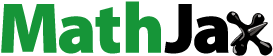
ABSTRACT
Inflammatory diseases are normally treated with non-steroidal anti-inflammatory drugs which may cause adverse effects if used for prolonged periods. Thus, there is a need to search for alternative anti-inflammatory medicine. This study investigated the phytochemical characteristics, cytotoxic, and anti-inflammatory activities of C. iria Linn. roots ethanol extract. The extract was subjected to preliminary phytochemical screening using standard procedures. The anti-inflammatory activities were determined using the egg albumin denaturation assay and the human red blood cell membrane stabilization method while the cytotoxic activity was investigated using the brine shrimp lethality assay. Preliminary phytochemical screening revealed the presence of flavonoids, phenolic acids, terpenoids, and saponins in the extract. Anti-inflammatory assays revealed that the extract has comparable results with the positive control (250 µg/ml Celecoxib) while being non-toxic. These results suggest that the synergetic actions of identified phytochemicals may contribute to the anti-inflammatory effect on egg albumin and human red blood cells.
1. Introduction
Inflammation is a natural and complex process associated with vascular permeability, membrane alteration, and an increase in protein denaturation. Immune cells play important roles in inflammatory responses and are activated by the release of chemical mediators from damaged tissues [Citation1]. However, if this process is not properly directed, inflammation can result in more adverse tissue damage leading to allergy, rheumatoid arthritis, or pancreatic and prostate cancers [Citation2,Citation3]. Inflammation is commonly medicated with either steroidal anti-inflammatory drugs (SAIDs) or non-steroidal anti-inflammatory drugs (NSAIDs). However, most of these drugs are expensive and may cause gastrointestinal (GI) and renal adverse effects in long-term use [Citation4]. GI adverse effects may include minor symptoms such as heartburn, dyspepsia and nausea, and serious gastrointestinal hemorrhaging. Renal adverse effects include the inability to detoxify the blood and some serious kidney diseases [Citation5]. Thus, there is an increasing interest in discovering new non-toxic, anti-inflammatory agents that originate from natural products [Citation6].
The role of cytotoxicity test in the development of drugs is very important, it is a critical factor in drug development from natural products [Citation7]. Cytotoxicity studies are widely used for the toxicity of substances and drug screening. It is an important initial study in determining the tolerable level of a substance such as natural products in cells. Substances may have different cytotoxic mechanisms including cell lysis, malfunctioning of binding receptors, and protein synthesis inhibition [Citation8,Citation9]. Cytotoxic assays are widely used for preliminary in vitro toxicity screening before further experiments on animal models. Testing the cytotoxicity level of a natural product is an initial step in developing it into an alternative medically important substance [Citation10]. Thus, there is a need to look for alternative medicine which is non-toxic, has fewer adverse side effects, and is yet effective.
Natural products are an important source of novel drugs for the treatment of many diseases [Citation11–15]. Plants that are known to have medicinal value have potential applications in biomedical [Citation16–18]. Nowadays, most pharmaceutical drugs originated from traditional alternative medicine [Citation19]. The Food and Drug Administration (FDA) approved more than one-third (39.1%) of drugs that are of natural origin [Citation20]. Up to this date, natural products are still important sources of drug discovery [Citation21–24]. The diversity of secondary metabolites illustrates the importance of natural products for the development of new drugs [Citation25]. The ability of plants to develop a complex defense system resulted in a complex range of chemicals. This plant’s ability makes it one of the important sources of novel active compounds that have pharmaceutical importance [Citation26]. Compared to NSAIDs and SAIDs, natural products are believed to be reasonable, environment-friendly, cost-efficient, and have fewer adverse side effects [Citation27].
Traditionally, Cyperus plants had been used as a remedy to treat different human ailments involving digestive and inflammatory diseases [Citation28]. Several Cyperus species such as Cyperus rotundus [Citation29,Citation30], Cyperus esculentus L. [Citation31], and Cyperus articulatus [Citation32] had been proven to have medicinal value due to the presence of different bioactive constituents. This only proved that several Cyperus spp. had promising pharmacological effects and biological activities that may be beneficial for various disease treatments [Citation33]. Cyperus iria Linn. (C. iria Linn.), or payong-payong, is a common annual sedge weed belonging to the same family. It is widely distributed on irrigated rice fields in the different parts of the Philippines and is considered an invasive species that reduce crop yield and quality [Citation34,Citation35]. But in traditional Chinese medicine, C. iria Linn. has been used as a treatment for fracture and rheumatism [Citation36]. A review of the phytochemical composition of Cyperus spp. revealed that several of its members are rich in biological compounds that exhibited pharmacological properties [Citation33] and this may also be present in C. iria Linn. Currently, there is no published research on the anti-inflammatory and cytotoxic activities of C. iria Linn.
The phytochemical properties of C. iria Linn. have not been reported except for the presence of terpenoids [Citation36]. However, several species from the Cyperus genus have well-documented phytochemistry [Citation33]. Cyperus species such as C. rotundus, and C. esculentus L. have been found to harbor the following phytochemicals: alkaloids, flavonoids, phenols, and tannins [Citation37,Citation38]. Thus, the chances of these phytochemicals being present in C. iria Linn. is likely to be high. The presence of phytochemicals, cytotoxic effects, and anti-inflammatory potential of the C. iria Linn. roots ethanol extract (CREE) are determined in this study. This research hopes to reduce the presence of C. iria Linn, an invasive weed in rice agriculture, by utilizing it as a possible source of alternative anti-inflammatory medicine. This study may also provide information to other researchers who have a scientific interest in C. iria Linn. particularly its anti-inflammatory and cytotoxic properties.
