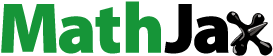
Abstract
One of the worst diseases, cancer claims millions of lives each year throughout the world, necessitating the creation of novel treatments. In this study, we designed a novel series of 1,3,4-thiadiazoles through the reaction of 2-(4-methyl-2-(2-(1-phenylethylidene)hydrazineyl)thiazole-5-carbonyl)-N-phenylhydrazine-1-carbothioamide (3) with the proper hydrazonoyl halides. Using the MTT assay, the newly synthesized thiadiazoles' growth-inhibitory potential against the liver cancer cell line HepG2-1 was assessed. In comparison to the standard drug doxorubicin (IC50 = 0.72 ± 0.52 µM), the results showed that two compounds, 16b and 21 (IC50 = 0.69 ± 0.41 and 1.82 ± 0.94 µM, respectively) had promising anticancer activity. The structural activity relationship (SAR) was investigated. In addition, molecular docking analysis onto quinone oxidoreductase2 (NQO2) receptor (PDB: 4ZVM) was investigated against the potent compounds to examine the reliability of the in vitro results. The newly prepared thiadiazole-thiazole hybrids are therefore regarded as potent anticancer drugs.
1. Introduction
Cancer is currently the second leading explanation for death worldwide [Citation1]. Over 90% of primary liver tumours are hepatocellular carcinomas (HCC), which are primary tumours of the liver. Of patients with cirrhosis, HCC occurs in about 85% of cases [Citation2]. HCC is currently the sixth most prevalent kind of cancer in the world, but it's also the third most frequent cause for cancer mortality [Citation3]. HCC is that the second greatest reason for cancer-related death in men, after carcinoma [Citation3]. HCC incorporates a 5-year survival rate of 18%, which is second only to carcinoma [Citation4]. Hepatitis (hepatitis B and C), alcoholic disease, and non-alcoholic steatohepatitis/non-alcoholic liver disease are all significant risk factors for hepatocellular cancer. HCC occurs in between 80% and 90% of cirrhotic patients. It is still a difficult task for medicinal chemists to research fresh leads for the development of novel structures that could be beneficial in developing more practical and fewer toxic new anticancer medicines. Medical researchers have focused on 1,3-thiazole and 1,3,4-thiadiazole systems in their quest to produce effective anticancer drugs.
In the process of creating new lead chemicals and developing new drugs, 1,3-thiazole derivatives were heavily taken into account. Thiosemicarbazones are the source of several pharmaceuticals that exhibit a broad range of pharmacological properties, such as, antiparasitic, anti-inflammatory, and anticancer activities [Citation5–12]. Thiazole derivatives are generated from thiosemicarbazones. Moreover, acid hydrazides were widely used for synthesis of many heterocyclic compounds as 1,2,4-triazoles, 1,3,4-oxadiazoles, 1,3,4-thiadiazoles, and 1,2,4,5-tetrazines [Citation13]. Inhibitors of myeloperoxidase, insecticides, glycogen phosphorylase, antituberculous medicines (isoniazid), and HIV are only a few of the biological functions that hydrazides exhibit [Citation14]. Recent studies on the synthesis of hydrazides directly from the corresponding acids without the use of any hazardous solvents or other support reagents like surfactants, as well as their confirmation using green chemical principles, have been reported [Citation15,Citation16].
According to several literature reviews, the 1,3,4-thiadiazole derivatives also have antioxidant, antidepressant, anti-cancer, anticonvulsant, anti-inflammatory, antibacterial, anti-leishmanial, and radio-protective activities [Citation17–19]. These significant biological properties prompted various research teams to explore various strategies for synthesizing novel thiadiazoles using several synthones, including thioacylhydrazines, acylhydrazines, dithiocarbazates, thiosemicarbazides, thiocarbazides, and bithioureas [Citation20].
As a follow-up to our studies [Citation6–8,Citation21–25] on the use of hydrazonoyl halides in the preparation of different bridgehead nitrogen polyheterocycles, we would like to present here a novel, simple method for producing a number of different functionalized 1,3,4-thiadiazoles derivatives having thiazole moiety as potent cytotoxic agents against liver cancer cell lines. Furthermore, GLIDE (Grid-based Ligand Docking with Energetics) of Schrödinger was used to explore the possible binding interactions of the most active derivatives onto the Protein quinone reductase-2 (4ZVM).
