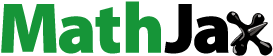
ABSTRACT
Four natural dyes were extracted from different fruits used in the Dye-sensitized solar cell (DSSC). It includes ber, blackberry, black grapes, and blueberry by individual dye or combined with other dyes. The dye solution's absorption spectra were extended to the whole visible region 200–750 nm. FTIR spectroscopy was employed to identify the possible functional group vibrations responsible for the dyes' chemical activity and optical behaviour. The TiO2 powder was coated on the ITO conducting surface to form a thin film. The experimental results showed that the ber dye produces the highest solar cell efficiency (η), ˜13%, among all four extracted anthocyanin studied. It also found that ber combination with other dyes is higher conversion efficiency than blackberry, black grapes, and blueberry with another and vice versa. Ber dye can be helpful in the development of more eco-friendly and cost-effective solar cells with commercially promising DSSC.
GRAPHICAL ABSTRACT
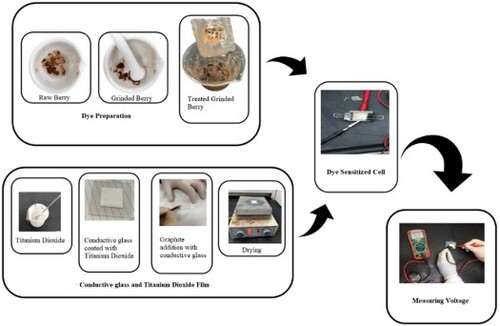
1. Introduction
The growth of any country differs in the development of energy. It may be production, distribution, or consumption. That is why people from various countries continuously use different resources like oil, gas and coal. The natural resources for fuel decrease with time, and one day, they may disappear from the world [Citation1,Citation2]. One particular solution to this challenge is to build renewable energies and feedstocks in adequate quantities to partially or fully meet industry and transport requirements [Citation3]. Solar energy is among them an essential source of renewable energy. This technology can compete for a vital role in future development as a source of sustainable energy [Citation4–7]. Compared to the conventional sources of energy, which are concentrated in a limited number of countries, renewable energy resources exist over broad geographical areas.
Several documents related to the concept of the dye-sensitized solar cell were revealed from 2000 to 2020. Dye-sensitized solar cells (DSSC) produce a cheaper and reliable substitute for various photovoltaic tools, likely inorganic and organic. DSSC technology typically depends on photosensitizer (dye), electrolyte, and the metal oxide semiconductor [Citation8,Citation9]. The history of solar cells advancement showed that “dye” is one of the keywords associated with solar cells [Citation10]. Several parameters involved for the conversion, likely open-circuit voltage, power density in closed circuit, charge transfer resistance at the interface, fill factor, energy conversion efficiency, incident photon-to-current efficiency, depending on semiconductor’s morphological properties, electrolyte’s electrical properties and dye’s spectroscopic properties [Citation11,Citation12]. Organic dye molecules are reflected as efficient due to their high hole-transporting ability, higher extinction coefficient, and less toxicity [Citation13,Citation14]. However, the natural dye extracted from seeds, roots, stem, leaves, fruit, and flower [Citation15,Citation16] is assumed to be transferred electron slowly with less stability and absorbance. But it can easily extract high absorption coefficients in the visible region, uncomplicated synthesis process, cost-effective, biodegradable, environment-friendly, and nontoxic [Citation17]. The most used natural organic dyes are chlorophyll [Citation18–20], carotenes [Citation21,Citation22], curcumin [Citation23], betacyanins [Citation24], and anthocyanins [Citation19, Citation25]. These all can be extracted from fruits raspberries, grapes [Citation26], Malabar spinach, red spinach [Citation27], flowers of amaranthus caudatus, bougainvillaea spectabilis, Delonix regia, Nerium oleander, spathodea companulata [Citation28], eggplants, plums, hibiscus flowers [Citation29], Indian Jamun, plum, black currant, berries [Citation30], spinach leaves, red cabbage, onion peels [Citation31], pomegranate, blackberry, beetroot [Citation32], flowers extract cape honeysuckle, bauhinia, kalanchoe [Citation33], Talisay leaves, spent coffee grounds, fresh Talisay leaves, alugbati fruit [Citation34], sunflower, Indian almond, and green leaves [Citation35]. Some of these fruits are low in crop productivity and high in commercial projections. They require a moderate amount of heat and humidity to control their breeding and production. Furthermore, some of these fruits do not exist in the local store, reflecting on the transportation cost, thus the DSSC running cost.
