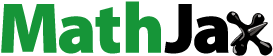
Abstract
Al Bayda’s younger granitic pluton located within the western Arabian Shield, Al Madinah area, KSA, has been studied using collaborative techniques. The collected samples were studied petrographically using a polarizing microscope and geochemically using the XRF technique. Four suites of intrusions can be distinguished based on their mineralogical and geochemical characteristics. A muscovitized epidotize hornblende leucocratic altered granite is the earliest intrusion, followed by perthite alkali feldspar granite. Both are intruded by a chessboard-like texture, Arfvedsonite-aegirine nepheline peralkaline granite. These three types of younger granitoid rocks are intruded by pegmatite-aplite sheets. The metasomatically altered epidotized, muscovitized granite formed during a late magmatic phase that was probably followed by successive co-magmatic and hydrothermal alterations in a closed system. The metasomatic alteration produced enrichment in Zr (up to 699 ppm), Nb (up to 67 ppm), and REE (up to 261 ppm) contents. As a result, residual alkalis, fluoride complexes, and iron-rich mixing fluids contribute to these enrichments. The hypersolvus perthite alkali feldspar exhibited post-orogenic A-type geochemical characteristics, whereas the peralkaline exhibited an anorogenic affinity, and both have been generated within plate-related tectonic settings. An A-type signature is probably the result of melting in the lower crust, whereas tectonic mechanisms shifted from post-orogenic to anorogenic due to the Najd fault system. Utilizing Al Bayda granite placer deposit products in the ceramic industry requires removing zircon minerals. The recorded HFSE, Nb, and REE are also recommended for detailed exploration.
1. Introduction
This study concerns petrogenesis, classification, and co-magmatic hydrothermal alteration of the Nb-Zr bearing younger granitoid rocks located in Jabal Al Bayda, Al Madinah area, KSA (Figure ). The study area is considered a part of the Arabian Shield (770.000 km2, [Citation1]), which represents one of the largest tracts of juvenile crust on the earth [Citation2Citation3]. Plutonic rocks of granitic composition are widespread and abundant in the Arabian Shield and include granodiorite, monzogranite, syenogranite, and alkali feldspar granite peralkaline, metaluminous, and peraluminous in terms of alumina saturation [Citation1]. The Arabian Shield contains one of the largest fields of alkali granites globally [Citation4]. Moreover, the alkali-feldspar granite constitutes about 7% of all plutonic rocks of the Arabian Shield [Citation5]. Al Bayda’s younger granitoid exposures intruded the Neoproterozoic volcanoclastic sedimentary rocks of the Al Ays group and were extruded by the Neogene basaltic rocks. The exposed younger granitoid rocks located in Jabal Al Bayda, Al Madinah area, KSA, were classified as Alkaline to the peralkaline complex by Elliott [Citation1] and as alkali granite, alkali feldspar granite, and quartz syenite by Gougazeh et al. [Citation6]. The alkali feldspar granites are typically chemically more evolved than the monzogranites and syenogranites and form the most important environment in the Arabian Shield for mineral deposits associated with granitic rocks [Citation5]. Overall, the alkaline igneous settings are among the most favourable sites for the exploration of high field strength elements (HFSE). Furthermore, the alkaline granitoid suites form along with some aluminous granite, one of the economically important groups of granites in the Arabian Shield, and are associated with Nb, Ta, Th, U, Sn, W, F, Mo, Zr, Y and rare earth elements’ (REE) mineralization [Citation7–Citation10].
Accordingly, Jabal Al Bayda is generating considerable interest in terms of exploration surveys as part of the Arabian Shield and consider a promising area to undertake such survey, especially for the rare ore metals. The rare ore metals’ high anomalous contents are expected in Al Bayda altered granite and their proper Pre-Cambrian younger alkaline granitoid rock units. A few studies on the study area were published, where most of the previous studies have discussed the geologic setting [Citation1Citation5], evaluation of this granite as a feldspar source [Citation6], rock classification or even proposed a geologic map [Citation11]. However, geochemical exploration efforts were attempted in the study area by the Saudi ministry of petroleum and mineral resources a long time ago. Meanwhile, what is known about the study area is largely based on Pellatone’s work [Citation11]. Regarding ore metals exploration efforts in the study area, in the last 40 years, there has been no considerable growing interest in research and literature. Moreover, the studied granitoid did not receive much attention in petrogenetic modelling, classification, and magma generation. Therefore, the present work aims to investigate the petrographic and geochemical classifications as well as magma type, tectonic setting, and geochemical signature of Jabal Al Bayda granitoids to attain their petrogenesis and origin of fresh and altered bedrocks and their related expected mineralization.
A combined petrographic, whole rock-major, and trace element analysis of the Jabal Al Bayda granitoids is presented. The primary aim is to constrain the petrogenesis of the diverse granitoid suites (alkali feldspar granite to aplite-pegmatitic granites) and characterization of Nb-Zr enrichment in the studied granite. We also explore the potential for feldspar sands for ceramic production and the studied granites for Nb-Zr deposits.
1.1. Geologic setting
Jabal Al Bayda area is located between Long. 39° 20′ & 39° 30′ E and Lat. 24° 40′ & 24° 45′ N. Al Bayda’s oval-shaped granitoid outcrop is trending in the NW direction. The study area is composed mainly of Pre-Cambrian intrusive and extrussive igneous rocks and slightly metamorphosed volcanoclastic sedimentary rocks (Figure ). The basement rocks in the study area occur as uplands, scarp, and low land mountainous relief, where the Phanerozoic cover rocks have been stripped because of Paleozoic epeirogeny and Neogene ramping. Shield outcrops are divided into three tectonic provinces by NW trending shear zones of the Najd fault system (NFS) of late Proterozoic and possibly early Paleozoic time. A modified geologic map (Figure ) of the studied district has been constructed based on the geological observations, field relationships, and the petrographic investigations of the different rock units collected from the studied area. The exposed rock units in the studied localities are arranged from oldest to youngest in Table .
Figure 2. Geologic map of the studied district; Modified after pellaton [Citation11].
![Figure 2. Geologic map of the studied district; Modified after pellaton [Citation11].](/cms/asset/0f6f6c12-fc51-4963-b0e4-582ec17980cf/tusc_a_2077615_f0002_oc.jpg)
Table 1. Lithostratigraphic units in the Al Bayda area.
The oldest exposed rock unit in the studied district is Al Ays’ volcanoclastic sedimentary rocks. Al Ays group is highly jointed and fractured, dipping mainly in Najd fault system direction ∼30°–50° NW-SE with other subordinate NE-SW and E-W directions. In the studied area, the Al Ays group is represented by Farshah formation (Fm) and Urayfi Fm (Figure ). Al Ays group is divided into western facies of predominantly metasedimentary rocks and eastern facies of predominantly metavolcanic rocks. Al Ays group age ranges from 725 ± 12 (U-Pb and Sr; [Citation12]) to 736 ± 5 Ma (zircon data; [Citation13]).
In the study area, the granitic rocks intrude in the Al Ays group in sharp thermal contact. Based on field relationships, granitoids arranged from oldest to youngest as altered mica-rich granites, followed by fresh K-feldspar-rich granites, are intruded by fresh mafic-rich granitoids. The fresh granitic rock units that form the main bulk of Jabal Al Bayda pluton are exposed as small circular moderate altitude stocks having less than several kilometers in diameter (Figure (a)). They are distinguished by their red colour with abundant subhedral quartz crystals. The studied alkali feldspar granite occurs as massive to locally foliated, homogeneous, medium to coarse-grained rocks (Figure (b)). Al Bayda granitoids are highly exfoliated due to the wide temperature variation range (Figure (c)). Some parts of the pluton show a variable degree of deformation and hydrothermal alteration; those are more common and intense in the southern part of the pluton. The altered mica granite with alteration zones is exposed near the contact with Neogene basaltic rocks and occurs as whitish pink colour altered granite (Figure (d&e)).