2. Results and discussion
2.1. Chemistry
Thiazole ester 1 [Citation26] could be converted into the corresponding acid hydrazide 2 by reacting with hydrazine hydrate in refluxing ethanol [Citation27] (Scheme 1).
As shown in Scheme 1, the reaction of carbohydrazide derivative 2 with phenyl isothiocyanate in EtOH produced the N-phenylhydrazine-1-carbothioamide derivative 3. The spectral data (IR, NMR, and ms) for compound 3 and its structure were in agreement.
The thiosemicarbazone derivative 3, which is produced when 1,3,4-thiadiazoles combine with hydrazonoyl chlorides, offers synthetic methods for producing useful heterocycles. Thus, a catalytic quantity of Et3N allowed compound 3 to react with hydrazonoyl chloride 4 in EtOH to yield a single product, which was recognized as the respective 1,3,4-thiadiazole derivative 6 (Scheme 1). The structure 6 was elucidated based on study of spectral and elemental data (see Experimental part).
The 1,3,4-thiadiazole derivative 6 was produced by cyclization through the elimination of the aniline molecule after the reaction was carried out by the nucleophilic displacement of the chlorine atom, which produced the thiohydrazonate intermediates.
Product 6's alternate technique provided more proof of the structure attributed to it. Thus, the hydrazine derivative 7 [Citation28] and ester 1 were allowed to react producing product 6 (Scheme 1).
Similarly, compound 3 was treated with 2-oxo-N'-arylpropanehydrazonoyl chlorides 9a,b to afford the respective 1,3,4-thiadiazoles 11a,b rather than 1,3-thiazole 12a,b (Scheme 2). 1,3,4-Thiadiazole 11 was formed by cyclizing the hydrazine-NH intramolecularly with an activated azomethine linkage of the intermediates 10, then eliminating the aniline molecule to give the respective 1,3,4-thiadiazole 11 (Scheme 2). Based on analytical and spectral investigations, products 11's structures were clarified. were elucidated (see Experimental part). As a typical example, the 1H-NMR spectrum of derivative 11a revealed five singlet signals at δ 2.30 (s, 3H, CH3), 2.42 (s, 3H, CH3), 2.46 (s, 3H, CH3), 10.26, 11.94 (2s, br, 2H, 2NH), as well as a multiplet signal at 7.31-7.54 assignable for ten aromatic protons. Additionally, the 13C-NMR spectrum showed two signals at δ 22.19 and 194.71 due to CH3CO group. The molecular ions are represented by a peak in the mass spectra of compounds 11a,b that is compatible with the predicted molecular formula.
An alternative technique was used to demonstrate the chemical structure of 10 (Scheme 2). As a result, the reaction of compound 13 with 9a in refluxing EtOH containing Et3N produced a product that is completely identical to compound 11a.
Compounds 14a,b also interacted with derivative 3 to produce the corresponding thiadiazoles 16a,b (Scheme 3) in a similar manner. When 16a was used as an example of the constructed series for IR, 1H-NMR and 13C-NMR spectra, it could be seen that the hydrazone-NH function had disappeared while the ester CO2CH2CH3 existed excluding the other structure 18a. A peak corresponding to each of the reaction products could be seen in each case's mass spectrum.
Additionally, a different technique was used to illustrate chemically the structure of 16 (Scheme 3). As a result, the reaction of compound 13 with compound 14a in refluxing EtOH/TEA produced a product that is completely identical to compound 16a in all respects.
Additionally, compound 19 and compound 3 were reacted to produce compound 21 (Scheme 4). Compound 21's 1H-NMR spectrum showed a signal at 11.70 ppm corresponding to the carboxamide proton and an aromatic multiplet in the 7.12-7.74 ppm region. Compound 21's mass spectra showed a m/z = 568, which agrees with the suggested structure.
Also, compound 21 was proved chemically using a different technique from the reaction of compound 13 with 19 resulted in a product, which is identical to compound 21 in all aspects (Scheme 4).
2.2. Cytotoxicity evaluation
Numerous studies showed that 1,3,4-thiadiazoles and 1,3-thiazoles have anticancer activity with excellent IC50 and IG50 values () [Citation29–35].
The liver carcinoma (HepG2-1) cell line was used to test the antitumour effect of the newly prepared derivatives. As a reference standard, doxorubicin showed an IC50 value of 0.72 ± 0.52 μM against HepG2-1. The concentration (μM) of test compounds necessary to kill 50% of the cell population (IC50) was established by generating data to create a dose–response curve. The mean IC50 population from three different trials served as the basis for the cytotoxic effects.