The maximum efficiency of DSSC can be reached when applying TiO2 as semiconducting material to collect the electrons, iodide/tri-iodide redox-based electrolyte to fill the gas between the counter electrodes and the working electrode, and the dye which absorbs the lights and provide the photoelectron. Then cell efficiency was estimated by measuring the short circuit photocurrent, open-circuit voltage, fill factor. Recent publications in DSSC have been focusing on two major areas. The design of the cell, such as electrodes, the current collector, external circuit; and the second area is where the material such as the semiconductors pastes, electrolytes, and the sensitized dye comes in place. Due to the numerous properties which dyes possess, many publications address them as an exciting area. In DSSC, the component responsible for donating the electron is the dye and should maintain maximum absorbance properties. A proper dye should also be luminescent and should cover UV–Vis is and near-infrared regions. For the electron to travel through the external circuit, the electron must have higher energy than the conduction band energy.
As per our best knowledge, few natural resources have not been tested in the DSSC. Therefore, it is necessary to prepare DSSC and verify all those natural sources abilities within a short time interval and low sensitizer cost such as ber fruit to convert solar energy to electrical energy. Ber considers nutrition-rich hard fruit, which can survive in extreme summer and requires a simple irrigation system. There is usually an abundance of Ber in the market during the harvest season, which down its price. However, due to the perishability property of the fruit, Ber cannot be stored for an extended period making their way to the landfill for fertilization purposes. Ber can supply constant production even in the dry period and crops between 80 and 200 kg fruit depending on the tree size. These potentials can contribute to DSSC markets.
DSSC has been constructed with different fruits in the proposed work, namely blueberry, blackberry, black grapes, and ber dye, to improve the cell’s conversion efficiency. DSSC co-sensitized with four different combinations of green and red dyes to find the optimum combination. Furthermore, natural dyes chemical characteristics and absorption characteristics are measured by FTIR and UV–Vis spectroscopy.
2. Experimental
2.1. Materials and reagents
Titanium dioxide (TiO2), iodine (I2), potassium iodide (KI), ethanol (C2H5OH), nitric acid (HNO3), isopropanol (C3H8O) were bought from Sigma-Aldrich through a local vendor (All reagents are of analytical grade, having more than 99% purity). Conducting glass plates (ITO) (Sigma-Aldrich, 4 cm × 5 cm) are used as substrates. All fruits, blueberry, blackberry, black grapes, and ber purchased from the local market.
2.2. Extraction of natural dye sensitizer
Fresh blueberry, blackberry, black grapes, and ber fruit were found in the local market. The tiny seeds were removed using a spatula. The black and orange-red coloured berry, grape and ber were used for this study as they have higher anthocyanins concentrations [Citation36]. The skin and pulp were dried and crushed into a powder using mortar and pestle (Figure ). It was sonicated in 95% ethanol and acidified with 0.1 M HNO3. Then, the mixture was centrifuged at 1500 rpm for 15 min and decanted. Large particles separated with the help of mesh and filtered using filter paper. All dyes are used as sensitizer sources for DSSC.
The natural dye, blueberry combined with other dyes as per below combination:
Blueberry and blackberry (1:1)
Blueberry and black grapes (1:1)
Blueberry and ber (1:1)
Blueberry and blackberry (1:2)
Blueberry and black grapes (1:2)
Blueberry and ber (1:2)
Blueberry and blackberry (2:1)
Blueberry and black grapes (2:1)
Blueberry and ber (2:1)
Similarly, the blackberry and the black grapes dyes were mixed with other dyes with the same ratio and prepared as the sensitizer for DSSC.
