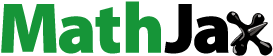
Abstract
Ni-Al-Cd oxide nanostructures were fabricated by facile co-precipitation method and was embedded on CNTs by the ultrasonication method. The properties and structural features of prepared materials were analyzed by various techniques such as X-Ray diffraction, scanning electron microscopy, UV-visible spectroscopy and Fourier transform infrared spectroscopy. The synthesized nanomaterials were employed for degradation of methylene blue under sunlight and for antibacterial study. The photocatalytic results indicate that the ternary oxide of Ni-Al-Cd has a greater potential for degradation of methylene blue and other harmful compounds owing to possible heterojunction development. The photocatalytic degradation of ternary Ni-Al-Cd oxides/CNTs was 67.16% within 2 h. Moreover, photocatalytic activity was increased by carbon nanotubes. The results revealed that ternary oxides and their nanocomposite with CNTs have an excellent ability for photocatalytic degradation and showed better antibacterial activity than ternary oxides.
GRAPHICAL ABSTRACT
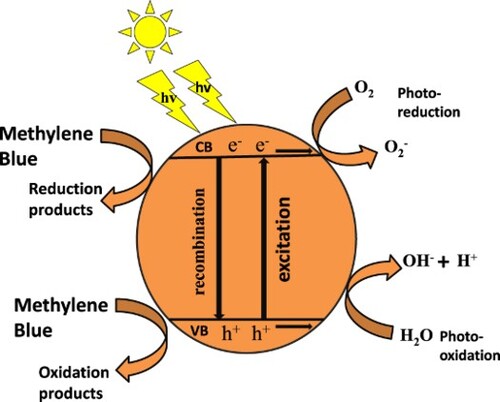
1. Introduction
The effluents of industries have hazardous, poisonousand carcinogenic materials. These waste materials are the basic reason for different environmental crises. Coloured compounds, such as organic dyes, are a dramatic source of non-aesthetic pollution, acidificationand anthropogenic for life on earth [Citation1,Citation2]. Pure water is a necessity for living organisms for their healthy survival on earth. Human activities deteriorate the cleanliness of the water and continuously damage the ecosystem [Citation3,Citation4]. The colours are getting a huge place on the commercial level and rapidly destroying the food chain as they are mostly irremovable organic dyes that could resist the natural process of degradation and require some proper methodologies to reduce into harmless materials. There are several techniques to reduce the harmful influence of organic compounds on the ecosystem. Photocatalysis, among them, is an excellent replacement to decolorize and detoxify industrial wastewater [Citation5]. Photocatalytic degradation of organic dyes is more appropriate because the pollutant is degraded rather than modified [Citation6–8]. This technique assists to convert a large variety of environmental toxins, organic dyes, coloured materials, pesticides and herbicides into eco-friendly products [Citation9–11]. Solar energy is the natural ingredient of photocatalysis. The spectrum of light is composed of various radiations of different energies which are used in the process of photocatalysis. However, visible radiation makes it a more convenient and economical process. The shifting of electrons from the valance band to the conduction band occurs and photogenerated electron – holes pairs are formed by the absorption of accelerated light photons.
A series of redox reactions started after the absorption of an energetic photon. Oxygen molecules and electrons reacted to produce superoxide radical anion and water reacts with the hole to produce hydroxyl radical. The formation of free radicals ensures the conversion of organic dyes into secondary products [Citation12]. Although several materials have been fabricated for the photocatalytic treatment of dyes in the laboratory and on a commercial scale. However, metal oxides and Tri-metal oxides have been widely used materials among them. The development of advanced tri-metal oxides as photocatalysts is the main field of research due to their unique working principle and excellent work efficiency [Citation13]. The tri-metal oxides can arrest photons to initiate a redox reaction which breaks the complex structured dyes found in wastewater. This happens due to the narrow bandgap energy and high chemical reactivity of as-synthesized material. Moreover, the nanocomposites of tri-metal oxides with carbonaceous nanomaterials, such as graphene-reduced graphene oxide and CNTs, induced interesting properties in them [Citation14,Citation15]. The resultant product contains the properties of metal oxides and carbonaceous materials such as reduced band gap, enhanced surface area, controlled recombination rate and enhanced photon-capturing ability. Tri-metal oxide photocatalyst performs a vital role at the commercial level due to its acid–base and redox properties. A material with modified structural and electronic properties is obtained by suitable mixing of individual metal oxides. The morphology of tri-metal oxides has been attractive due to their magnified photocatalytic performance. Moreover, the addition of carbon nanotubes boosts the performance of photocatalysts [Citation16].