According to the results, as shown in , the most of the investigated compounds revealed a highly variable activity when compared to the reference drug. The descending order of activity of the newly synthesized compounds was as follow: 16b > 21 > 16a > 3 > 11b > 11a > 6.
Table 1. Anticancer activities of examined derivatives towards liver carcinoma cell lines.
The following conclusions are drawn from an examination of the SAR ():
The 1,3,4-thiadiazoles 16b and 21 (IC50 = 0.69 ± 0.41 and 1.82 ± 0.94 μM, respectively) have encouraging anticancer activity against HepG2-1 while 1,3,4-thiadiazoles 16a, 11b, and 11a have moderate activity (IC50 = 4.03 ± 13.18 µM). 1,3,4-Thiadiazole 6, on the other hand, shows low anticancer activity (IC50 = 49.39 ± 2. 51 µM).
The CO2Et group at position 2 in the 1,3,4-thiadiazole enhances anticancer activity than the CONHPh group than the COCH3 group, and finally the Ph group .
2.3. Molecular docking study
N-Ribosyldihydronicotinamide: quinone oxidoreductase2 (NQO2/QR2) is a cytosolic enzyme, that is highly concentrated within the liver and can be found in various tissues throughout mammals [Citation36]. It is a critical quinone's metabolism detoxification enzyme. Compared to other types of cancer, hepatocellular tumour tissue has a higher NQO2 activity[Citation37]. Thus, in the present work, the NQO2 receptor PDB (4ZVM) was chosen from the protein database to explore possible binding interactions of the most active compounds using molecular modelling analysis [Citation38,Citation39]. Molecular docking simulations were done against the NQO2 receptor to achieve a multitarget approach and identify a promising candidate. The simulation was done using the GLIDE of Schrödinger [Citation40]. The affinity of the novel synthesized compounds was investigated via SP Glide docking mode against the receptor (4ZVM). In terms of accuracy, we have re-docked the co-crystallized inhibitor doxorubicin into the receptor's active site using the generated grid for validation. The re-docked doxorubicin showed similar binding modes under the criterion of RMSD 1.6 A° as presented in . The novel compounds were similarly docked in the quinone oxidoreductase2 4ZVM. The resulted SP docking score of the studied compounds was between −7.01 and −4.31 kcal/mol (). Compound 16b, the most potent inhibitor against HepG2liver cell lines, showed the lowest docking score (−7.01 kcal/mol) with glide emodel −88.59. The 16b- 4ZVM complex demonstrated four H-bonds with amino acids TRP 105, ASN161 and THR147 as presented in . Previous research has indicated that the inhibitor resveratrol fitted deeply into the NQO2 catalytic site and potently inhibits NQO2 activity via forming a direct hydrogen bond with Asn116 [Citation34] (1). This may justify the efficacy of compound 16b which displayed two hydrogen bonds with ASN161 (2.28 and 2.68 A°). The interaction network of 16a-4ZVM and 11b-4ZVM showed one hydrogen bond with ASN161 and GLU193, respectively. While the interaction mode of 3 presented two H-bonds against ASN161 (2.54 A°) and TRP105 (1.83A°). Compound 21 with a docking score of −6.42 kcal/mol, is located in the cleft of the active pocket but it did not establish any hydrogen interaction with the receptor's amino acids. It was bonded to the receptor via pi-pi stacking against TRP105 residue (). The docked compounds 6 and 11a unveiled a similar pattern (two -
stacking against TRP105) with a higher docking score of −4.31 and −5.10 kcal/mol, respectively.
Figure 3. An Overlay of docking conformation of doxorubicin-4XVM complex (blue stick) and its X-ray structure (pink stick) (PDB: 4ZVM); 2D interaction representation of doxorubicin-4XVM.
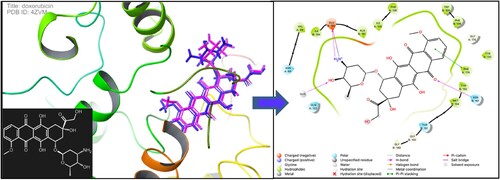
Figure 4. 2D interaction representation of quinone oxidoreductase2 -ligand interactions of the thiadiazole-thiazole derivatives in complexes with PDB (4ZVM).