2. Materials and methods
2.1. Chemicals
Phosphate buffered saline (Sigma-Aldrich) and isotonic saline (0.9%) were obtained from local distributors. All the other chemicals used in the study were analytical grade and were readily available in the Chemistry Analytical Research Laboratory of Ateneo de Davao University.
2.2. Preparation of C. iria Linn. Roots Ethanol Extract (CREE)
The Cyperus iria Linn. (payong-payong) whole plant was collected from Maguindanao province, Philippines, and was authenticated at the Ateneo de Davao University (AdDU) Biological Collections, Philippines. The voucher specimen (AdDU2019.02) was kept in the AdDU Herbarium for future reference. The roots of C. iria Linn. plants were immediately washed with running tap water to remove dirt and other debris. The roots were cut into small pieces (1 cm) and were oven-dried at 40°C using a precision compact heating and drying oven (BIO-BASE BOV-T105F, Metro Manila, Philippines). Small pieces of rough dry roots were weighed using an analytical balance (Essae PG-34000 Precision Weighing Balance) every 24 h until a constant mass was reached. The oven-dried sample (250 g) was soaked in a 1.5 L ethanol solution (95%) for 48 h. The extract was filtered through Whatman filter paper No. 1 and was concentrated using a rotary evaporator (IKA RV-3, Selangor, Malaysia) set at 60°C. The crude ethanolic extracts of C. iria Linn. roots weighed 41 g.
2.3. Phytochemical screening
The presence of secondary metabolites such as flavonoids, tannins, phenolic acids, alkaloids, terpenoids, and saponins of the CREE was evaluated using the standard methods by Harborne [Citation39] and Guevarra [Citation40].
2.4. Egg albumin denaturation assay
CREE's in vitro anti-inflammatory potential was estimated using the egg albumin denaturation assay with some modifications as described by Alam et al. [Citation41]. This assay was conducted to determine if the CREE can inhibit protein denaturation which can cause inflammation [Citation2]. A mixture of 200 µL of egg albumin (from fresh hen’s egg), 2800 µL of phosphate-buffered saline (pH 6.4), and 2000µL of the samples or controls in different test tubes were prepared. These mixtures were incubated at 370C for 15 min, then heated in a water bath at 700C for 5 min. The mixtures were allowed to cool to room temperature for 5 min. Five hundred microliters (500 µL) of every mixture were transferred in their respective microwell on a 24-well plate. The absorbance of the samples was read at 660 nm using Epoch Microplate Spectrophotometer (Biotek, Winooski, USA). The experiment was conducted in triplicates and in three independent trials. The percent inhibition of protein denaturation was determined using the formula:
2.5. Human Red Blood Cell Membrane (HRBCM) stabilization method
HRBCM stabilization method was conducted to determine if CREE can protect cells from lysis which is one of the causes of inflammation [Citation42,Citation43]. Fresh whole human blood (5cc) was extracted by a licensed Medical Technologist from healthy volunteers who have not consumed any steroidal or non-steroidal anti-inflammatory drugs before the collection. The extracted blood was immediately transferred to heparin tubes and used for the bioassay. The collected blood sample was mixed in a conical tube with an equal volume of isotonic saline (0.9%) and was set aside for one minute to allow the blood cells to settle. The blood was centrifuged (Fisher Scientific, Waltham, USA) at 3000 rpm for 10 min and the supernatant was discarded. The packed cells were mixed with an equal volume of 0.9% isotonic saline (pH 7.2) for washing. This was done three times. The volume of the blood was determined and transformed as 10% v/v suspension with isotonic saline. The suspension was used immediately for the heat-induced hemolysis.
Heat-induced hemolysis was conducted by mixing 1000 µL of 0.15M phosphate buffer (pH 7.4), 2000µL isotonic saline (0.9% NaCl), 500 µL HRBC suspension (10% v/v), and 500 µL of the samples or controls in conical tubes. The mixtures were gently shaken and heated at 400C using an incubator for 60 min. The tubes were allowed to cool for 10 min and centrifuged at 1500 rpm for another 10 min. The resulting supernatant in every reaction mixture was transferred using a single channel adjustable pipette to its respective microwell plates. The absorbance was read at 560 nm using an Epoch Microplate Spectrophotometer (Biotek, Winooski, USA) to determine the percent inhibition of hemolysis of the human red blood cells. The experiment was conducted in triplicates and in three independent trials. Percent inhibition of hemolysis was determined using the formula:
2.6. Brine shrimp lethality assay
Brine shrimp lethality assay was conducted to determine the cytotoxicity of the different concentrations of CREE [Citation44]. A complete randomized design by drawing lots was applied in the assignment of samples and control. Three thousand microliters (3000 µL) of the different samples or control (artificial seawater) were placed in the respective well using a Pasteur pipette. Ten brine shrimps were pipetted in every microwell. The number of alive, impaired, and dead brine shrimps were counted after 30 min, 6, and 24 h of exposure to the samples and control. The experiment was conducted in five replicates and three independent trials.
2.7. Statistical analysis
All values for the anti-inflammatory assays were analyzed with ANOVA (SPSS vs. 26) followed by Tukey’s HSD post hoc test. The values of p < 0.05 were considered statistically significant. This test was used to determine if the results of the anti-inflammatory assays have significant differences compared to the results of positive control.
