-pair chromatography. J Chromatogr Sci. 2017;55:1013–1020.
- Matabosch X, Pozo OJ, Perez-Mana C, et al. Identification of budesonide metabolites in human urine after oral administration. Anal Bioanal Chem. 2012;404:325–340.
- Mallikarjuna GM, Ramakrishna SA, Shantakumar SM, et al. Development and validation of selective UV spectrophotometric analytical method for budesonide pure sample. J Appl Pharm Sci. 2011;1(7):158–161.
- Bhusari S, Chaudhari T, Shrangare G, et al. Development and validation of Uv-visible spectrophotometric method For estimation Of budesonide In Bulk And formulation. J Pharm Res. 2018;7(12):300–304.
- Madankar VS, Jadhav SD, Londhe NB. Spectrophotometric methods development and validation of budesonide in bulk and marketed formulation. J Emer Techno Innov Res. 2018;5:438–446.
- Furlanetto S, Orlandini S, Giannini I, et al. Pitfalls and success of experimental design in the development of a mixed MEKC method for the analysis of budesonide and its impurities. Electrophoresis. 2009;30:633–643.
- Alghamdi AF, Hefnawy MM, El-shabrawy Y. Non-extractive ultra-trace determination of simvastatin in biological fluids by voltammetric method via complexation with cadmium. Dig J Nano Bios. 2014;9:355–368.
- Alghamdi AF. Voltammetric analysis of montelukast sodium in commercial tablet and biological samples using the hanging mercury drop electrode. Port Electro Act. 2014;32:51–64.
- Morrison RT, Boyd RN. Organic chemistry, 6th edition. Englewood Cliffs (NJ): Prentice Hall; 1992. pp. 425–430.
- Wang J. Stripping analysis: principles, instrumentation and applications. Deerfield Beach (FL): VCH; 1985.
- Laviron E, Roullier L, Degrand C. A multilayer model for the study of space distributed redox modified electrodes: part II. theory and application of linear potential sweep voltammetry for a simple reaction. J Electroanal Chem Interfacial Electrochem. 1980;112:11–23.