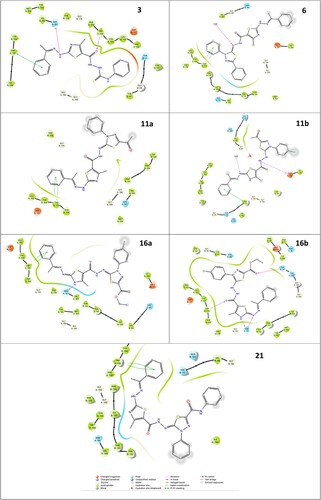
Table 2. The analysis of glide docking for selected thiadiazole-thiazole derivatives on quinone oxidoreductase2 (PDB: 4ZVM).
3. Experimental
See supporting information.
3.1. Synthesis of compound 3
A mixture of carbohydrazide derivative 2 (2.89 g, 10 mmol) and phenyl isothiocyanate (1.35 g, 10 mmol) in ethanol (50 mL) was refluxed for four hours. The formed solid after cooling was recrystallized from EtOH to get the yellow product (3 in 71% yield); mp = 177-179°C; 1H-NMR (500 MHz, DMSO-d6) δ 2.33 (s, 3H, CH3), 2.43 (s, 3H, CH3), 7.06-7.53 (m, 11H, Ar-H and NH), 9.92 (s, 1H, NH), 10.18 (s, 1H, NH), 11.95 (s, 1H, NH) ppm; IR (KBr): v 3412-3237, 3203 (4NH), 3057, 3018, 2927 (C–H), 1663 (C = O), 1603 (C = N) cm−1; MS m/z (%): 424 (M+, 71). Anal Calcd for C20H20N6OS2 (424.54): C, 56.58; H, 4.75; N, 19.80. Found: C, 56.37; H, 4.59; N, 19.64%.
3.2. Synthesis of 1,3,4-thiadiazole derivatives 6, 11a,b, 16a,b and 21
In ethanol (20 mL) containing Et3N (0.1 g, 1 mmol), compound 3 (0.424 g, 1 mmol) and the appropriate hydrazonoyl halides 4, 9a,b, 14a,b, and 19 (1 mmol) were heated under reflux for 4–8 h (as monitored by TLC). The formed solid after cooling was crystallized from DMF or DMF\ EtOH to produce products 6, 11a,b, 16a,b, or 21, respectively. The resulting products’ physical characteristics and spectrum information are shown below.
1,3,4-Thiadiazole derivative 6. Yellow solid (81%); m.p. 241–243 °C; 1H-NMR (DMSO-d6): δ 2.31 (s, 3H, CH3), 2.46 (s, 3H, CH3), 7.08-7.69 (m, 15H, Ar-H), 10.23, 11.79 (2s, br, 2H, 2NH) ppm; IR (KBr): v 3429, 3250 (2NH), 3039, 2941 (CH), 1664 (C = O), 1603 (C = N) cm−1; MS m/z (%): 525 (M+, 100). Anal. Calcd. for C27H23N7OS2 (525.65): C, 61.69; H, 4.41; N, 18.65. Found C, 61.75; H, 4.31; N, 18.49%.
1,3,4-Thiadiazole derivative 11a. Yellow solid (73%); m.p. 191–193 °C; 1H-NMR (DMSO-d6): δ 2.30 (s, 3H, CH3), 2.42 (s, 3H, CH3), 2.46 (s, 3H, CH3), 7.31-7.54 (m, 10H, Ar-H), 10.26, 11.94 (2s, br, 2H, 2NH) ppm; 13C-NMR (DMSO-d6) δ 14.49, 17.91, 22.19 (CH3), 116.19, 118.50, 119.00, 122.37, 124.10, 126.38, 129.17, 129.63, 130.38, 130.57, 137.10, 137.25, 142.69, 149.38 (Ar-H and C = N), 161.49, 194.71 (C = O) ppm; IR (KBr): v 3425, 3248 (2NH), 3051, 2930 (CH), 1715, 1659 (2C = O), 1599 (C = N) cm−1; MS m/z (%): 491 (M+, 88). Anal. Calcd. for C23H21N7O2S2 (491.59): C, 56.20; H, 4.31; N, 19.95. Found C, 56.03; H, 4.18; N, 19.70%.
1,3,4-Thiadiazole derivative 11b. Yellow solid (78%); m.p. 216–218 °C; 1H-NMR (DMSO-d6): δ 2.20 (s, 3H, CH3), 2.35 (s, 3H, CH3), 2.41 (s, 3H, CH3), 7.06-7.54 (m, 9H, Ar-H), 10.19, 11.73 (2s, br, 2H, 2NH, D2O-exchangeable) ppm; IR (KBr): v 3444, 3237 (2NH), 3057, 2929 (CH), 1712, 1662 (2C = O), 1597 (C = N) cm−1; MS m/z (%): 528 (M++2, 23), 526 (M+, 73). Anal. Calcd. for C23H20ClN7O2S2 (526.03): C, 52.52; H, 3.83; N, 18.64. Found C, 52.72; H, 3.90; N, 18.51%.