Figure 3. The main geologic features of Al Bayda granites; (a) general view of the studied granites; (b) NW trending NFS cutting through Al Bayda granite; (c) Al Bayda granitic exfoliation; (d) The contact between the studied granites and Neogene basaltic rocks; (e) Altered granites and alteration sites. Gr = Granite & Bs = Basalt.
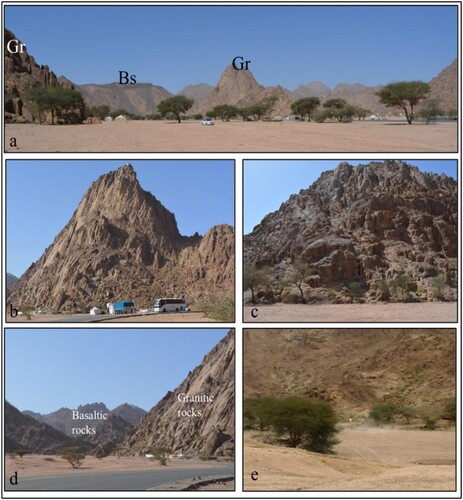
The altered granitic exposures occupy the southern side of Jabal Al Bayda with sharp intrusive contact at low altitudes. The studied granitic rocks are fractured and jointed with parallel joints. The main fracture trend is NW, reflecting the effect of the Najd fault system, with subordinate and cross-cutting E-W direction reflecting the Red Sea system. Mafic and felsic dikes crosscutting the alkali feldspar granite are common. Moreover, numerous veins of aplite and endogenous pegmatite are also recorded. Rubidium strontium age determinations run on samples of this granite gave 561 ± 16 m.y. [Citation14]. Moreover, Stoeser [Citation5] stated that these rocks were emplaced between 670 and –565 Ma, and most are younger than 630 Ma. Al Bayda granite is highly altered and metasomatized (Figure (d&e)), especially when it comes to contact with the Neogene basalt. Moreover, fresh and altered granitic rocks are intruded with pegmatite-aplite sheets. Sometimes, pegmatite-aplite rocks occur as xenoliths included in the studied massive alkali feldspar granites. These field observations encourage the authors to believe in multiple generations of the pegmatite-aplite rock unit. Furthermore, the Al Bayda pluton is extruded with the Red Sea rift-related Oligo-Miocene basaltic flood. These basaltic rocks occur principally in the centre and on the western edge of the area and less extensively to the east of the Jabal al Bayda (Figure ). This basalt has weathered into rounded blocks which have been considerably eroded by meteoric action. The surface of the flows forms a scrap with gravel deposits between well-rounded basalt blocks (Figure (a)).
1.2. Petrography
The studied thin sections of Al Bayda granitoid rock units reveal the presence of a wide variety of fresh and altered younger granitoid rocks. These granitic rocks are depicted in thin sections as alkali feldspar, peralkaline, altered albitized-muscovitized epidotized hornblende perthite, and aplite as pegmatoidal granites. Hereunder, the detailed petrographic description of each is given.
1.2.1. Alkali feldspar granite
The studied alkali feldspar is petrographically composed essentially of orthoclase, quartz, hornblende, biotite, and muscovite arranged in decreasing order. The modal analyses of the studied alkaline granites are given in Table and Figure .
Figure 4. The modal analysis of the studied granites on the ternary diagram of Streckeisen [Citation15].
![Figure 4. The modal analysis of the studied granites on the ternary diagram of Streckeisen [Citation15].](/cms/asset/01cf5806-f83a-49ca-93fa-3a9957382b29/tusc_a_2077615_f0004_ob.jpg)
Table 2. The modal analyses (mineral %) of the studied alkaline granites.
In the thin sections, the studied fresh alkali feldspar granite is depicted hypersolvus perthite alkali feldspar granite (Figure ). It occurred as holocrystalline coarse-grained perthitic equigranular texture granite. This rock is composed essentially of microcline and orthoclase perthites (54–61%), quartz (34–38%, Table , Figure ), and hornblende (3–6.5%), as well as subordinate contents of muscovite (<1%) and a few grains of biotite. The accessory minerals are represented by sphene, zircon, iron oxides and opaque minerals (Figure ). The orthoclase and microcline-perthite appear as subhedral coarse crystals of moderate relief associated with vein and patchy inter-growth of exsolved plagioclase within the host crystal of orthoclase and microcline (Figure ).
Figure 5. Photomicrographs showing alkali feldspar granite essential, accessory, and opaque minerals and their mutual relationships.
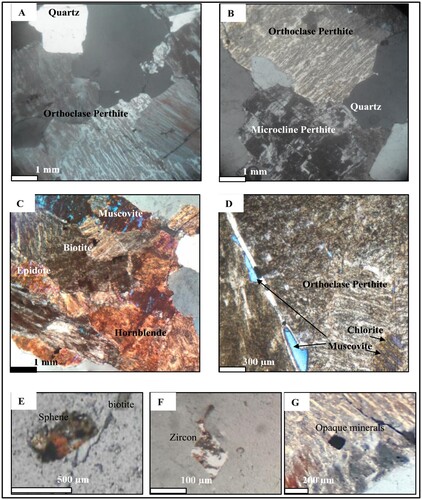
Orthoclase and microcline perthitic occur in an equigranularity mutual relationship with coarse grain quartz. Both types of perthite are slightly fractured, fissured and slightly sericitized, especially along their edges. The fractures and fissures are filled with iron oxides. Furthermore, the perthite shows subordinate poikilitic texture (Figure ). There are two types of quartz, medium to coarse-grained euhedral to subhedral moderate to low positive relief equidimensional crystals displaying wavy extinction and intergrown crystals with feldspar crystals. Hornblende occurs as anhedral grains with obvious cleavage and high anomalous interference colours. Muscovite that filled the interstitial spaces between feldspars and hornblende occurs as subhedral prismatic colourless fine to very fine grain crystal exhibited high anomalous interference colours. Biotite is represented by fine crystals with moderate relief, faint cleavage, and marked pleochroism from light brown to dark brown and stained with iron oxides (Figure (d)). Zircon is subhedral prismatic with high relief (Figure (f)) crystals. It is enclosed in perthite crystals and shows high-order polarization. Sphene occurs as fine-grain euhedral short prismatic, light grey crystals with two sets of cleavage and high anomalous interference colours and high relief included by perthite (Figure (e)). The recorded opaque minerals that are mostly scattered within perthite and quartz, are rare and represented by ilmenite and magnetite (Figure (g)). The opaque minerals are equant disseminated and patchy crystals (Figure (g)).
1.2.2. Peralkaline granite
Arfvedsonite-aegirine nepheline perthite granite or peralkaline sub-solvus granite, exhibits holocrystalline coarse-grained porphyritic to equigranular texture with a local tendency of chessboard-like texture (Figure (a)). Furthermore, the phenocrysts demonstrate orientation and eventually show cumulative texture.
Figure 6. Photomicrographs showing peralkaline granite essential, accessory, and opaque minerals and their mutual relationships.