2.3. Preparation of conductive ITO glass
The conductive ITO glass with a resistivity of 10 Ω/cm was bought from Sigma-Aldrich. Two-piece of conductive glass joined separately into two identical beakers comprising about 20 ml absolute ethanol. Both of the beakers undergo an ultrasonic bath for 10 min. Then resistivity decreased to 8 Ω/cm, which improved the ITO substrate’s conductivity.
2.4. Preparation of electrolyte solution
The electrolyte solution was prepared by dissolving KI (2.08 g) and I2 (0.32 g) in 25 ml ethylene glycol [Citation37]. The solution was kept in the dark to avoid direct sunlight per the guideline [Citation38].
2.5. Assembly of the solar cell ITO
At first, the ITO coated glass plates were washed and cleaned with detergent, followed by a rinse with distilled water, then with acetone and isopropyl alcohol for a minimum of 5 min. TiO2 (6.5 g) mixed with 10 ml of vinegar until obtaining a cohesive paste. Then the paste was applied to one of the pretreated ITO glasses (working electrode) by maintaining a fixed thickness of 0.04 mm layer using a screen printing method. It was then kept for a few minutes to dry and sintered on a hotplate for 20 min at 300°C. Later on, it was cooled at room temperature. The blueberry dye, preferably one or two drops, was added to the TiO2 layer, uniformly distributed on the glass, and kept for a few minutes. Another ITO coated glass was used to draw a few lines with a pencil, assumed as the counter electrode. Both ITO glasses were sandwiched with the help of binder clips, followed by 3–4 drops of the electrolytic solution in between, and measuring the voltage using the voltmeter (Figure ). A 200–watt incandescent bulb was used as the light source to the above setup to measure the voltage, which aids to plot the J-V curve and the power–voltage curve. The photocurrent was calculated for each recorded data using Ohm’s law
Also, the power for each load resistance was calculated using the following formula
Similar procedures were continued with blackberry, black grapes and ber dye (Figure ).
3. Results and discussion
3.1. UV–Visible spectrum of natural dye
The UV–Vis absorption spectra of blueberry, blackberry, black grapes, and ber fruit dye are determined. The measured absorbances resulted from a wide range of visible light with the extracted dye. The extracted dye shows several possible characteristics of potential dye sensitizers and its absorption proficiency within the visible region 350–700 nm. Also, it displays the conversion capacity of light energy to electrical energy. The distinct spectral ranges were observed with extracted dye due to the natural dye's varying amounts of anthocyanins and carotenoids. It was noticed that the absorption of blueberry and black grapes is widest compared to blackberry and ber fruit dye as indicated in (Figure ). It was also seen that there is an absorption peak at about 515 nm of the blackberry extract. Other dyes showed their absorption peak at 530, 510, 505 nm, with blueberry, black grapes, and ber dyes, respectively, compared to literature. The combination of dyes (2:1) has shown an absorption peak at 455, 463, 471, 478, 483, 488 nm, and the main absorption range was within the range of 450–500 nm.
Widen absorption spectrum, and higher absorbance in the visible range increases the possibility of higher solar cell efficiency [Citation39]. UV analysis expected a shift in the absorption spectra of both the dyes upon loading into the TiO2. The absorption spectrum was lengthened to the whole visible region due to all these moderately better properties [Citation40]. It supports identical components like anthocyanins and the functional groups [Citation41], which considers the core component of natural dyes [Citation42].
3.2. FTIR spectroscopy
The dye characterization was continued using Fourier transform infrared (FTIR) spectroscopy to investigate the functional groups of the extracted dyes. Figure shows the result of the FTIR spectra of four dyes.
All dyes showed very similar transmission wavenumber spectrum ranging from 4000 to 600 cm−1. The broad absorption range between 3200 and 3450 cm−1 indicates the chemical has an intermolecular H-bond. Strong absorption between 1600 and 1700 cm−1 reveals that C = O stretching vibration is conjugate. The peak at 1030–1060 cm−1 is the C–O–C stretching vibration of esters acetates (Figure ). From this result, we understood that all the presented dyes shared almost identical chemical compounds, listed in Table pigments and their functional groups. This result also showed that the ber extract from the ber fruit maintains an anthocyanin property.