1,3,4-Thiadiazole derivative 16a. Yellow solid (77%); m.p. 169–171 °C; 1H-NMR (DMSO-d6): δ 1.26 (t, 3H, CH3), 2.30 (s, 3H, CH3), 2.32 (s, 3H, CH3), 2.47 (s, 3H, CH3), 4.31 (q, 2H, CH2), 7.05-7.78 (m, 9H, Ar-H), 10.15, 11.88 (2s, br, 2H, 2NH) ppm; 13C-NMR (DMSO-d6) δ 12.38, 14.46, 17.08, 21.05 (CH3), 60.67 (CH2), 116.44, 120.99, 121.50, 129.59, 129.85, 136.49, 136.84, 137.19, 140.74, 143.86, 146.55, 147.49, 147.55, 154.44 (Ar-C and C = N), 161.71, 168.60 (C = O) ppm; IR (KBr): v 3431, 3238 (2NH), 3037, 2918 (CH), 1683, 1651 (2C = O), 1593 (C = N) cm−1; MS m/z (%): 535 (M+, 97). Anal. Calcd. for C25H25N7O3S2 (535.64): C, 56.06; H, 4.70; N, 18.30. Found C, 56.01; H, 4.53; N, 18.19%.
1,3,4-Thiadiazole derivative 16b. Yellow solid (75%); m.p. 163–165 °C; 1H-NMR (DMSO-d6): δ 1.27 (t, 3H, CH3), 2.30 (s, 3H, CH3), 2.46 (s, 3H, CH3), 4.33 (q, 2H, CH2), 7.05-7.95 (m, 9H, Ar-H), 10.21, 11.92 (2s, br, 2H, 2NH) ppm; IR (KBr): v 3427, 3251 (2NH), 3049, 2933 (CH), 1679, 1653 (2C = O), 1604 (C = N) cm−1; MS m/z (%): 558 (M++2, 14), 556 (M+, 47). Anal. Calcd. for C24H22ClN7O3S2 (556.06): C, 51.84; H, 3.99; N, 17.63. Found C, 51.62; H, 3.90; N, 17.47%.
1,3,4-Thiadiazole derivative 21. Yellow solid (82%); m.p. 203–205 °C; 1H-NMR (DMSO-d6): δ 2.32 (s, 3H, CH3), 2.46 (s, 3H, CH3), 7.12-7.74 (m, 15H, Ar-H), 10.19, 10.60, 11.70 (3s, br, 3H, 3NH) ppm; IR (KBr): v 3425, 3328, 3248 (3NH), 3044, 2928 (CH), 1679, 1658 (2C = O), 1599 (C = N) cm−1; MS m/z (%): 568 (M+, 83). Anal. Calcd. for C28H24N8O2S2 (568.67): C, 59.14; H, 4.25; N, 19.72. Found C, 59.00; H, 4.14; N, 19.68%.
3.3. Alternate synthesis of 1,3,4-thiadiazole derivative 6
Equimolar concentrations of 1,3,4-thiadiazole 7 (0.268 g, 1 mmol) and ester 1 (0.303 g, 1 mmol) were mixed with 20 mL of EtOH and refluxed until no more H2S was emitted, and all of the starting materials had been consumed (12 h, monitored by TLC). The separated product was identified as compound 6.
3.4. Synthesis of compound 13
Addition of carbohydrazide 2 (2.89 g, 10 mmol) to a stirred KOH solution (0.56 g, 10 mmol) in DMF. After cooling, CS2 (10 mmol) was progressively added, and the reaction mixture was heated under reflux for 2 h before adding CH3I (1.4 g, 10 mmol) dropwise while cooling with an ice bath. The precipitated product which formed after addition solution into 10 mL of H2O, was suction-isolated and crystallized from EtOH to yield compound 13 as a white solid in 68% yield; m.p. 149-151°C; 1H-NMR (DMSO-d6): δ 2.31 (s, 3H, CH3), 2.46 (s, 3H, CH3), 2.79 (s, 3H, SCH3), 7.17-7.38 (m, 5H, Ar-H), 9.06, 11.13, 11.84 (3s, br, 3H, 3NH) ppm; IR (KBr): v 3436-3272, 3229 (3NH), 3053, 2937 (CH), 1666 (C = O), 1601 (C = N) cm−1; MS m/z (%): 379 (M+, 36). Anal. calcd for C15H17N5OS3 (379.52): C, 47.47; H, 4.52; N, 18.45. Found: C, 47.38; H, 4.51; N, 18.38%.