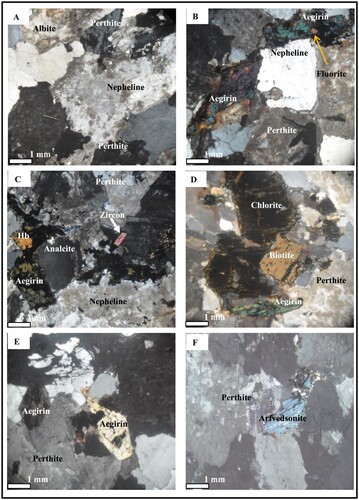
The studied peralkaline granite is composed essentially of fresh orthoclase and microcline perthite, albite, and Na-mafic silicates (i.e. clinopyroxene and amphiboles), nepheline, and albite (Figure (a&b)). The minor and accessory minerals are epidote, sphene, zircon, biotite, muscovite, and opaque minerals (Figure (c)). Exsolved orthoclase and microcline perthite occurs as cloudy and grey subhedral coarse (up to 3 mm) laths and grains invaded with clear fresh fine to medium euhedral rectangular microcline and albite crystals. More than one generation (i.e., primary and secondary generation) of microcline and orthoclase perthite have been recorded (Figure (d)). The alkali feldspar makes up a framework with interstitial nepheline and mafic silicates. The exsolved feldspar is complete, even in slightly altered nepheline grains. Furthermore, most of the perthite surface is fractured. Replacement of nepheline by aggregates of sericite, calcite and albite begins along grain boundaries and fractures (Figure (e)). Fresh coarse anhedral twined nepheline crystals with obvious irregularities to sub-conchoidal fractures, grey polarization colours and straight extinction are recorded (Figure (f)). Despite the bulk of nepheline mineral grains being fresh, aggregates of sericite, calcite and albite grains along the grain boundaries and fractures have been recorded. Albitization is recorded within the studied thin section (Figure (a)). Albite plagioclase occurs as subhedral fine laths and sericitized equidimensional or tabular crystals. Aegirine that is recorded in relatively abundant content occurs as a medium subhedral to euhedral nature prismatic green colour crystals depicting high positive relief, weak pleochroism, and ∼45° extinction and characteristic patterns of cleavage (Figure (b)). Moreover, some pyroxenes are zoned, with rims showing a stronger green colour than the cores. In this respect, the overgrowth of pale green clinopyroxene by grass-green, pleochroic amphiboles is manifested. Amphibole recorded in relatively abundant content occurs as subhedral medium tabular to prismatic grains exhibiting pale green to olive-green pleochroism, obvious cleavage and intermediate interference colours of the second order (Figure (c)). All amphibole grains occur in contact with fresh nepheline. Furthermore, most amphibole crystals are cracked and invaded with fine-grain Fe-oxide opaque minerals. Meanwhile, arfvedsonite alkali amphibole anhedral fibrous high polarization colour crystal is recorded with a straight structure between the albitized perthite (Figure (f)).
Epidote is an abundant minor mineral occurring as pale-yellow green elongated prismatic medium grain with perfect cleavage and anomalous interference colours (Figure (d)). Most epidote grains are cracked and contain relics of pre-existing amphibole. Moreover, fine grain epidote is also recorded within the fractures of perthite and nepheline. Sphene is relatively occurring as colourless sphenoidal subhedral to euhedral crystals with high anomalous interference colours. However, most sphene crystals are nearly included in and between all the essential rock-forming minerals except clinopyroxene. Zoned high relief, anomalous polarization colours, and euhedral tetragonal prismatic zircon crystals with pyramidal terminations are recorded as minor accessory minerals (Figure (e)). Fluorite, magnetite, and apatite (Figure (b)) are recorded as accessory minerals. Apatite crystals are acicular and zoned with “dusty” core, rich in inclusions with a clear rim. The recorded opaque minerals are mostly homogeneous magnetite with a small hematite content. Some miarolitic cavities are filled with minerals that have crystallized after feldspar, nepheline, and pyroxene (Figure (f)). Euhedral laths of dark green chlorite after biotite are also recorded.
1.2.3. Altered muscovite perthite granite
The alteration zone enclosed between the adjacent alkali-peralkaline granitic suites and Neogene basaltic rocks exhibits a yellowish to reddish-brown intermediate to irregular fine-grained and a gradational contact zone (Figure (e)). The studied hydrothermal altered granitic rocks are represented by muscovitized epidotized k-feldspar granite. This altered granite occurs at relatively intermediate temperature, slightly pervasive metasomatic, fractured, and fissured altered perthite granite. Metasomatic altered muscovitized epidotized hornblende leucocratic granite (Figure ) exhibits intergranular to perthitic chessboard-like texture (Figure (a)). The rock is formed of coarse grain subhedral orthoclase, microcline perthite, quartz, muscovite, hornblende, epidote, fluorite, iron oxides, opaque minerals and clay minerals. Most of the rock surface is covered with secondary k-feldspar, epidote, and iron oxides (Figure (c,d&f)). Two types of muscovite are noted (Figure (b)); coarse-grain high anomalous interference colour crystals and fine muscovite minutes fill the interstitial spaces between the main rock-forming minerals. Except quartz, the essential and minor aluminosilicate minerals composed of pre-existing perthite granite are friable. Most of the grains are covered with clay minerals and iron oxides (Figure (c)).
Figure 7. Photomicrographs showing altered muscovite granite essential, accessory, and opaque minerals and their mutual relationships.
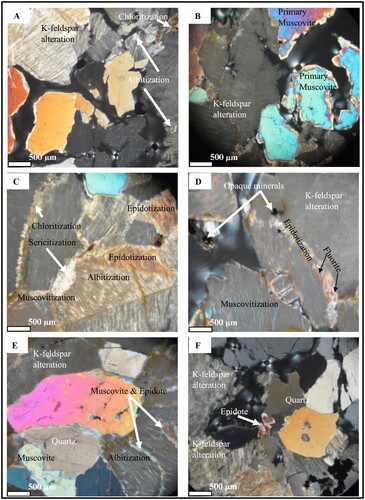
More than one generation of perthite has been recorded; subhedral coarse perthite grains covered with diamond-shaped secondary generated perthite. In this respect, perthite altered to coarse anhedral grains of microcline and orthoclase perthite. Furthermore, microcline perthite survives more against alteration than orthoclase perthite. Most of the fine-grain muscovite and sericite minerals occur most probably as alteration products of feldspars (Figure (c&d)).
An attack of orthoclase and/or anorthite by a hydrothermal solution produced clinozoisite and/or epidote (i.e. aluminium epidote), muscovite and quartz according to the following equation:
High anomalous fluorite crystals are entirelyenclosed within the epidotization zone and fill the rock cracks (Figure (d)). Biotite and hornblende fine-grain flakes are recorded as altered grains. Sphenoidal sphene fine euhedral grains are also recorded. Epidote occurs as coarse-grain euhedral crystals, while the opaque oxides or hydroxides are widespread all over the thin section (Figure (c–f)).