3. Results and discussion
3.1. Phytochemical screening
Phytochemical screening was conducted to evaluate the presence of secondary metabolites in CREE (Table ). The presence of flavonoids, phenolic acids, terpenoids, and saponins was confirmed using the Wilstatter “Cyanidin” test, ferric chloride test, Salkowski test, and froth test, respectively. On the other hand, tannins and alkaloids were absent when tested using the gelatin test and Dragendorff’s and Mayer’s test, respectively.
Table 1. Qualitative phytochemical constituents in C. iria roots ethanol extract.
3.2. Egg albumin denaturation assay
Figure shows the percent inhibition of protein denaturation of the different concentrations of CREE relative to the positive control (250 µg/mL Celecoxib). The observed IC50 is 434 µg/mL, indicating that this is the amount of concentration that inhibits the process in protein denaturation assay by half [Citation45]. Results (Table ) showed that 1000 µg/mL CREE exhibited comparable protein denaturation inhibition activity with the positive control (250 µg/mL Celecoxib) (p < 0.999). Similar to other Cyperus species such as C. rotundus [Citation29,Citation30, Citation89–91] and C. esculentus [Citation31], this implies that CREE may have a potent anti-inflammatory property [Citation33].
Table 2. The percent inhibition of protein denaturation and hemolysis of C. iria roots ethanol extract.
The potential of CREE to protect proteins from denaturation may be attributed to its flavonoid contents [Citation46]. Flavonoids possess a significant binding affinity for proteins [Citation47,Citation48]. The non-covalent attraction between protein and flavonoids may give rise to multiple hydrogen bonds that influence the strength of the formed protein-flavonoids complexes [Citation49,Citation50]. In the study of Zhang et al. [Citation51], it is indicated that proteins that interact with flavonoids have structures that are more conformationally open and flexible. Seczyk et al. [Citation52] emphasized that phenolic molecules can also link with different fragments of the polypeptide chain of protein molecules making it stable even if exposed to a stressor such as heat.
The presence of flavonoids in the CREE could also have potentially increased the thermal stability of the protein making it more stable. This observation is supported by several studies conducted [Citation53–56]. Particularly, studies conducted by Ali et al. [Citation53] and Hu et al. [Citation55], demonstrated that the thermal stability of proteins increased as a result of interaction with flavonoids. In the study conducted by Ali et al., [Citation53], the aggregation bands were not observed among complexes of the protein bovine serum albumin (BSA) and flavonoids. The inhibition of aggregation in BSA suggests that the hydroxyl groups of flavonoids can bind simultaneously at more than one site on this molecule preventing its unfolding [Citation57]. Higher thermal stability was also observed in the complexes of protein glycinin and flavonoids [Citation53,Citation58]. The denaturation temperature of glycinin increased in the presence of the flavonoids indicating conformational structural changes in the protein [Citation59–62], possibly resulting in the formation of protein-flavonoids complexes through hydrogen bonding and hydrophobic interaction.
The presence of saponins may also contribute to the anti-denaturation activity of CREE [Citation63,Citation64]. A study conducted by Grabowski et al. [Citation64], showed that saponins were potent inhibitors of BSA molecules that were denatured via increasing temperature. Another phytochemical that may also contribute to the inhibited denaturation of albumin is the terpenoids [Citation65,Citation66]. A study conducted by Truong et al. [Citation66] showed that terpenoids have the potential to inhibit the denaturation of protein albumin exposed to heat.
3.3. Human red blood cell membrane (HRBCM) stabilization method
The percent inhibition of hemolysis of the different concentrations of CREE relative to the positive control (250 µg/mL Celecoxib) is shown in Figure . In this assay, the observed IC50 is 355 µg/mL, indicating that this is the amount of concentration that inhibits the process in the HRBCM stabilization method by half [Citation45]. Results from the HRBCM stabilization method (Table ) showed that 1000 µg/mL CREE exhibited comparable percent inhibition of hemolysis with the positive control (250 µg/mL Celecoxib) (p < 0.900). This implies that CREE may have a potent anti-inflammatory property [Citation33].
The potential of CREE to inhibit hemolysis may be attributed to its flavonoid contents [Citation67,Citation68]. Some flavonoid contents such as naringenin, rutin, and genistein [Citation69] had been known to interact with lipid bilayers and can alter the membrane’s physicochemical properties [Citation70]. Flavonoids usually partition the unilamellar vesicles and decrease the membrane fluidity of cells [Citation70]. The decrease in the membrane fluidity in cells leads to an increase in membrane rigidity and strength, thus making the membrane more stable [Citation71]. Considering the structural similarity of human RBC and the lysosomal membranes, the results of this study suggest that CREE may stabilize lysosomal membranes thereby preventing the release of lysosomal components that may contribute to inflammatory responses [Citation72].
A possible mechanism in which plant extracts may inhibit membrane lysis is by reducing lipid peroxidation [Citation73–75]. The deterioration of lipids in plasma and the organellar membrane is due to the presence of free radicals [Citation76–80]. These free radicals such as oxygen-derived free radicals particularly the hydroxide (OH) may cause oxidative damage by linking to the double bonds in the unsaturated fatty acids [Citation81]. This interaction can produce unstable and reactive peroxide leading to an extensive membrane, organellar and cellular damage [Citation80]. Lipid peroxidation due to oxidative destruction of polyunsaturated fatty acids is harmful because it may alter the integrity of membranes [Citation82]. The presence of phenolic acids in CREE may reduce lipid peroxidation [Citation83–85]. Thus, phenolic acids can stabilize cell membranes by reducing lipid peroxidation [Citation86–88]. The presence of phenolic acids in CREE implies a potent reduction of lipid peroxidation as a possible mechanism for stabilizing membranes thereby preventing inflammatory responses.