3.5. Alternate synthesis of 1,3,4-thiadiazole derivatives 11a, 16a and 21
Triethylamine (0.1 mL, 1 mmol) was added to an ethanolic solution of compound 13 (0.379 g, 1 mmol) and hydrazonoyl chloride 9a, 14a, or 19 (1 mmol). The resulting solid after removal of excess solvent and addition of ice/HCl solution, was recrystallized from DMF to provide the corresponding thiadiazoles 11a, 16a, or 21, which were similar in all aspects (IR spectra, mixed m.p. and m.p.) to those produced by the reaction of 9a, 14a, or 19 with compound 3.
3.6. Cytotoxic assay
The MTT assay was used to evaluate the cytotoxicity of the compounds under study. A complete cytotoxicity assay is given in the supplementary file [Citation41,Citation42].
3.7. Molecular docking analysis
3.7.1. Ligands preparation
First, the ligands were prepared using the LigPrep module of the Schrödinger package [Citation43]. The novel synthesized compounds’ final 3D structures were prepared for computational analysis under physiological pH circumstances. An OPLS3e force field algorithm was used to reduce the ligand geometry.
3.7.2. Protein preparation, grid generation and glide docking
The 3D structure of quinone oxidoreductase2 (PDB code: 4ZVM) in 1.97 Å resolution was downloaded from the RCSB Protein Data Bank [Citation39]. The protein was prepared using a protein preparation wizard in the Schrödinger suite supported by the OPLS3e force field [Citation44]. The protein was utilized to generate receptor grids for the docking procedure. The receptor grid was created by using the centroid of the co-crystallized inhibitor doxorubicin as the grid centre on the active site of the 4ZVM protein. The grid coordinates were 48.43 (X), 4.81 (Y), and 13.89 (Z), respectively. Rigid receptor docking protocol was run in Glide's standard precision (SP) mode based on the OPLS-3e force field [Citation40]. During the process of docking, the protein was fixed, while ligands were flexible.
4. Conclusion
Novel 1,3,4-thiadiazole-based thiazoles have been synthesized, these newly prepared compounds were determined based on both spectroscopic and elemental data, as well as other methods when feasible. All of the synthesized compounds were tested for their anti-cancer effects using HepG2-1 cell line. It is found that the thiazoles 16b, and 21 having IC50 values = 0.69 ± 0.41 and 1.82 ± 0.94 µM, respectively, were the compounds with the highest level of activity of the produced series. Moreover, SP Glide docking of these compounds revealed promising high affinities against NQO2 receptor (PDB: 4ZVM). On the basis of the experimental findings gathered from the anticancer activity, the structure–activity correlations were dissected and addressed.
Supplemental Material
Download MS Word (17.2 KB)Acknowledgements
Ghadah Aljohani extended her appreciation to, Taibah University, represented by the Deanship of Scientific Research, for providing the license of Schrödinger suite. Also, the authors acknowledge the Deanship of Scientific Research at Princess Nourah bint Abdulrahman University for financial support through the Research Funding Program (PNURSP2022R24).
Disclosure statement
No potential conflict of interest was reported by the author(s).
Additional information
Funding
References
- Gomes JPM, Cardoso CRP, Varanda EA, et al. Mutagenic and (anti)estrogenicactivities of tingenone and pristimerin. Rev Bras Farmacogn. 2011;21:963–971.
- Ioannou GN, Splan MF, Weiss NS, et al. Incidence and predictors of hepatocellular carcinoma in patients with cirrhosis. Clin Gastroent Hepatol. 2007;5:938–945.
- Ferlay J, Soerjomataram I, Dikshit R, et al. Cancer incidence and mortality worldwide: sources, methods and major patterns in GLOBOCAN 2012. Int J Cancer. 2015;136:E359–E386.
- Jemal A, Ward EM, Johnson CJ, et al. Annual report to the nation on the status of cancer, 1975-2014, featuring survival. J Nat Cancer Inst. 2017;109. doi:10.1093/jnci/djx030
- Sujatha K, Vedula RR. Novel one-pot expeditious synthesis of 2,4-disubstituted thiazoles through a three-component reaction under solvent free conditions. Synth Commun. 2018;48:302–308.