1.2.4. Pegmatite-aplite sheets
Aplitic granite is formed of rounded grains of quartz and interstitial alkali feldspar (Figure (a–c)), with sparse grains of iron ore and chlorite pseudomorphs after the primary mafic silicate minerals. Locally, quartz and alkali feldspar form micrographic intergrowths with around 50% quartz. The chilled margins of the aplite-dykes intersecting the alkaline granitic rocks have been noticed. Moreover, the rock unit is composed of quartz and alkali feldspar distributed in almost equal amounts. The aplite contains so many green spots of chlorite even in hand specimens. The pegmatoidal rock unit (Figure (d)) is massive, medium- to coarse-grained with tabular perthitic alkali feldspar and interstitial very coarse quartz grains. The interstitial micrographic quartz-feldspar intergrowths form around 50% quartz. Alkali feldspar commonly has clear rims of albite. Aegirine and bluish-green amphibole are recorded in the studied pegmatoidal granite. Meanwhile, recorded aegirine is generally replaced by chlorite, serpentine and pseudomorph relics. Magnetite, zircon, and fluorite are accessory minerals.
1.3. Materials and methods
Fifty bedrock samples were gathered from fresh alkali feldspar and altered granitic rocks. Fresh samples were collected from the main pluton of Jabal Al Bayda, while the altered granitic samples were collected near contact with Neogene basaltic rocks.
The polished thin section preparation and study were performed in the geology department, Taibah University. A total of 50 samples have been studied petrographically using a polarizing microscope. Moreover, 7 thin sections of fresh alkali feldspar and 5 thin sections of peralkaline granite were mineralogically allotted for modal classification. Twenty representative samples covering the different fresh and altered granitic rocks were petrographically selected for chemical analyses. Major and minor oxides, trace and REE elements were determined using the XRF technique at the laboratories of the Saudi Geological Survey, Saudi Arabia. Major oxides were determined from fused pellets, while trace elements were detected from powdered pellets. In this respect, analytical precision has been checked using international standards. The obtained results are accurate to within 3% for major oxides and better than 10% for trace elements. Loss of ignition (LOI) was determined by heating about 2 gm of the powdered sample in a porcelain crucible at 1100°C for constant weight.
2. Results
2.1. Geochemical signatures of the studied granites
Major and trace element distributions of Al Bayda granites are given in Table and Figure . The studied granitic rocks are enriched in SiO2 (72.95–75.76 Wt.%), Na2O (3.45–5.12 Wt.%), K2O (3.72–5.86 Wt.%), FeO*t/MgO ratio (3.05–10.80), Zr (280–731 ppm), Nb(43–82 ppm) and Y (45–82 ppm) with intermediate enrichment of total REE (up to 162 ppm). However, the studied granitic rocks are depleted in CaO, MgO, MnO, P2O5 and TiO2. The binary variation diagrams (Figure ) between major and trace elements show that (1) the peralkaline and alkali feldspar granite show a similar manner in most of their major and trace elements trends: (2) the peralkaline rocks are discriminated from the other granitic types; (3) reverse correlations between SiO2 and Al2O3, CaO, MgO, Fe2O3 and TiO2 (Figure ); (4) direct relation between Al2O3 and total alkali in alkali feldspar and peralkaline granites and (5) Hf, Nb and Ga contents are increased with increasing Zr content in unaltered granite, while the altered rock does not show any relation between Zr and other trace elements.
Figure 9. Variation diagrams of selected major and trace elements of Al Bayda fresh and altered younger granitoid rocks.
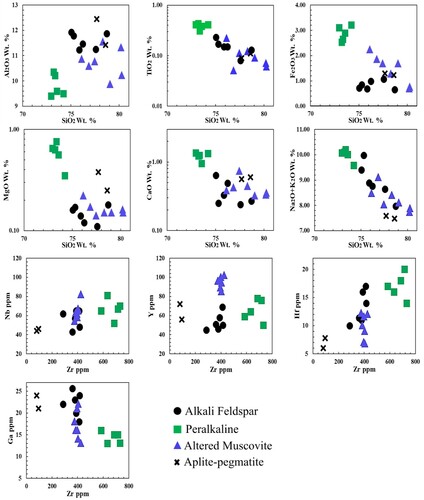
Table 3. Major (wt.%) and trace elements (ppm) abundance in Al Bayda fresh and altered granitoids.
2.2. Discussion
Based on the petrographic study, Al Bayda granitic pluton is classified into alkali feldspar granite, peralkaline granite, altered muscovite granite and pegmatite-aplite rock units. The geochemical discrimination of the magma type and tectonic setting of the investigated granites and the geochemical classification are determined mainly for the studied unaltered granites.
Geochemically, the studied altered granitic rocks are generally characterized by increases in SiO2, Fe2O3, alkali, Y, Nb and REE and decreases inTiO2, MgO and Al2O3 compared to the unaltered granitic body. The distribution patterns of certain elements of altered granite are given in Figure . However, the variation in minor and trace elements contained in these granites is significant. Except for zircon, the changes cannot be checked easily in terms of mineral composition. The breakdown of feldspars during metasomatic processes is largely responsible for the Al losses and the increase in Si [Citation16]. The K liberated by potassic alteration reactions is taken up by hydrothermal fluids, probably to generate second-stage K-metasomatism. Albitization is followed by incipient H+ metasomatism, usually characterized by the growth of sericite. Experimental work on silicate systems with volatile components and fluid inclusion studies of quartz from albitized granites can be used to estimate the temperature-pressure conditions of sodic metasomatism. In this respect, the results indicate temperatures between 400 and 6008C approximately, at pressures of 1 kbar or less [Citation17].
The studied fresh rocks are classified using the Alkali silica diagram of Middlemost ([Citation18], Figure (a)), and the R1–R2 projection [Citation19] that also has geotectonic and petrogenetic implications for granitoid suites (Figure (b)). Therefore, the studied alkali feldspar granite and peralkaline granite occupy the alkali felspar granitic field, while the pegmatite-aplite rocks are plotted in the normal granitic field (Figure (a)). Moreover, most of the studied granitoids are plotted in anorogenic to post-orogenic granitic fields (Figure (b)).
Figure 10. Classification, Magma type and tectonic setting of the studied granites. (a) Middlemost, [Citation18], (b) Batchelor & Bowden [Citation19], (c) Rickwood [Citation20], (d) Agapitic index (AI) vs. SiO2 diagram [Citation21]. The post-collision granite field is added by Farahat et al., [Citation22]., (e) Maniar and Piccoli [Citation23], (f&g) Whalen et al., [Citation24], (h) Pearce et al., [Citation25], VAG-volcanic arc granite, syn-COLG-syn collision granite, ORG-oceanic ridge granite, WPG-within plate granite. Symbols as Figure (9).
![Figure 10. Classification, Magma type and tectonic setting of the studied granites. (a) Middlemost, [Citation18], (b) Batchelor & Bowden [Citation19], (c) Rickwood [Citation20], (d) Agapitic index (AI) vs. SiO2 diagram [Citation21]. The post-collision granite field is added by Farahat et al., [Citation22]., (e) Maniar and Piccoli [Citation23], (f&g) Whalen et al., [Citation24], (h) Pearce et al., [Citation25], VAG-volcanic arc granite, syn-COLG-syn collision granite, ORG-oceanic ridge granite, WPG-within plate granite. Symbols as Figure (9).](/cms/asset/85560d79-1dd4-4f9d-9b07-7a083d63aa8d/tusc_a_2077615_f0010_oc.jpg)
According to the K2O vs. SiO2 diagram of Rickwood [Citation20], all the investigated granitoids display a high-K character (Figure (c)). More magmatic differentiation of the studied granite is obtained using SiO2- agpaitic index diagram of Liegeois and Black [Citation21]. In this diagram, most of the studied alkali feldspar granite and peralkaline granite are plotted in the alkaline to peralkaline domain, respectively (Figure (d)), while the pegmatite-aplite rocks are plotted in calc-alkaline meta-aluminous field. These results are generally in harmony with the petrographic examination. Furthermore, a plot of A/CNK versus A/NK [Citation23] reveals that most of the studied alkaline and peralkaline granites are plotted in a peralkaline field. Meanwhile, the studied aplitic pegmatitic rocks are plotted in a peraluminous field (Figure (e)).