Table 1. Pigments and functional groups are present in the dye.
3.3. Determination of total anthocyanins
The pH differential method determines the total anthocyanins contents [Citation43] to estimate the anthocyanins content in each dye. All the dyes are prepared in two different buffers. Potassium chloride buffer was prepared by taking 1.86 g KCl and dissolved in a 1000 ml volumetric flask with distilled water, and pH = 1 is adjusted with hydrochloric acid (HCl). The second sodium acetate buffer pH = 4.5 was prepared by diluting 54.43 g sodium acetate in a 1000 ml volumetric flask. Its pH was maintained with HCl. The same procedure was followed then absorbances were measured at 510 and 700 nm. The values were expressed as mg cyanidin-3-glucoside (c3 g) equivalents per 100 g fresh weight using a molar extinction coefficient of 26.900. All determinations were performed in triplicates. The amount of anthocyanins present in the fruit dyes are represented in Table .
Table 2. Amount of anthocyanins in fruit dye.
3.4. Photoelectrochemical properties of DSSCs sensitized with natural dyes
The current studies included preparing DSSC using the nanoparticles TiO2. The synthesis involved the natural dyes, mainly from blueberry (Cyanococcus), blackberry (Rubus), black grapes (Vitis vinifera) and ber fruit (Ziziphus mauritiana), along with the counter electrode and iodide/tri-iodide couple electrolyte solution. An incandescent bulb is used as a light source to plot the current–voltage curve (I–V) and power–voltage curve (PV). When the beam of light energy hits the working electrode, photon enters the DSSC, absorbed by the sensitizer dyes of our samples which are present in the inner pores of the TiO2 layer and motivate the electrons to the conduction band of the adjacent particles, leaving the oxidized molecules on the surface of the thin film. The electrolyte helps for the diffusion of the ions and the further connectivity of DSSC.
The performance of DSSC has been evaluated with the help of current–voltage (I–V) characteristics curves (Figure ) by measuring the maximum output voltage (Voc), maximum output current (Isc), fill factor (FF) and % efficiency (η). The maximum power output (Pmax) was measured from the I–V characteristics curve using equation 2, where the voltage and the current were at maximum values. The Pmax was determined by using the following relations:
Where Vmax and Imax were the maximum voltage and current at the Pmax, respectively.
FF determined the overall performance of the cell. The FF and η of the cell have been determined by using the following relations.
Where Pin is denoted as the input power (100 mW/cm2).
Figure shows the current density of the DSSC versus the voltage fabricated in-house with the simple setup to evaluate the cell performance using different extracted anthocyanin dyes. The I–V data was recorded by varying the voltage from 0 to 500 voltage (mV). (mention again in method section)
From the above figure, it was observed that the ber dye maintained the highest current density (0.28 mA/cm2) among all four dyes. Followed by blackberry, which shows 0.26 mA/cm2 of current density when tested in the DSSC, reflecting an improvement of ∼13% in the efficiency of the DSSC as listed in Table . The ber dye’s cell efficiency was improved due to its functional groups. It enhances absorbance properties, increasing the dye molecule excitement and facilitating the electron's travel from the ground to the excited state. The difference in the current density values in the DSSC using different types of dyes may also attribute to the anthocyanins concentration in each fruit.
Table 3. Photoelectrical parameter for DSSC treated with FTO/TiO2.
Furthered investigation of the DSSC performance has been made by using combination dye solutions. These dyes were mixed in different ratios to obtain the highest current density in the DSSC and compare it with the ber dye. Twenty dyes combinations were prepared from the four fruits at three different ratios, 1:1, 1:2, and 2:1, to investigate the performance of the DSSC through boosting the properties of the dye. All dyes combinations were coated on the working electrode and sandwiched with the counter electrode following the aforementioned procedures. Figure shows the DSSC I–V curve at room temperature and an active cell area of 20 cm2.