The findings of this study suggest that CREE may have a potential source of anti-inflammatory compounds similar to other Cyperus species such as C. rotundus [Citation29,Citation30,Citation89–91] and C. esculentus [Citation31]. A study conducted by Fenanir et al. [Citation91], revealed that several essential oil molecules from the roots of C. rotundus may have potent inhibitory activity against 5-LO and LTA4H, which plays an important role in inflammatory responses. Since C. iria and C. rotundus are closely related, the chances of having similar compounds present in their system are possibly high [Citation92]. And to establish the potential of CREE for its anti-inflammatory activity, the Quantitative Structure–Activity Relationship (QSAR) and molecular docking of potential new active compounds towards acetylcholinesterase enzyme must also be considered in the future studies [Citation93–95].
3.4. Brine shrimp lethality assay
The percent mortality of brine shrimps after 24 h of exposure to the various CREE concentrations was shown in Figure . Results showed that the CREE concentrations are non-toxic since the rate of mortality of brine shrimps in all concentrations did not reach 50%. Data of BSLA shows an LC50 of 2250 µg/mL CREE concentration. The absence of alkaloids in CREE may have contributed to the non-toxicity of the extract. Alkaloids can penetrate the cell membrane of larval brine shrimps, thus causing damage and disrupting the membrane’s permeability leading to brine shrimps’ death [Citation96].
Figure 3. Percent (%) mortality of brine shrimps after 24 h of exposure to C. iria root ethanol extract
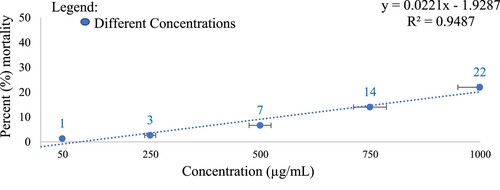
This low cytotoxicity of CREE is consistent with the data obtained from studies using other species of the genus Cyperus. In the study of Khojaste et al. [Citation97], results of the cytotoxicity test suggested that Cyperus rotundus tuber alcoholic extract showed no toxic effects on human gingival fibroblast (HGF) cells after 24 h of exposure to the samples while in the study of Hu et al. [Citation55], C. rotundus rhizome showed an effective decrease in the development of nicked DNA and elevated supercoiled form of DNA. This indicates a potential to prevent DNA damage in cells.
4. Conclusions
The CREE at a concentration of 1000 ug/mL showed potential anti-inflammatory activity using egg albumin denaturation assay and human red blood cell membrane stabilization method. The synergistic effect of the phytochemicals such as the flavonoids, phenolic acids, terpenoids and saponins in CREE may have contributed to its anti-inflammatory activity. Moreover, all the concentrations of CREE are non-toxic to brine shrimps. The non-toxicity of CREE may be attributed to the absence of alkaloids. Thus, this implies that CREE at 1000 ug/mL is non-toxic to cells and may have contributed to the stability of the protein albumin and stabilizes biological membranes, thus having a potent anti-inflammatory property. This discovery of the potential medicinal use of C. iria may elevate its value from being an agricultural pest to being pharmaceutically significant. For further studies, the actual mechanism of membrane stabilization and enhancement of protein stability by CREE be determined to verify further its anti-inflammatory property. The conduct of in vivo assays, such as the paw edema test and ear carrageenan test can be used to further test the anti-inflammatory property of CREE.
Disclosure statement
No potential conflict of interest was reported by the author(s).
References
- McKinley M, O’Loughlin D, Bidle T. Anatomy & Physiology: An Integrative Approach (2nd Ed.). McGraw-Hill Ed. New York, USA; 2016.
- Ullah HMA, Zaman S, Juhara F, et al. Evaluation of antinociceptive, in-vivo & in-vitro anti-inflammatory activity of ethanolic extract of Curcuma zedoaria rhizome. BMC Complement Altern Med. 2014 Oct 5;14(1):1–12.
- Sánchez-Quesada C, López-Biedma F, et al. Bioactive properties of the main triterpenes found in olives, virgin olive oil, and leaves of olea europaea. Vol. 61. J Agric Food Chem. 2013;50:12173–12182.
- Ojha D, Mukherjee H, Mondal S, et al. Anti-inflammatory activity of Odina wodier Roxb, an Indian folk remedy, through inhibition of toll-like receptor 4 signaling pathway. PLoS ONE. 2014 Aug 25;9(8):1–12.
- Wong RSY. Disease-Modifying Effects of Long-Term and Continuous Use of Nonsteroidal Anti-Inflammatory Drugs (NSAIDs) in Spondyloarthritis. Vol. 2019. Advances in Pharmacological Sciences. Hindawi Limited. 2019;2019:1–6.
- Chiu YJ, Huang TH, Chiu CS, et al. Analgesic and antiinflammatory activities of the aqueous extract from Plectranthus amboinicus (Lour.) Spreng. both in vitro and in vivo. Evidence-Based Complementary Altern Med. 2012;2012:1–12.
- Bácskay I, Nemes D, Fenyvesi F, et al. Role of Cytotoxicity Experiments in Pharmaceutical Development. In: Celik Tulay Askin, editor. Cytotoxicity. 1st. Aydin, Turkey: InTech; 2018. p. 131–146.
- Hamidi MR, Jovanova B, Panovska K. Toxicological evaluation of the plant products using Brine Shrimp (Artemia salina L.) model. Vol. 60. Macedonian pharmaceutical bulletin. 2014;1:9–18.
- Li W, Zhou J, Xu Y. Study of the in vitro cytotoxicity testing of medical devices. Biomed Rep. 2015 Sep;3(5):617–620.