Transition metal oxides reduce the electron–hole pair recombination because they are active acceptors of electrons and promote the interfacial charge transfer process. In recent work, we prepared an economically approachable material for the elimination of organic dye like methylene blue from water using Ni-Al-Cd oxide and Ni-Al-Cd oxide/CNTs as promising photocatalysts [Citation13,Citation17,Citation18]. Yousaf et al. synthesized the nanocomposite of NiO-ZnO/rGO and successfully degraded the organic dyes present in wastewater [Citation19]. Irshad et al. prepared the Ag-doped NiAl2O4 for the photocatalytic approach. The overall removal of BA, CV and MB by Ag-doped NiAl2O4 was 76.91%, 98.54% and 99.67%, respectively. Rahman et al. studied the photocatalytic activity of a ternary composite of Ce-Fe-Ni oxide for RhB dye. The degradation rate of RhB is 97% within 130 min [Citation20]. Hassena et al. used the Al-oxide/Fe-oxide nanocomposite for photocatalytic degradation under visible light. Rahman et al. went through the dual metal exchange of CdO with excellent performance for dye photodegradation and antibacterial action [Citation21]. Karthik et al. synthesized the MMO of cadmium, nickel and zinc by the microwave-assisted method. The ZnO-CdO-NiO photocatalyst degraded the methylene blue under visible light with a high percentage [Citation22]. For photocatalytic destruction until now, there has been no reported research on Ni-Al-Cd oxide/CNTs.
In this report, ternary metal oxide nanoparticles and their nanocomposite with carbon nanotubes were synthesized and used for the degradation of an organic pollutant under sunlight and for antibacterial study. The synthesized materials were characterized by physio-chemical techniques.
2. Experimental studies
2.1. Materials
Nickel chloride hexahydrate (NiCl2·6H2O), aluminium nitrate Al(NO3)3, Cadmium chloride (CdCl2·H2O),Sodium carbonate (Na2CO3) and carbon nanotubes (CAS # 308068-56-6) were used as starting materials in the synthesis of ternary metal oxides and their nanocomposite with carbon nanotubes. All the used chemicals were bought from basic suppliers like Sigma Aldrich. Without any purification, the chemicals of analytical grade were used.
2.2. Characterization
To study the morphological features and effects of synthesized materials techniques, such as FTIR, XRD, SEM and UV-Visible, were used. An FEI Inspect S50 scanning electron microscope (SEM) was used to analyze the morphology of the fabricated samples. For functional group analysis, FTIR spectra were recorded using a Thermo Scientific Nicolet 380 FT-IR spectrometer. For structural analysis, XRD spectra were recorded on a Philips X’Pert XRD diffractometer. It involves the use of CuKα as a radiation source. For optical analysis, a dual-beam spectrophotometer (carry 60) was used.
2.3. Synthesis of Ni-Al-Cd oxide by the coprecipitation method
The Ni-Al-Cd oxide was fabricated by a facile co-precipitation route. The precursors’ salts of each metal were used for the synthesis of Ni-Al-Cd oxide. Without further purification, the chemicals were used. In double distilled water 0.25 M aqueous solution of each salt was prepared. To get a homogeneous mixture of three different salt solutions stirred all the metal solutions for 1 min at room temperature. After that, precipitating agent solution was prepared. Then 30 cm3 solution of sodium carbonate was added dropwise to the solution of the metal. For 4 h at 45°C, the mixture was stirred until precipitates were formed. Precipitates were neutralized and additional impurities were removed by washing at certain times with deionized water. Then dehydration was implemented at 140°C for 4 h. After that grinding and annealing of the dried sample were done for 4 h at 550°C in the Muller furnace. The annealing converts the carbonates into Ni-Al-Cd oxide and this as-synthesized material was used to explore the photocatalytic ability of dye removal [Citation8].