Figure shows the current densities of different dyes combinations extracted from four fruits. The combination dyes which contain ber was always showing a higher current density when running the solar cell. These mixed dyes were prepared and tested in the DSSC to investigate the better performance in the DSSC efficiency when using the ber dye. Also, to elevate the electron transfer properties from the dye molecules to the conduction band and the functional group bonding properties with the porous surface of the TiO2 film. Among all the combination dyes, the ber: Blackberry (2:1) ratio showed the highest current density of 0.225 mA/cm2. Followed by the Ber: Black Grapes (2:1) 0.205 mA/cm2, the Ber: Blueberry (2:1) 0.19 mA/cm2.
Table summarizes the photoelectrical properties such as Voc, Isc, FF and η values of DSSC using single individual natural dyes and combining these four dyes with different volume ratios. It also included the photoelectrical parameters of DSSC using other dyes and their various combinations. The result indicated that the % efficiency is higher with ber dye and its combination than berries and grapes. As well, the maximum output voltage (Voc), maximum output current (Isc), and fill factor (FF) were maximum with ber fruit dye. The anthocyanin and its phenolic substances are responsible for the efficiency.
The essential colour pigments in fruits are anthocyanin, carotenoid and chlorophyll. The amount of chlorophyll decreases as the fruit ripens; hence anthocyanin and carotenoid are responsible for output current density in the dye-sensitized solar cell. Ber fruit is the seasonable fruit. It contains nine different phenolic compounds: para coumaric acid, ortho coumaric acid, vanillin, ferulic, para hydroxybenzoic, protocatechuic, vanillic, and caffeic acid [Citation44]. These values vary from place to place, climate and other factors. It also contains a higher amount of bioactive components, involving polyphenols and triterpenic acids. It also has higher amounts of flavonoids, including procyanidin B2, epicatechin, quercetin-3-O-rutinoside, quercetin-3-O-galactoside, kaempferol-glucosyl-rhamnoside [Citation45]. The mixture of a variable amount of lutein, β-carotene, and anthocyanin is perhaps responsible for the better dying nature of ber fruit compared to the other berries. Therefore, the DSSC efficiency showed a higher result when using ber fruit dye than blackberry, black grapes, blueberry.
4. Conclusion
The current study includes the physical nature of DSSC, environment–friendly materials, processing, and realization of various anthocyanin sensitizing dyes used to investigate the solar cell performance. The experimental results show that the economical ber dye, compared to berries and grapes, maintained the highest efficiency and other dyes. Also, it produced a photoelectric conversion efficiency (η) of up to 1.194%, an open-circuit voltage (VOC) of 0.4 V, and a short-circuit current (JSC) of 5.71 mA. As well, its combination with other dyes is also more compared to berries and grapes. To our best knowledge, until now, ber fruits have not been used with DSSC for the conversion and consider an economical dye source for the use of organic solar cells. Blackberry and black grapes are well enough for conversion than blueberry. Still, it can be used as a sensitizer for the DSSC. Therefore, the dye extracted from different fruits is an excellent pigment for converting solar energy to electrical energy.
Disclosure statement
No potential conflict of interest was reported by the author(s).
References
- Nehring R. Traversing the mountaintop: world fossil fuel production to 2050. Philos Trans R Soc Lond Ser B Biolog. Sci. 2009;364:3067–3079.
- Dolf G, Francisco B, Deger S, et al. The role of renewable energy in the global energy transformation. Energy Strat. Rev. 2019;24:38–50.
- Verzijlbergh RA, De Vries LJ, Dijkema GPJ, et al. Institutional challenges caused by the integration of renewable energy sources in the European electricity sector. Renew Sustain Energy Rev. 2017;75:660–667.
- Olivier B. Hydrogen fuel cell road vehicles and their infrastructure: An option towards an environmentally friendly energy transition. Energies. 2020;13:6132.
- Mahmure OAA, Ayse AS, Seniye UOF, et al. A comparative analysis of renewable energy use and policies: global and Turkish perspectives. Sustainability. 2015;7:16379–16407.