- JM M ITK, M M PKG, SG K DKJ. Cytotoxicity of selected medicinal plants extracts using the brine shrimp lethality assay from Samburu county, Kenya. The Journal of Medical Research [Internet]. 2018 Oct;4(5):249–255. Available from: http://www.medicinearticle.com/JMR_20185_11.pdf
- Khan GA, War JA, Kumar A, et al. A facile synthesis of novel indole derivatives as potential antitubercular agents. Journal of Taibah University for Science. 2017 Nov;11(6):910–921.
- Soumya K, Haridas KR, James J, et al. Study of In vitro antioxidant and DNA damage protection activity of a novel luteolin derivative isolated from Terminalia chebula. Journal of Taibah University for Science. 2019 Dec 11;13(1):755–763.
- Alzahrani RR, Alkhulaifi MM, Alenazi NM, et al. Characterization and biological investigation of silver nanoparticles biosynthesized from Galaxaura rugosa against multidrug-resistant bacteria. Journal of Taibah University for Science. 2020 Jan 1;14(1):1651–1659.
- Gomaa MN, Almaghrabi OA, Elshoura AA, et al. Novel mixture of chloroxylenol and copper alters Candida albicans biofilm formation, biochemical characteristics, and morphological features. Journal of Taibah University for Science. 2020 Jan 1;14(1):889–895.
- Riaz MT, Yaqub M, Javed S, et al. In situ evaluation of the biological active poly functionalized novel amino-1,8-naphthyridine derivatives as DNA-electrochemical biosensor. Journal of Taibah University for Science. 2021 Jan 1;15(1):559–566.
- Gurnani N, Gupta M, Mehta D, et al. Chemical composition, total phenolic and flavonoid contents, and in vitro antimicrobial and antioxidant activities of crude extracts from red chilli seeds (Capsicum frutescens L.). Journal of Taibah University for Science. 2016 Oct;10(4):462–470.
- Adesina DA, Adefolalu SF, Jigam AA, et al. Antiplasmodial effect and sub-acute toxicity of alkaloid, flavonoid and phenolic extracts of Sida acuta leaf on Plasmodium berghei -infected animals. Journal of Taibah University for Science. 2020 Jan 1;14(1):943–953.
- Alhumaydhi FA. In vivo-analgesic, muscle relaxant, sedative and toxicological studies of Senna bicapsularis (L.) Roxb. Journal of Taibah University for Science. 2021 Jan 1;15(1):340–346.
- Ribeiro T, Lemos F, Preto M, et al. Cytotoxicity of portoamides in human cancer cells and analysis of the molecular mechanisms of action. PLoS ONE. 2017 Dec 1;12(12):1–18.
- Ivanz H, Boy A. Recommended Medicinal Plants as Source of Natural Products: A Review [Internet]. Vol. 1. Digital Chinese Medicine. 2018;1:131–142. http://dcmhi.com.cn.
- Patridge E, Gareiss P, Kinch MS, et al. An analysis of FDA-approved drugs: Natural products and their derivatives. Vol. 21. Drug Discovery Today. Elsevier Ltd. 2016;2:204–207.
- Yao H, Liu J, Xu S, et al. The structural modification of natural products for novel drug discovery. Vol. 12. Expert Opinion on Drug Discovery. Taylor and Francis Ltd. 2017;12:121–140.
- Bernardini S, Tiezzi A, Laghezza Masci V, et al. Natural products for human health: an historical overview of the drug discovery approaches. Vol. 32. Natural Product Research. Taylor and Francis Ltd. 2018;32:1926–1950.
- Zhang X, Bian G, Kang P, et al. Recent advance in the discovery of tyrosinase inhibitors from natural sources via separation methods. Vol. 36. Journal of Enzyme Inhibition and Medicinal Chemistry. Taylor and Francis Ltd. 2021;36:2104–2117.
- Svahn S. Digital Comprehensive Summaries of Uppsala Dissertations from the Faculty of Pharmacy 195. In 2015. Available from: http://urn.kb.se/resolve?urn=urn:nbn:se:uu:diva-242611
- Atanasov AG, Waltenberger B, Pferschy-Wenzig EM, et al. Discovery and resupply of pharmacologically active plant-derived natural products: A review. Vol. 33. Biotechnology Advances. Elsevier Inc. 2015;33:1582–1614.
- Samuelsson G, Bohlin L. Drugs of Natural Origin: A Textbook of Pharmacognosy. 5th ed. Sweden: Swedish Pharmaceutical Press,Stockholm; 2004.
- Udari LM. Medicinal Properties of Cyperus Species (Sedge Family, Cyperaceae) [Internet]. Available from: https://thekeep.eiu.edu/theses/4314
- Rocha FG, Brandenburg MdM, Pawloski PL, et al. Preclinical study of the topical anti-inflammatory activity of Cyperus rotundus L. extract (Cyperaceae) in models of skin inflammation. J Ethnopharmacol. 2020 May 23;254:1–10.
- Fenanir F, Semmeq A, Benguerba Y, et al. In silico investigations of some Cyperus rotundus compounds as potential anti-inflammatory inhibitors of 5-LO and LTA4H enzymes. J Biomol Struct Dyn. 2021;6:1–16.
- Udeffa AL, Amama AE, Archibong EA, et al. Antioxidant, anti-inflammatory and anti-apoptic effects of hydro-ethanolic extract of Cyperus esculentus L. tigernut) on lead acetate-induced testicular dysfunction in Wistar rats. Biomedicine & Pharmacotherapy. 2020;129:1–14.