Moreover, Whalen et al., [Citation24] suggested utilizing plots of Ga/Al versus certain major and trace elements to distinguish between M-, I-, S-, and A-type granitoids. The binary scatter diagrams 10,000 Ga/Al versus Nb and 10000 Ga/Al versus (Zr + Nb + Y+Ce) of Whalen et al. [Citation24] will be used to discriminate the A-type granites from the other types (I- S- and M-type granites). Except for the pegmatite-aplite rocks, the studied granitoid rocks are classified as alkali feldspar granite and exhibit hyper to subsolvous alkaline to peralkaline mineralogical characteristics within plate or intraplate magmatism A-type granite (Figure (f,g&h)).
As depicted in Table and Figure , the studied A-type granitoids have a pronounced depletion in CaO, MgO, and P2O5, and a marked enrichment in FeO*/MgO, Ga/Al, HFSE (Zr, Hf, Y, and Nb). These chemical aspects characterize post-orogenic and anorogenic silicic magmatism A-type granites [Citation24,Citation26–29]. In this regard, Eby [Citation28] used a trinary diagram of Ce, Y, and Nb to distinguish between anorogenic (A1-subtype) and post-orogenic granites (A2- subtype). On the Nb–Ce–Y diagram (Figure (a)), the peralkaline and 2 samples of alkali feldspar granites are plotted in the anorogenic field (i.e. A1, crust or arc sources). The anorogenic granites are formed by the partial melting of pre-existing sialic crust [Citation31]. Meanwhile, most alkali feldspar granitic samples are plotted in a post-orogenic field (i.e. A2). Moreover, the compositional differences of magmas are produced by partial melting of various source rocks, such as amphibolites, tonalitic gneisses, metagreywackes and metapelites, under variable melting conditions can be discriminated by their molar oxide content ratios. In this respect, Altherr et al. [Citation30] suggested a binary diagram of molar CaO/(MgO + FeO) versus molar Al2O3/MgO + FeOt to discriminate between different granitic sources. In this diagram, the peralkaline granite samples are plotted in the partial melt from the metagreywacke source (Figure (b)). Despite being located outside of the area proposed by Altherr et al. [Citation30], the alkali feldspar granites remain mysterious. Nevertheless, the present authors propose the partial melting of felsic rocks as the source of these granites (Figure (b)).
Figure 11. (a) Anorogenic and post-orogenic A-type granite discrimination diagram, Eby [Citation28]. (b) Granitic source binary diagram, modified after Altherr et al. [Citation30]. Symbols as Figure (9).
![Figure 11. (a) Anorogenic and post-orogenic A-type granite discrimination diagram, Eby [Citation28]. (b) Granitic source binary diagram, modified after Altherr et al. [Citation30]. Symbols as Figure (9).](/cms/asset/ede9e7dc-812c-4953-bda2-13d00cafa3e0/tusc_a_2077615_f0011_oc.jpg)
The variation of trace elements and REE is well defined by chondrite and primordial mantle normalized diagrams using the normalizing values of Sun & McDonough [Citation32]. Overall, the alkali feldspar and peralkaline granites show similar distribution patterns (Figures and ), and both show slight differences (i.e. 189–202 ppm) in total REE (including Y), which probably point to similar fractionation history. In all studied rocks, Eu is less than 1 ppm. Moreover, both fresh alkaline granites and altered granites are characterized by a smooth negative slope with LREE enrichment. Negative anomalies were observed for Eu, Li and Ti, with a moderate anomaly of K in all the studied rocks that may attribute to the fractionation of K-feldspar (K) and Fe-Ti oxides (Ti). The close similarity between alkali feldspar and peralkaline rocks in their REE chondrite normalized and REE primitive mantle normalized diagrams (Figures and ) indicate that both types have been generated from the same magma source. Moreover, the alkaline rocks depict a flat-HREE pattern without significant HREE fractionation. The alkali feldspar and peralkaline granites show enrichment in Zr, Nb, Hf and moderate anomaly in REE contents. Moreover, a distinctive comparable depletion in Li (under detection limit), Ti and Eu (Figure ). These characteristic features are related to plate granitoid geologic setting [Citation33]. Furthermore, the hydrothermal altered muscovite granites show a slight increase in the total REE compared to the fresh rocks (∼241 ppm).
Figure 12. Chondrite-normalized multi-elements diagram for the studied granites. Normalizing values from Sun and McDonough [Citation32]. Symbols as Figure (9).
![Figure 12. Chondrite-normalized multi-elements diagram for the studied granites. Normalizing values from Sun and McDonough [Citation32]. Symbols as Figure (9).](/cms/asset/c0dff339-4ad5-4d14-9843-3529ccc4ced4/tusc_a_2077615_f0012_oc.jpg)
Figure 13. Primitive mantle-normalized multi-elements diagrams for the studied granite. Normalizing values from Sun and McDonough [Citation32]. Symbols as Figure (9).
![Figure 13. Primitive mantle-normalized multi-elements diagrams for the studied granite. Normalizing values from Sun and McDonough [Citation32]. Symbols as Figure (9).](/cms/asset/30fc7e8f-0b39-4c19-a271-29f7e06502b8/tusc_a_2077615_f0013_oc.jpg)
2.2.1. A-type granite verification
Al Bayda fresh granite has high alkali contents(Na2O + K2–O = 7.97 to 10.20 wt%), high HFSE contents (Zr + Nb + Ce + Y = 423–947 ppm), high Fe2O3t/MgO ratios (up to 9.72), and high Ga/Al ratios. Al Bayda granites exhibit extreme depletion in Ca, HREE, P, Mn, Mg, Ti, Li, Co, Cr, Cu and Eu. Unlike A-type granites, however, Al Bayda granite characteristically has low to moderate ∑REE contents (121–162 ppm). The studied alkaline and peralkaline granites from Al Bayda are plotted in the A-type granite field in various chemical classification diagrams of 10,000 Ga/Al versus Nb, 10,000 Ga/Al versus (Zr + Nb + Ce + Y, Figure ). These geochemical signatures characterize A-type granites of Whalen et al., [Citation24], Loiselle & Wones [Citation34], Collins et al. [Citation35], and Eby [Citation27]. The chemistry is reflected by the occurrence of characteristic alkali mafic silicates, including amphiboles and/or pyroxenes (arfvedsonite and aegirine) in peralkaline rocks. Eby [Citation28] divided A-type granite into two groups: A1-type granite, which is related to hotspots, plums, or continental rift zone located in anorogenic setting, and A2-type granite, which is related to post-orogenic magmatism. According to the geochemical subdivision of A-type granites by Eby [Citation28], Al Bayda A-type alkali feldspar granite corresponds to the A2-type, while the peralkaline granite corresponds to the A1-type (Figure (a)). In this respect, A-type granites are generally derived from relatively anhydrous and high-temperature magmas [Citation34,Citation36]. Furthermore, the binary variation diagrams (Figure ), of major oxides show linear trends for alkaline and peralkaline granite, indicating that fractional crystallization plays an important role in the evolution of Al Bayda granite. Meanwhile, the samples are characterized by low MgO contents, which are due to the fractionation of MgO-rich minerals such as hornblende (see Petrography). A negative TiO2 anomaly is commonly related to ilmenite or sphene fractionation. CaO is mainly concentrated in plagioclase and apatite; Fe2O3 in magnetite. Therefore, the decrease in CaO and Fe2O3 with increasing SiO2 demonstrates that the studied granite was possibly produced by fractional crystallization of plagioclase and magnetite. The negative correlation between SiO2 and Al2O3 and the positive correlation between Al2O3 and alkali indicate that K-feldspar had been accumulated in the residual magma during the fractional crystallization of high-temperature magma. This permits Al2O3 to replace SiO2 producing feldspathoid minerals. Meanwhile, Chondrite-normalized REE patterns and primitive mantle-normalized trace element patterns (Figures and ) also support the magma fractionation during their evolution. In this respect, the studied alkali feldspar granite distribution patterns depict flat-HREE patterns without significant HREE fractionation. In addition, the recorded mafic amphiboles and pyroxenes in thin sections play an important role in producing intermediate and acidic magmas during fractionation processes [Citation37,Citation38].