- Joseph O, Mario P, Vittorio T, et al. Impacts of renewable energy resources on effectiveness of grid-integrated systems: succinct review of current challenges and potential solution strategies. Energies. 2020;13:4856.
- Alper K, Tekin K, Karagoz S, et al. Sustainable energy and fuels from biomass: a review focusing on hydrothermal biomass processing. Sus Ener Fuels. 2020;4:4390–4414.
- Mohamed Y, Asmae B, Cemil O, et al. Organic/metal-organic photosensitizers for dye-sensitized solar cells (DSSC): recent developments, new trends, and future perceptions. Dyes Pigm. 2021;192:109227.
- Karam AK, Fadhil AMQ. National grid connected 3-phase inverter based on photovoltaic Solar System. J Eng. 2020;26:21–43.
- Fraas LM. History of solar cell development, low cost solar electric power. Switzerland: Springer International Publishing; 2014, doi:10.1007/978-3-319-07530-3.
- Sharma K, Sharma V, Sharma SS. Dye-sensitized solar cells: fundamentals and current status. Nanoscale Res Lett. 2018;13:381.
- Rebecca JY. Overcoming technical barriers and risks in the application of building integrated photovoltaics (BIPV): hardware and software strategies. Automa Constru. 2015;51:92–102.
- Qian X, Lan X, Yan R, et al. T-shaped (D)2–A–π–A type sensitizers incorporating indoloquinoxaline and triphenylamine for organic dye-sensitized solar cells. Electrochim Acta. 2017;232:377–386.
- Qian X, Wang X, Shao L, et al. Molecular engineering of DeDepeA type organic dyes incorporating indoloquinoxaline and phenothiazine for highly efficient dyesensitized solar cells. J Power Sources. 2016;326:129–136.
- Ono T, Arakawa HY. Study on dye-sensitized solar cell using novel infrared dye. Sol Energy Mater Sol Cells. 2009;93:831–835.
- Narayan MR. Review: dye sensitized solar cells based on natural photo-sensitizers. Renew Sustain Energy Rev. 2012;16:208–215.
- Shalini S, Balasundaraprabhu R, Prasanna S, et al. Review on natural dye sensitized solar cells: operation, materials and methods. Renew Sustain Energy Rev. 2015;51:1306–1325.
- Syafinar R, Gomesh N, Irwanto M, et al. Chlorophyll pigments as nature based dye for dye-sensitized solar cell (DSSC). Energy Proc. 2015;79:896–902.
- Pratiwi DD, Nurosyid F, Kusumandari, et al. Performance improvement of dye-sensitized solar cells (DSSC) by using dyes mixture from chlorophyll and anthocyanin. J Phys Conf Ser. 2017;909:012025.
- Kabir F, Sakib SN, Matin N. Stability study of natural green dye based DSSC. Optik Int J Light Electron Optics. 2019;181:458–464.
- Yamazaki E, Murayama M, Nishikawa N, et al. Utilization of natural carotenoids as photosensitizers for dye-sensitized solar cells. Sol Energy. 2007;81:512–516.
- Feng Y, Meng L, Zhao J, et al. Bioorganic dye-sensitized solar cell of carotenoid–pheophytin a–TiO2. RSC Adv. 2014;4:63016–63024.
- Kim HJ, Kim DJ, Karthick SN, et al. Curcumin dye extracted from Curcuma longa L. used as sensitizers for efficient dye-sensitized solar cells. Int J Electrochem Sci. 2013;8:8320–8328.
- Dongshe Z, Suzanne ML, Jonathan A, et al. Betalain pigments for dye-sensitized solar cells. J Photochem Photobio A Chem. 2008;195:72–80.
- Garcia CG, Polo AS, Iha NYM. Fruit extracts and ruthenium polypyridinic dyes for sensitization of TiO2 in photoelectrochemical solar cells. J Photochem Photobiol A Chem. 2003;160:87–91.
- Khan MZH, Al-Mamun MR, Al-Amin M, et al. Dye-sensitized solar cell using used semiconductor glass and natural dye: towards alternative energy challenge. Int J Renew Energy Res. 2015;5:38–44.