- Rubert J, Monforte A, Hurkova K, et al. Untargeted metabolomics of fresh and heat treatment Tiger nut (Cyperus esculentus L.) milks reveals further insight into food quality and nutrition. J Chromatogr, A. 2017 Sep 8;1514:80–87.
- Nogueira ML, de Lima EJSP, Adrião AAX, et al. Cyperus articulatus L. (Cyperaceae) rhizome essential oil causes cell cycle arrest in the G2/M phase and cell death in HepG2 cells and inhibits the development of tumors in a xenograft model. Molecules. 2020 Jun 1;25(11):1–19.
- Taheri Y, Herrera-Bravo J, Huala L, et al. Cyperus spp.: A Review on Phytochemical Composition, Biological Activity, and Health-Promoting Effects. Vol. 2021. Oxidative Medicine and Cellular Longevity. Hindawi Limited. 2021;2021:1–17.
- Celedonio MA, Roble ND. Bioinvasion Assessment of Sedges: Cyperus Spp. in the Philippine Agricultural System [Internet]. Vol. 6. International Journal of Interdisciplinary Research and Innovations. 2018;6:109–118. www.researchpublish.com.
- Vicencnio EJ, Buot IE Weed research in asean countries: an annotated bibliography. Journal of Nature Studies. 2017;16(2):24–53.
- Jiang Y, Ownley BH, Chen F. Terpenoids from Weedy Ricefield Flatsedge (Cyperus iria L.) Are Developmentally Regulated and Stress-Induced, and have Antifungal Properties. Molecules. 2018 Nov 30;23(12):1–19.
- Ngoc QN, Minh TN. Cyperus rotundus cyperaceae: a study of phytochemistry, total polyphenol content, flavonoid content, and anti-oxidant activity. ES3 Web of Conferences. 2021;332:1–7.
- Prakash N, Ragavan B. Phytochemical observation and antibacterial activity of Cyperus esculentus L. Anc Sci Life. 2009;28(4):16–20.
- Harborne JB. Phytochemical methods: a guide to a modern technique in plant analysis. New York (NY): Chapman and Hall; 1993.
- Guevara B. Phytochemistry. A guidebook to plant screening: Phytochemical and Biological. Manila: UST Publishing House; 2005.
- Alam A, Jawaid T, Alam P. In vitro antioxidant and anti-inflammatory activities of green cardamom essential oil and in silico molecular docking of its major bioactives. Journal of Taibah University for Science. 2021 Jan 1;15(1):757–768.
- Anosike CA, Obidoa O, Ezeanyika LU. Membrane stabilization as a mechanism of the anti-inflammatory activity of methanol extract of garden egg (Solanum aethiopicum). DARU. J Pharm Sci. 2012;20(1):1–7.
- Bag A, Kumar Bhattacharyya S, Kumar Pal N, et al. Anti-inflammatory, anti-lipid peroxidative, antioxidant and membrane stabilizing activities of hydroalcoholic extract of Terminalia chebula fruits. Pharm Biol. 2013 Dec;51(12):1515–1520.
- Adoum OA, Dabo NT, Fatope MO. Bioactivities of some savanna plants in the brine shrimp lethality test and in vitro antimicrobial assay. International Journal of Pharmacognosy. 1997;35(5):334–337.
- Kikuchi R, Peterkin VC, Chiou WJ, et al. Validation of total IC50 method which enables in vitro assessment of transport inhibition under semi-physiological conditions. Xenobiotica. 2016;15(32):1–24.
- Ghamali M, Chtita S, Hmamouchi R, et al. The inhibitory activity of aldose reductase of flavonoid compounds: Combining DFT and QSAR calculations. Journal of Taibah University for Science. 2016 Oct;10(4):534–542.
- Papadopoulou A, Frazier RA. Characterization of protein-polyphenol interactions. Trends Food Sci Technol. 2004;15(3–4):186–190.
- Tang X, Tang P, Liu L. Molecular structure–Affinity relationship of Flavonoids in Lotus leaf (Nelumbo nucifera Gaertn.) on Binding to Human serum albumin and Bovine serum albumin by Spectroscopic Method. Molecules. 2017 Jul 1;22(7):1–11.
- Brudzynski K, Maldonado-Alvarez L. Polyphenol-protein complexes and their consequences for the redox activity, structure and function of honey. A current view and new hypothesis - A review. Vol. 65. Polish Journal of Food and Nutrition Sciences. Polish Academy Sciences. 2015;65:71–80.
- Ma CM, Zhao XH. Depicting the non-covalent interaction of whey proteins with galangin or genistein using the multi-spectroscopic techniques and molecular docking. Foods. 2019;8(9):1–13.
- Zhang H, Yu D, Sun J, et al. Interaction of plant phenols with food macronutrients: Characterisation and nutritional-physiological consequences. Vol. 27. Nutrition Research Reviews. Cambridge University Press. 2014;27:1–15.
- Seczyk L, Swieca M, Kapusta I, et al. Protein–phenolic interactions as a factor affecting the physicochemical properties of white bean proteins. Molecules. 2019 Jan 1;24(3):1–24.
- Ali H, Alli I, Ismail A, et al. Protein-Phenolic Interactions in Food. Vol. 7. Eurasian J Anal Chem. 2012;3:123–133.
- Song BJ, Manganais C, Ferruzzi MG. Thermal degradation of green tea flavan-3-ols and formation of hetero- and homocatechin dimers in model dairy beverages. Food Chem. 2015 Apr 15;173:305–312.