- Gomha SM, Abdelhady HA, Hassain DZH, et al. Thiazole based thiosemicarbazones: synthesis, cytotoxicity evaluation and molecular docking study. Drug Design Development and Therapy. 2021;15:659–677.
- Alshabanah LA, Al-Mutabagani LA, Gomha SM, et al. Three-component synthesis of some new coumarin derivatives as anti-cancer agents. Front Chem. 2022;9:762248.
- Mahmoud HK, Sayed AR, Abdel-Aziz MM, et al. Synthesis of new thiazole clubbed imidazo[2,1-b]thiazole hybrid as antimycobacterial agents. Med Chem. 2022;18:1100–1108.
- Nayak S, Gaonkar S L. A review on recent synthetic strategies and pharmacological importance of 1,3-thiazole derivatives. Mini Rev Med Chem. 2019;19:215–238.
- Kumar S, Aggarwal R. Thiazole: A privileged motif in marine natural products. Mini Rev Org Chem. 2019;16:26–34.
- Sayed AR, Elsawy H, Shaaban S, et al. Design, synthesis, and biological evaluations of novel az-othiazoles based on thioamide. Curr Issues Mol Biol. 2022;44:2956–2966.
- Morigi R, Locatelli A, Leoni A, et al. Recent patents on thiazole derivatives endowed with antitumor activity. Recent Pat Anticancer Drug Discov. 2015;10:280–297.
- Fuqiang LIU, Palmer DC, Sorgi KL. Diethoxyphosphinyl acetic acid hydrazide: a uniquely versatile reagent for the preparation of fused [5,5]-, [5,6]-, and [5,7]-3-[(E)-2-(arylvinyl)]-1,2,4-triazoles. Tetrahedron Lett 2004;45:1877–1880.
- Danilenko GI, Rubalko SL, Maksimov YN, et al. Adamantane-1- and norbornane-2-carboxylic acid hydrazides as HIV inhibitors. Pharmaceut Chem J. 2000;34:23–24.
- Constable DJC, Curzons AD, Cunningham VL. Metrics to ‘green’ chemistry-which Are the best? Green Chem. 2002;4:521–527.
- Saha A, Kumar R, Kumar R, et al. Development and assessment of green synthesis of hydrazides. Indian J Chem. 2010;49B:526–531.
- Kushwaha N, Kushwaha SKS, Rai AK. Biological activities of thiadiazole derivatives: A review. Inter J Chem Res. 2012;4:517–531.
- Singh AK, Mishra G, Jyoti K. Review on biological activities of 1,3,4-thiadiazole derivatives. J App Pharm Sci. 2011;1:44–49.
- Siddiqui N, Ahuja P, Ahsan W, et al. Thiadiazole: progress report on biological activities. J Chem Pharm Res. 2009;1:19–30.
- Gomha SM, Riyadh SM, Huwaimel B, et al. Synthesis, molecular docking study and cytotoxic activity on mcf cells of some new thiazole clubbed thiophene scaffolds. Molecules. 2022;27:4639.
- Gomha SM, Abdel-Aziz HA. Enaminones as building blocks in heterocyclic preparations: synthesis of novel pyrazoles, pyrazolo[3,4-d]pyridazines, pyrazolo[1,5-a]pyrimidines, pyrido[2,3-d]pyrimidines linked to imidazo[2,1-b]thiazole system. Heterocycles. 2012;85:2291–2303.
- Abbas IM, Riyadh SM, Abdallah MA, et al. A novel route to tetracyclic fused tetrazines and thiadiazines. J Heterocycl Chem. 2006;43:935–942.
- Said MA, Riyadh SM, Al-Kaff NS, et al. Synthesis and greener pastures biological study of bis-thiadiazoles as potential COVID-19 drug candidates. Arabian J Chem. 2022;15:104101.
- Eldebss TMA, Gomha SM, Abdulla MM, et al. Novel pyrrole derivatives as selective CHK1 inhibitors: design, regioselective synthesis and molecular modeling. Med Chem Com. 2015;6:977–987.
- Gomha SM, Edrees MM, Muhammad ZA, et al. 5-(Thiophen-2-yl)-1,3,4-thiadiazole derivatives: synthesis, molecular docking and in-vitro cytotoxicity evaluation as potential anticancer agents. Drug Design Development and Therapy. 2018;12:1511–1523.