- Kabir F, Bhuiyan MMH, Manir MS, et al. Development of dye-sensitized solar cell based on combination of natural dyes extracted from Malabar spinach and red spinach. Results Phys. 2019;14:102474.
- Desalegn JG, Sisay TA, Teketel YA. Dye sensitized solar cells using natural pigments from five plants and quasi-solid state electrolyte. J Braz Chem Soc. 2015;26:92–101.
- Gidea TT, Aline V, Bianca VD, et al. Recombination study of dye sensitized solar cells with natural extracts. J Braz Chem Soc. 2019;30:371–378.
- Nipun S, Soumitra S. Utilization of naturally occurring dyes as sensitizers in dye sensitized solar cells. IEEE J Photovol. 2017;7:539–544.
- Ahmed MA, Hemdan SHM, Moataz MKY, et al. Dye-sensitized solar cells (DSSCs) based on extracted natural dyes. J Nanomater. 2019: 1867271.
- Samia T, Ayesha S, Munira S, et al. Assemble of dye sensitized solar cell based on locally available natural dye. J Atmos Sci Res. 2019;2:32–36.
- Giphin G, Raja SY, Anu ME. (2020) Fabrication of dye-sensitized solar cells using natural flower dye extracts: a study on performance analysis and solar dye degradation. Energy Sources Part A Recov Utiliz Environ Effects. doi:10.1080/15567036.2020.1796849
- Gee JCB, Jhon PSDM, Judy ACA, et al. Performance of dye-sensitized solar cells with natural dye from local tropical plants. Mindanao J Sci Tech. 2020;18:242–258.
- Selva E, Meenakshi S. Fabrication of natural dye sensitized solar cell using sunflower, Indian almond fruit and pongame oil tree leaf extracts with TiO2 photoanode. Int J Sci Tech Res. 2020;9:7082–7087.
- Khoo HE, Azlan A, Tang ST, et al. Anthocyanidins and anthocyanins: colored pigments as food, pharmaceutical ingredients, and the potential health benefits. Food Nutr Res. 2017;61:1361779.
- Kabir F, Sakib SN. Various impacts of blocking layer on the cell stability in natural dye based dye-synthesized solar cell. Optik (Stuttg). 2019;180:684–690.
- Kabir F, Bhuiyan MMH, Hossain MR, et al. Improvement of efficiency of dye sensitized solar cells by optimizing the combination ratio of natural red and yellow dyes. Optik (Stuttg). 2019;179:252–258.
- Alniami JH, Saggar RAM, Abbas MF. The physiology of ripening of jujube (Ziziphus jujuba Mill.). Sci Hortic. 1992;51:303–308.
- Varsha Y, Swati C, Chandra MSN, et al. Textile dyes as photo-sensitizer in the dye sensitized solar cells. Opt Mat. 2020;109:110306.
- Bastos VJ, Neves LC, da Silva PMC, et al. Harvest point determination of Indian jujube fruit (Ziziphus mauritiana L.) based on physicochemical and functional parameters. Sci Hortic. 2016;213:392–402.
- Shi Q, Zhang Z, Su J, et al. Comparative analysis of pigments, phenolics, and antioxidant activity of Chinese jujube (Ziziphus jujuba Mill.) during Fruit Development. Molecules. 2018;23:1917.
- Giusti MM, Wrolstad RE. Characterization and measurement of anthocyanins by UV-visible spectroscopy. Curr Protocol Food Anal Chem, John Wiley & Sons, 2001. doi:10.1002/0471142913.faf0102s00
- Ayaz AM, Najma M, Muhammad IB, et al. Phenolic acids composition of fruit extracts of ber (Ziziphus mauritiana L., var. Golo Lemai). Pak J Anal Environ Chem. 2012;13:37–42.
- Guo S, Duan JA, Zhang Y, et al. Contents changes of triterpenic acids, nucleosides, nucleobases, and saccharides in jujube (Ziziphus jujuba) fruit during the drying and steaming process. Molecules. 2015;20:22329–22340.