- Hu QP, Cao XM, Hao DL, et al. Chemical Composition, Antioxidant, DNA Damage Protective, Cytotoxic and Antibacterial Activities of Cyperus rotundus Rhizomes Essential Oil against Foodborne Pathogens. Sci Rep. 2017 Mar 24;7:1–9.
- Almatroodi SA, Almatroudi A, Anwar S, et al. Antioxidant, anti-inflammatory and hepatoprotective effects of olive fruit pulp extract: in vivo and in vitro study. Journal of Taibah University for Science. 2020 Jan 1;14(1):1660–1670.
- Dumitrascu L, Stănciuc N, Grigore-Gurgu L, et al. Investigation on the interaction of heated soy proteins with anthocyanins from cornelian cherry fruits. Spectrochimica Acta - Part A: Molecular and Biomolecular Spectroscopy. 2020 Apr 15;231:1–8.
- Rawel HM, Rte Czajka D, Rohn S, et al. Interactions of different phenolic acids and flavonoids with soy proteins [Internet]. Available from: www.elsevier.com/locate/ijbiomac
- Chen G, Wang S, Feng B, et al. Interaction between soybean protein and tea polyphenols under high pressure. Food Chem. 2019 Mar 30;277:632–638.
- Zhou SD, Lin YF, Xu X, et al. Effect of non-covalent and covalent complexation of (−)-epigallocatechin gallate with soybean protein isolate on protein structure and in vitro digestion characteristics. Food Chem. 2020 Mar 30;309:1–10.
- Quan W, He W, Qie X, et al. Effects of β-cyclodextrin, whey protein, and soy protein on the thermal and storage stability of anthocyanins obtained from purple-fleshed sweet potatoes. Food Chem. 2020 Aug 1;320:1–10.
- Tosif MM, Najda A, Bains A, et al. A comprehensive review on the interaction of milk protein concentrates with plant-based polyphenolics. Vol. 22. International Journal of Molecular Sciences. MDPI. 2021;22:1–20.
- Jang KJ, Ki Kim H, Han MH, et al. CytoAnti-inflammatory effects of saponins derived from the roots of Platycodon grandiflorus in lipopolysaccharide-stimulated BV2 microglial cells. Int J Mol Med. 2013 Jun;31(6):1357–1366.
- Grabowska K, Wróbel D, Żmudzki P, et al. Anti-inflammatory activity of saponins from roots of Impatiens parviflora DC. Nat Prod Res. 2020 Jun 2;34(11):1581–1585.
- Prakash V. Terpenoids as source of anti-inflammatory compounds. Vol. 10. Asian Journal of Pharmaceutical and Clinical Research. Innovare Academics Sciences Pvt. Ltd. 2017;10:68–76.
- Truong DH, Ta NTA, Pham TV, et al. Effects of solvent—solvent fractionation on the total terpenoid content and in vitro anti-inflammatory activity of Serevenia buxifolia bark extract. Food Science and Nutrition. 2021 Mar 1;9(3):1720–1735.
- Erlejman AG, Verstraeten Sv, Fraga CG, et al. The interaction of flavonoids with membranes: Potential determinant of flavonoid antioxidant effects. Free Radical Res. 2004 Dec;38(12):1311–1320.
- Tsuchiya H. Membrane interactions of phytochemicals as their molecular mechanism applicable to the discovery of drug leads from plants. Molecules. 2015 Oct 16;20(10):18923–18966.
- Arora A, Byrem TM, Nair MG, et al. (2000). Modulation of Liposomal Membrane Fluidity by Flavonoids and Isoflavonoids [Internet].. Available from: http://www.idealibrary.com
- Reis A, Perez-Gregorio R, Mateus N, et al. Interactions of dietary polyphenols with epithelial lipids: advances from membrane and cell models in the study of polyphenol absorption, transport and delivery to the epithelium. Critical Reviews in Food Science and Nutrition. Taylor and Francis Inc. 2020;18:1–24.
- Tarahovsky YS. Plant polyphenols in cell-cell interaction and communication. Plant Signaling and Behavior. 2008;3(8):609–611.
- Sabiu S, Ajani EO, Ajao AA, et al. Biomembrane stabilization and antiulcerogenic properties of aqueous leaf extract of Gossypium barbadense L. (Malvaceae). Beni-Suef University Journal of Basic and Applied Sciences. 2017 Dec;6(4):301–309.
- Awaad AS, Soliman GA, El-Sayed DF, et al. Hepatoprotective activity of Cyperus alternifolius on carbon tetrachlorideinduced hepatotoxicity in rats. Pharm Biol. 2012 Feb;50(2):155–161.
- Essaidi I, Koubaier HBH, Snoussi A, et al. Chemical Composition of Cyperus rotundus L. Tubers Essential Oil from the South of Tunisia, Antioxidant Potentiality and Antibacterial Activity against Foodborne Pathogens. Journal of Essential Oil-Bearing Plants. 2014;17(3):522–532.
- Adelakun SA, Ogunlade B, Fidelis OP, et al. Cyperus esculentus suppresses hepato-renal oxidative stress, inflammation, and caspase-3 activation following chronic exposure to sodium fluoride in rats’ model. Phytomedicine Plus. 2022 Feb 1;2(1):1–14.
- Mitamura JA, Seligman ML, Solomon JJ, et al. Loss of essential membrane lipids and ascorbic acid from rat brain following cryogenic injury and protection by methylprednisolone. Neurol Res. 1981;3(4):329–344.
- Sailaja Rao P, Kalva S, Yerramilli A, et al. Free Radicals and Tissue Damage: Role of Antioxidants. Free Radicals and Antioxidants. 2011 Oct;1(4):2–7.