2.2.2. Petrogenesis of the A-type granite
There is no consensus on the origin of A-type granites due to their huge compositional variations [Citation36]. A-type granite may be produced by direct fractionation of mantle-derived alkaline basalts [Citation39–41]; by partial melting of lower crustal granulite [Citation24,Citation35,Citation42,Citation43]; by anatexis of I-type tonalitic crustal sources [Citation44–47]; and/or by hybridization between anatectic granitic and mantle-derived mafic magmas [Citation48–51]. Nevertheless, the interaction of mantle-derived magma with crustal rocks and the melting of the deep continental crust are considered the most important mechanism [Citation52,Citation53]. Eby [Citation28] subdivided A-type granite into A1-type (M-type related granites) and A2-type (I-type related granites). According to Eby (op cit) A1-type granite was derived by the differentiation of oceanic island basaltic magma but emplaced in continental rift or during intraplate magmatism, while the A2-type granite was derived from the partial melting of the continental crustal or underplated crust. The studied peralkaline anorogenic A1 type and the alkali feldspar post-orogenic A2 type granite (Figure (a)) have low A/CNK, high content of Y, absence of basic and intermediate microgranular enclave, flat HREE pattern (i.e. no garnet minerals). Furthermore, the absence of large volumes of mafic-intermediate rocks from the study area does not support the extensive mafic magma fractional crystallization theory. Thus, the direct fractionation of mantle-derived alkaline basalts and partial melting of lower crustal granulite in a separate manner is completely ruled out. Considering the role of magmatic mingling (see petrography) of anhydrous high-temperature magma in time and space, field relationships (Figure ), tectonic regime affecting the study area (i.e. Najd fault system), petrographic observations (i.e. recording of feldspathoid, mafic, sodic mafic silicate, and fluoride minerals), and the previous discussion on the geochemical signature of the studied granitic rocks, encourage the authors to exclude a monogenetic source and believe in hybrid genetic source for the studied peralkaline and alkali feldspar granite.
Based on the obtained data and the previous discussion, the studied peralkaline granite is likely produced as a mix by the partial melting of pre-existing metavolcanosedimentary (see [Citation35]) country rocks (e.g. metagreywacke) and mantle-derived magma injection in the highly deformed crust. Similarly, the studied alkali feldspar granite was produced by the interaction of mantle-derived upwelling magma with pre-existing felsic igneous rocks that were recorded on the periphery of the study area. Overall, Al Bayda A-type granite was derived from partial melting and fractionation of the Neoproterozoic crustal basement rocks by asthenospheric mantle injection through a crustal tear. The deformation of the crust and distribution of the alkaline rocks in the Al Bayda area is constrained by the Najd strike-slip fault system effect. However, changing the tectonic mechanism from post-orogenic (i.e., alkali feldspar granite) to anorogenic (i.e. peralkaline granite) is probably related to slab rollback in the opposite direction by the action of Najd fault system forces. In this respect, Robinson et al., [Citation54] concluded that Arabian Shield alkali feldspar (hypersolvous) granite with alkaline A-type geochemistry and alkali feldspar (hypersolvous) granite with a distinctive peralkaline (i.e. Na mafic mineral-bearing) A-type signature are probably related to be products of late-orogenic slab tear/rollback inducing asthenospheric mantle injection and lower crustal melting/fractionation towards A-type/ferroan geochemistry.
2.2.3. Post-magmatic alteration
After the magmatic crystallization processes, the pre-existing mica granitic rocks were slightly altered during the late-magmatic stage, which was probably followed by successive hydrothermal alteration stages (see petrography for details). However, metasomatic activity pieces of evidence have been recorded as the most important processes during the alteration of studied altered muscovite granite. In this respect, K-feldspar alteration, chloritization, epidotization, hematite, and muscovitization have been introduced. Moreover, Na-metasomatism (i.e. albitization) is erratically distributed as white albite grains enclosed in alkali feldspar megacrysts. Meanwhile, most biotite mineral crystals are altered to chlorite iron oxide mixtures. Moreover, the recorded alkali feldspar exsolution textures are not primarily controlled by specific thermal events or cooling rates [Citation55]. In this respect, the abundance of exsolution textures in the studied altered granite indicates extensive structural rearrangement of magmatic alkali feldspar at low temperature (e.g. [Citation55–59]). In addition, the recorded subsolvus alkali feldspar has been developed isochemically by water-impelled dissolution and precipitation in a closed system [Citation4]. The infiltration of aqueous fluid is assured by the turbidity of alkali feldspar. The exsolution and alteration textures in the studied altered granite point to the probable presence of fluid for a long time after the crystallization of such granitoid.
Overall, this successive comagmatic-hydrothermal alterations in a closed system and post metasomatic processes along with the major and minor structures are the main factors controlling the concentration of HFSE and the depletion in other major and minor elements. Notwithstanding the content of HFSE, Nb and REE show high to moderate enrichment content, the main bulk of the ore metals is probably precipitated during migration along with the faults and other fracture zones.
2.2.4. Nb-Zr-REE enrichment
The Studied Alkali feldspar, peralkaline granite, altered muscovite granite and pegmatite-aplite sheets in the Al Bayda area exhibited high anomalous content in Zr (avg. 374, 699, 391 and 88 ppm, respectively), Nb (avg. 57, 67, 60 and 45 ppm, respectively), Y (avg. 53, 65, 97 and 64 ppm, respectively) and moderately anomalous in total REE (avg. 134, 137, 145 and 76 ppm, respectively). However, by comparing the Nb content in the studied granitoids with those in other related publications (e.g. [Citation43,Citation60]), it is important to note that the studied granitoid rock units cannot be considered granite-related Nb deposits. On the other hand, the obtained petrographic and geochemical results and previous discussions, the studied alkaline rock enrichments with these metals are related to partial melting of pre-existing rocks during magmatic fractionation and mingling with the original magma. The concentration, either in rock-forming minerals or in their mineral species, is mainly dependent on the original magma composition and the nature of the source regions where partial melting occurred [Citation61]. In this respect, these elements may enter the lattice of rock-forming minerals (e.g. mica and hornblende) that have crystallized in the subsolidus range of pressure and temperature. The processes that lead to the enrichment of these elements are linked with the activity of residual alkali-rich fluids. Moreover, hydrothermal alteration plays an important role in the redistribution and concentration of these metals in altered muscovite granite. In this respect, increasing the content of alkali and fluorine (i.e., fluorite in thin section) carrying solution increases the solubility of HFSE to form soluble alkali fluoride silicate complex [Citation35,Citation62,Citation63] especially in the late stage of granitic melt evolution [Citation4]. Shcherba [Citation64], noting that plagioclase and mica are the principal “carriers of rare metals”, the leaching of the metallic elements from their original sites in the lattices of these rock-forming minerals during alteration processes is controlled by the presence of F and Cl species in the fluids. In this respect, Cerny et al. [Citation65] stated that Nb content changes greatly during pegmatite evolution due to the higher fluorine-based thermal stability of the complexes, which makes Nb available for crystallization in the early stage.