- Makam P, Kankanala R, Amresh P, et al. 2-(2-Hydrazinyl)thiazole derivatives: design, synthesis and in vitro antimycobacterial studies. Eur J Med Chem. 2013;69:564–576.
- Al-Humaidi JY, Badrey MG, Aly AA, et al. Evaluation of the binding relationship of the RdRp enzyme to novel thiazole/ acid hydrazone hybrids obtainable through green synthetic procedure. Polymer. 2022;14(15):3160.
- Abdelhamid AO, Zohdi HF, Rateb NM. Reactions with hydrazonoyl halides XXI: reinvestigation of the reactions of hydrazonoyl bromides with 1,1-dicyanothio-acetanilide. J Chem Res. (S). 1998;3:184–185.
- Qiu XL, Li G, Wu G, et al. Synthesis and biological evaluation of a series of novel inhibitor of Nek2/Hec1 analogues. J Med Chem. 2009;52:1757–1767.
- Tsoua H, MacEwan G, Birnberg G, et al. Discovery and optimization of 2-(4-substituted-pyrrolo[2,3-b]pyridin-3-yl) methylene-4-hydroxybenzofuran- 3(2H)-ones as potent and selective ATP-competitive inhibitors of the mammalian target of rapamycin (mTOR). Bioorg Med Chem Lett. 2010;20:2321–2325.
- Mavrova AT, Wesselinova D, Tsenov YA, et al. Synthesis, cytotoxicity and effects of some1,2,4-triazole and 1,3,4-thiadiazole derivatives on immunocompetent cells. Eur J Med Chem. 2009;44:63–69.
- Oleson JJ, Slobada A, Troy WP, et al. The carcinostatic activity of some 2-amino-1,3,4-thiadiazoles. J Am Chem Soc. 1955;77:6713–6714.
- Matysiak J, Opolski A. Synthesis and antiproliferative activity of N-substituted 2-amino-5-(2,4-dihydroxyphenyl)-1,3,4-thiadiazoles. Bioorg Med Chem. 2006;14:4483–4489.
- Kaminskyy D, Kryshchyshyn A, Nektegayev I, et al. Isothiocoumarin-3-carboxylic acid derivatives: synthesis, anticancer and antitrypanosomal activity evaluation. Eur J Med Chem. 2014;75:57–66.
- Popsavin M, Spaić S, Svirčev M, et al. Synthesis and in vitro antitumour screening of 2-(β-d-xylofuranosyl)thiazole-4-carboxamide and two novel tiazofurin analogues with substituted tetrahydrofurodioxol moiety as a sugar mimic. Bioorg Med Chem Lett. 2012;22:6700–6704.
- Janda E, Nepveu F, Calamini B, et al. Molecular pharmacology of NRH:quinone oxidoreductase 2: A- detoxifying enzyme acting as an undercover toxifying enzyme. Molecular Pharmacol. 2020;98:620–633.
- Middleton MR, Knox R, Cattell E, et al. Quinone oxidoreductase-2-mediated prodrug cancer therapy. Sci Translational Med. 2010;2:40–50.
- Leung KKK, Shilton BH. Binding of DNA-intercalating agents to oxidized and reduced quinone reductase 2. Biochem. 2015;54:7438–7448.
- RCSB PDB - 4ZVM: Oxidized quinone reductase 2 in complex with doxorubicin [Internet]. [cited 2022 Jun 21]. Available from: https://www.rcsb.org/structure/4ZVM
- Schrödinger Release 2021-4: Glide, Schrödinger, LLC, New York, NY. New York, NY: Schrödinger, LLC.; 2021.
- Mosmann T. Rapid colorimetric assay for cellular growth and survival: application to proliferation and cytotoxicity assays. J Immunol Methods. 1983;65:55–63.
- Gomha SM, Riyadh SM, Mahmoud EA, et al. Synthesis and anticancer activities of thiazoles, 1,3-thiazines, and thiazolidine using chitosan-grafted-poly(vinylpyridine) as basic catalyst. Heterocycles. 2015;91:1227–1243.
- Schrödinger Release 2021-4: LigPrep, Schrödinger, LLC, New York, NY. New York, NY; 2021.
- Schrödinger Release 2021-4: Protein Preparation Wizard; Epik, Schrödinger, LLC, New York, NY, 2021; Impact, Schrödinger, LLC, New York, NY; Prime, Schrödinger, LLC, New York, NY. 2021.