- Slimen IB, Najar T, Ghram A, et al. Reactive oxygen species, heat stress and oxidative-induced mitochondrial damage. A review. Vol. 30. International Journal of Hyperthermia. Informa Healthcare. 2014;30:513–523.
- Çomu FM, Kılıç Y, Özer A, et al. Effect of picroside II on erythrocyte deformability and lipid peroxidation in rats subjected to hind limb ischemia reperfusion injury. Drug Des Devel Ther. 2016 Mar 1;10:927–931.
- Signorini C, Moretti E, Collodel G. Role of isoprostanes in human male infertility. Vol. 66. Systems Biology in Reproductive Medicine. Taylor and Francis Ltd. 2020;66(5):291–299.
- Silva Dd, Baião DdS, Ferreira VF, et al. Betanin as a multipath oxidative stress and inflammation modulator: a beetroot pigment with protective effects on cardiovascular disease pathogenesis. Vol. 62. Critical Reviews in Food Science and Nutrition. Taylor and Francis Ltd. 2021;62:539–554.
- Ayala A, Muñoz MF, Argüelles S. Lipid peroxidation: Production, metabolism, and signaling mechanisms of malondialdehyde and 4-hydroxy-2-nonenal. Vol. 2014. Oxidative Medicine and Cellular Longevity. Landes Bioscience. 2014;2014:1–31.
- Siquet C, Paiva-Martins F, Lima JLFC, et al. Antioxidant profile of dihydroxy- and trihydroxyphenolic acids - A structure-activity relationship study. Free Radical Res. 2006 Apr;40(4):433–442.
- Erukainure OL, Onifade OF, Odjobo BO, et al. Ethanol extract of Tetrapleura tetraptera fruit peels: Chemical characterization, and antioxidant potentials against free radicals and lipid peroxidation in hepatic tissues. Journal of Taibah University for Science. 2017 Nov;11(6):861–867.
- Abdelghffar EA, Barakat A, Torky ZA, et al. Pink Cedar (Acrocarpus fraxinifolius): its prophylactic role vs APAP–induced toxicity in rats and its antiviral activity vs HSV-1. Journal of Taibah University for Science. 2021 Jan 1;15(1):1108–1122.
- Maqsood S, Benjakul S, Abushelaibi A, et al. Phenolic Compounds and Plant Phenolic Extracts as Natural Antioxidants in Prevention of Lipid Oxidation in Seafood: A Detailed Review. Compr Rev Food Sci Food Saf. 2014 Nov 1;13(6):1125–1140.
- Adefegha SA, Oboh G. Antioxidant and inhibitory properties of Clerodendrum volubile leaf extracts on key enzymes relevant to non-insulin dependent diabetes mellitus and hypertension. Journal of Taibah University for Science. 2016 Oct;10(4):521–533.
- Ruiz-Ruiz JC, Matus-Basto AJ, Acereto-Escoffié P, et al. Antioxidant and anti-inflammatory activities of phenolic compounds isolated from Melipona beecheii honey. Food Agric Immunol. 2017 Nov 2;28(6):1424–1437.
- Srivastava RK, Singh A, Shukla Sv. Chemical Investigation and Pharmaceutical Action of Cyperus rotundus - A Review. Journal of Biologically Active Products from Nature. 2013 Jun 1;3(3):166–172.
- Tran HHT, Nguyen MC, Le HT, et al. Inhibitors of α-glucosidase and α-amylase from Cyperus rotundus. Pharm Biol. 2014 Jan;52(1):74–77.
- Fenanir F, Semmeq A, Benguerba Y, et al. In silico investigations of some Cyperus rotundus compounds as potential anti-inflammatory inhibitors of 5-LO and LTA4H enzymes. J Biomol Struct Dyn. 2021;2021:1–16.
- Chauhan BS, Johnson DE. The role of seed ecology in improving weed management strategies in the tropics. Advances in Agronomy. Academic Press Inc. 2010;105:221–262.
- Tarek L, el Houda HN, Yacine B, et al. Molecular Docking of New Active Compounds Towards the Acetylcholinesterase Enzyme. Current Research in Bioinformatics. 2019 Jan 1;8(1):18–20.
- Hammoudi NEH, Sobhi W, Attoui A, et al. In silico drug discovery of Acetylcholinesterase and Butyrylcholinesterase enzymes inhibitors based on Quantitative Structure-Activity Relationship (QSAR) and drug-likeness evaluation. J Mol Struct. 2021 Apr 5;1229:1–17.
- Hammoudi NEH, Benguerba Y, Attoui A, et al. In silico drug discovery of IKK-β inhibitors from 2-amino-3-cyano-4-alkyl-6-(2-hydroxyphenyl) pyridine derivatives based on QSAR, docking, molecular dynamics and drug-likeness evaluation studies. J Biomol Struct Dyn. 2022;40(2):886–902.
- Nerdy N, Lestari P, Sinaga JP, et al. Brine shrimps (Artemia salina Leach.) lethality test of ethanolic extract from Green Betel (Piper betle Linn.) and Red Betel (Piper crocatum Ruiz and Pav.) through the Soxhletation method for cytotoxicity test. Macedonian Journal of Medical Sciences. 2021;23(9(A)):407–412.
- Khojaste M, Yazdanian M, Tahmasebi E, et al. Effects of Cyperus rotundus extract Cell Toxicity and inhibitory effects of Cyperus rotundus extract on Streptococcus mutans, Aggregatibacter actinomycetemcomitans and Candida albicans. Vol. 28. Eur J Transl Myol. 2018;28(4):362–369.