3. Conclusion
Al Bayda granitic pluton is considered one of the most significant case studies of felsic alkaline and peralkaline as well as their related co-magmatic hydrothermal altered granitic rocks in Hijaz Terran, western Arabian Shield. The three studied granitoids are intruded by pegmatite-aplite rocks. Alkali feldspar and peralkaline granites are considered A-type granite, which is generated within a plate-related tectonic setting and is divided into A2 post-orogenic-type granite (i.e. alkali feldspar) and A1 anorogenic (peralkaline)-type granite. These alkaline hypersolvous A-type granitic rocks are probably products of late-orogenic slab tear/rollback inducing asthenospheric mantle injection and lower crustal melting/fractionation towards A-type geochemistry. Changing of tectonic mechanism from post-orogenic (i.e.,, alkali feldspar granite) to anorogenic (i.e. peralkaline granite) might be related to slab rollback in the opposite direction by Najd fault system forces. The alkali metasomatic altered epidotized, muscovitized granites were generated during a late-magmatic stage that is probably followed by successive comagmatic-hydrothermal alteration in a close system. The HFSE, Nb and REE enrichments are produced by metasomatic alteration. The processes that lead to the enrichment of these elements are linked with the activity of residual alkali-rich fluids, and the main bulk of the ore metals are transported in the form of fluoride complex and deposited as a result of iron-rich fluid mixing during migration along with the faults and other fracture zones. Mineralogical and geochemical analyses of the studied granitoid placer deposit products are recommended before processing this feldspar sand as ceramic raw materials to discover and remove any radioactive carrying minerals such as zircon. The recorded HFSE, Nb and REE are recommended for detailed exploration.
Acknowledgements
The authors would like to thank the geological survey of Saudi Arabia for the geochemical analyses. Thin-section preparation and microscopic examinations are conducted under the umbrella of the Geology Dept., Taibah University. The authors owe special thanks to A. Al oufi and H. Turkustani, Taibah University, for their help in sampling and thin section preparation. The authors thank an anonymous reviewer for constructive criticisms of an earlier version of the manuscript.
Disclosure statement
No potential conflict of interest was reported by the author(s).
References
- Elliott JE. Peralkaline and peraluminous granites and related mineral deposits of the Arabian Shield. Saudi geol. Surv, KSA; 1983.
- Stern RJ. Arc assembly and continental collision in the Neoproterozoic East African Orogen: implications for the consolidation of Gondwanaland. Ann Rev Ear Plan Sci. 1994;22:319–351.
- Stern RJ. Crustal evolution in the East African Orogen: a neodymium isotopic perspective. J Afri Ear Sci. 2002;34:109–117.
- Qadhi TM. Origin and hydrothermal alteration of rare-metal granites in the Al-Hamra area, northeastern Arabian Shield, Saudi Arabia. Cen Euro Geol. 2007;50(3):259–282.
- Stoeser DB. Distribution and tectonic setting of plutonic rocks of the Arabian Shield. J Afri Ear Sci. 1986;4:21–46.
- Gougazeh M, Bamousa A, Hasan A. Evaluation of granitic rocks as feldspar source: Al Madinah, Western Part of Saudi Arabia. J Taibah Univ Sci. 2018;12(1):21–36.
- Drysdall AR, Jackson NJ, Ramsay CR, et al. Rare earth mineralization related to Precambrian alkali granites in The Arabian Shield. Econ Geol. 1984;79:1366–1377.
- Ramsay CR, Drysdall AR, Clark MD. Felsic plutonic rocks of the Midyan region, Kingdom of Saudi Arabia – I distribution, classification, and resource potential. J Afri Ear Sci. 1986;4:63–77.
- Jackson NJ. Distribution, nature, and origin of trondhjemite in the Arabian Shield. A Reconn Surv. 1986;4:99–103.
- Qadhi TM. Petrogenesis and mineralization of the Ghurayyah pluton, Midyan, Northeast Saudi Arabia [PhD Thesis]. King Abdulaziz University, Jeddah; 1990.
- Pellaton C. Geologic map of the Al Madinah quadrangle, sheet 24 D, Kingdom of Saudi Arabia. S Arab Dep, Mins Min Res, Geo Map GM-52. 1981, 19 p.
- Galen F, Brown D, Schmidt L, et al. Geology of the Arabian Peninsula Shield Area of Western Saudi Arabia, U.S. G.S. Profess Paper, 560-a; 1990, 198 p.
- Johnson PR. Explanatory notes to the map of proterozoic geology of western Saudi Arabia. Saudi Geol Surv. 2006: Rep SGS-TR-2006-4.
- Kemp J, Pellaton C, Calvez JY. Geochronological investigations and geologic history in the Precambrian of north-western Saudi Arabia. Saudi Arab Dep Min Res. 1980: Report BRGM-of-01-1, 120.
- Streckeisen AL. To each plutonic rock its propername. Earth Sci. Rev. 1976;12:1–33.
- Kinnaird JA, Batchelor RA, Whitley JE, et al. Geochemistry, mineralization, and hydrothermal alteration of the Nigerian high heat producing granites. In: High heat production (HHP) granites, hydrothermal circulation, and ore genesis. Inst of Min Metal, Lon. 1985:169–195.
- Pollard PJ. Magmatic and post-magmatic processes in the formation of rocks associated with rare element deposits. Tra Inst Min Meta. 1983;92:B1–B9.
- Middlemost EAK. Magmas and magmatic rocks. London: Long; 1985.
- Batchelor RA, Bowden P. Petrogenetic interpretation of granitoid rocks series using multicationic parameters. Chem Geol. 1985;48:43–55.
- Rickwood PC. Boundary lines within petrologic diagrams which use oxides of major and minor elements. Lithos. 1989;22:247–263.
- Liégeois JP, Black R. Alkaline magmatism subsequent to collision in the Pan African belt of the Adrar des Iforase. In: Fitton, JG, Upton, B GJ Ed., Alkaline igneous rocks. Geol Soc. 1987;30:381–401.
- Farahat ES, Mohamed HA, Ahmed AF, et al. Origin of I- and A-type granitoids from the Eastern Desert of Egypt: implications for crustal growth in the northern Arabian–Nubian Shield. J. Afri Ear Sci. 2007;49:43–58.
- Maniar PD, Piccoli PM. Tectonic discrimination of granitoids. Geol. Soc. of Amer Bull. 1989;101:635–643.
- Whalen JB, Currie K, Chappell B. A-type granites: geochemical characteristics, discrimination and petrogenesis. Contr Min Petr. 1987;95:407–419.
- Pearce JA, Harris NBW, Tindle AG. Trace element discrimination diagrams for the tectonic interpretation of granitic rocks. J Petrol. 1984;25:956–983.
- Chappell WB, White AJ, Wyborn D. The importance of residual source material (restite) in granite petrogenesis. J Petro. 1987;28:1111–1138.
- Eby GN. The A-type granitoids: A review of their occurrence and chemical characteristics and speculations on their petrogenesis. Lithos. 1990;26:15134.
- Eby GN. Chemical subdivision of the A-type granitoids: petrogenetic and tectonic implications. Geol. 1992;20:641–644.
- Whalen JB, Jenner GA, Longstaffe FJ, et al. Geochemical and isotopic O, Nd, Pb and Sr constraints on A-type granite petrogenesis based on the Topsails Igneous Suite Newfoundland Appalachians. J Petr. 1996;37:1463–1489.
- Altherr R, Holl A, Hegner E, et al. High potassium, calc-alkaline I-type plutonism in the European Variscides: northern Vosges (France) and northern Schwarzwald (Germany). Lithos. 2000;50:51–73.
- Nardi LVS, Bonin B. Post-orogenic and non-orogenic alkaline granite associations: the Saibro intrusive suite, southern Brazil – a case study. Chem Geol. 1991;92:197–211.
- Sun SS, McDonough WF. Chemical and isotopic systematics of oceanic basalts: implication for mantle composition and processes. In: Saunders AD, Norry MJ, editor. Magmatism in the ocean basins. Geological Society Special Publication, no 42; 1989. p. 313–345.
- Brown GC, Thorp RS, Webb PC. The geochemical characteristics of granitoids in contrasting arc comments on magma source. J Geo Soc Lon. 1984;141:413–426.
- Loiselle MC, Wones DR. Characteristics and origin of anorogenic granites; Abstracts of papers to be presented at the Annual Meetings of the Geological Society of America and Associated Societies, San Diego, California, 11, Nov. 5–8, 468; 1979.
- Collins WJ, Beams SD, White AJR, et al. Nature and origin of A-type granite with reference to southeastern Australia. Contrib Min Petro. 1982;80:189–200.
- Bonin B. A-type granites and related rocks: evolution of a concept, problems, and prospects. Lithos. 2007;97:1–29.
- Martin H, Bonin B, Capdevila R, et al. The Kuiqi peralkaline granitic complex SE China petrology and geochemistry. J Petrol. 1994;35:983–1015.
- Han BF, Wang SG, Jahn BM, et al. Depleted mantle source for the Ulungur River A-type granites from North Xinjiang, China: geochemistry and Nd–Sr isotopic evidence, and implications for Phanerozoic crustal growth. Chem Geol. 1997;138:135–159.
- Turner SP, Foden JD, Morrison RS. Derivation of some A-type magmas by fractionation of basaltic magma: an example from the Padthaway Ridge, South Australia. Lithos. 1992;28-2:151–179.
- Litvinovsky BA, Jahn B, Zanvilevich AN, et al. Petrogenesis of syenite granite suites from the Bryansky Complex Transbaikalia, Russia: implications for the origin of A-type granitoid magmas. Chem Geol. 2002;189:105–133.
- Mushkin A, Navon O, Halicz L, et al. The petrogenesis of A-type magmas from the Amram Massif, Southern Israel. J Petrol. 2003;44-5:815–832.
- Clemens JD, Holloway JR, White AJR. Origin of an A-type granite: experimental constraints. Am Min. 1986;71:317–324.
- King PL, White AJR, Chappell BW, et al. Characterization and origin of aluminous A-type granites from the Lachlan Fold Belt, Southeastern Australia. J Petr. 1997;38(3):371–391.
- Sylvester PJ. Post-collisional alkaline granites. J Geol. 1989;97:261–280.
- Creaser RA, Price RC, Wormald RJ. A-type granites revisited: assessment of a residual-source model. Geol. 1991;19:163–166.
- Skjerlie KP, Johnston AD. Vapor-absent melting at 10 kbar of a biotite-and amphibole-bearing tonalitic gneiss: implications for the generation of A-type granites. Geol. 1992;20:263–266.
- Patiňo-Douce AE. Generation of metaluminous A-type granites by low-pressure melting of calc-alkaline granitoids. Geol. 1997;25:743–746.
- Kerr A, Fryer BJ. Nd isotope evidence for crust-mantle interaction in the generation of A-type granitoid suites in Labrador, Canada. Chem Geol. 1993;104(1–4):39–60.
- Wickham SM, Alberts AD, Zanvilevich AN, et al. A stable isotope study of anorogenic magmatism in East Central Asia. J Petr. 1996;37(5):1063–1095.
- Yang JH, Wu FY, Chung SL, et al. A hybrid origin for the Qianshan A type granite, northeast China: geochemical and Sr–Nd–Hf isotopic evidence. Lithos. 2006;89(1–2):89–106.
- Zhao KD, Jiang SY, Yang SY, et al. Mineral chemistry trace elements and Sr–Nd–Hf isotope geochemistry and petrogenesis of Cailing and Furong granites and mafic enclaves from the Qitianling batholith in the Shi-Hang zone, South China. Gond Res. 2012;22:310–324.
- Rämö OT, Haapala I. One hundred years of rapakivi granite. Min Petro. 1995;52:129–185.
- Bonin B. A-type granite ring complexes: mantle origin through crustal filters and the anorthosite-rapakivi magmatism connection. In: Demaiffe D, editor. Petrology and geochemistry of magmatic suites of rocks in the continental and oceanic crusts. Brux: ULB-MRAC; 1996. p. 201–218.
- Robinson FA, Bonin B, Peasel V, et al. A discussion on the tectonic implications of Ediacaran lateto post-orogenic A-type granite in the northeastern Arabian Shield, Saudi Arabia. Amer Geoph Uni Publ. 2017, 19 p.
- Parsons I. Feldspars and fluids in cooling plutons. Min Mag. 1978;42:1–17.
- Brown WL, Parsons I. Alkali feldspars: ordering rates, phase transformations and behavior diagrams forigneous rocks. Min Mag. 1989;53:25–42.
- Worden RH, Walker FDL, Brown WL. Development of microporosity, diffusion channels and deuteric coarsening in perthitic alkali feldspars. Contr Min Petr. 1990;104:507–515.
- Lee MR, Waldron KA, Parsons I. Exsolution and alteration microtextures in alkali feldspar phenocrysts from the shape granite. Min Mag. 1995;59:63–78.
- Walker FDL, Lee MR, Parsons I. Micropores and micropermeable texture in alkali feldspar: geochemical and geophysical implications. Min Mag. 1995;59:505–534.
- Collins WJ, Huang H, Bowden P, et al. Repeated S–I–A-type granite trilogy in the Lachlan Orogen and geochemical contrasts with A-type granites in Nigeria: implications for petrogenesis and tectonic discrimination. Geo Soc Lon. 2019. Available from: https://www.researchgate.net/publication/332853776.
- Pirajno F, Ernst RE, Borisenko AS, et al. Intraplate magmatism in Central Asia and China and associated metallogeny. Ore Geol Rev. 2009;35:114–136.
- Watson EB. Zircon saturation in felsic liquids: experimental data and application to trace element geochemistry. Contr Min Petr. 1979;70:407–419.
- Collerson KD. Geochemistry and Rb-Sr geochronology of associated Proterozoic peralkaline and subalkaline anorogenic granites from Labrador. Contrib. Min Petro. 1982;81:126–147.
- Shcherba GN. Greisens. Int Geol Rev. 1970;12:114–255.
- Cerny P, Meintzer RE, Anderson AJ. Extreme fractionation in rare-element granitic pegmatites: selected examples of data and mechanisms. Can Min. 1985;23:381–421.