2.4. Synthesis of a Ni-Al-Cd nanocomposite with carbon nanotubes
Ni-Al-Cd oxide nanocomposite with CNTs was synthesized by a simple sonication approach. Stoichiometric amounts of ternary oxide and carbon nanotubes were introduced in 20 cm3 of deionized water. The homogeneous mixture was obtained by the ultra-sonication process. The CNTs used in the synthesis process were purchased from Nano-Lab, Waltham-USA. The synthesized nanocomposite was dried in the air. The synthesis scheme of ternary oxides of Ni-Al-Cd and their nanocomposite with carbon nanotubes are displayed in Figure .
2.5. Photocatalytic study
The photocatalytic performance of ternary oxide of Ni-Al-Cd and ternary oxide of Ni-Al-Cd/CNTs nanocomposite was probed using methylene blue dye. The experiment was carried out by ternary oxide of Ni-Al-Cd and ternary oxide of Ni-Al-Cd/CNTs nanocomposite to compare the performance because of the addition of CNTs in the ternary metal oxide. Twenty milligrams of the photocatalyst was added to a 50 cm3 solution of 5 ppm organic dye (Methylene blue). When the photocatalyst and methylene blue established equilibrium in adsorption and desorption processes in the dark, the solution was subjected to light exposure. The light source used for the illumination was a 200 W low-pressure bulb. With time, the colour intensity of the solution decreased gradually and after 120 min of light exposure, the mixture of photocatalyst nanocomposite and dye became colourless ensuring the effective degradation ability of the photocatalyst. But the colour of the solution using photocatalyst ternary oxide of Ni-Al-Cd stays within 120 min. To explore the extent of reduction in absorption, a Carry 60 spectrophotometer was used. The photocatalytic efficiency was obtained by the following relation
(1)
(1)
3. Result and discussion
3.1. XRD analysis
The phase examination of ternary oxides of Ni-Al-Cd was carried out by XRD analysis. Figure reveals the XRD pattern of the ternary oxide (Ni-Al-Cd oxide) in which all the peaks are related to synthesizing metal oxides. For CdO, the diffraction peak was observed at reflection (311) well-matched with the cubic CdO as in JCPDS Card No. 65-2908 [Citation23]. For α – Al2O3, the reflection peak (110) was matched with JCPDS Card No. 46-1212 [Citation24]. For NiO, the reflections (111), (220) and (311) were matched with JCPDS Card No. 04-0835. The sample purity was ensured as all the peaks were related to metal oxides and no extra peak was observed. The XRD pattern of CNT-based composite showed an extra peak which is attributed to the carbon nanotubes. The XRD pattern of as-prepared samples is represented in Figure .
3.2. SEM analysis
The surface morphology of as-fabricated materials was analyzed with the help of a scanning electron microscope. Figure shows the SEM micrographs of (a) Ni-Al-Cs and (b) Ni-Al-Cd/CNTs nanocomposite. The results showed that the prepared ternary oxide showed the morphology of agglomerated particles. The CNT can be easily observed in the SEM micrograph of Ni-Al-Cd nanocomposite. The surface area of the ternary oxide was enhanced due to carbon nanotubes which is the main reason for better performance in photocatalytic and antibacterial studies.
3.3. FTIR study
A spectral survey revealed the functional groups of as-synthesized material. The peak at 3411 cm−1 represents the common band of O–H stretching [Citation5]. The peak at 1625 cm−1 is associated with the O–H band of H2O [Citation4,Citation25]. The peak at 1381 and 862 cm−1 is assigned to CdO stretching. The spectral analysis of metal oxides is presented in Figure .
3.4. Ultraviolet-visible spectroscopy
UV-visible spectroscopy was performed to confirm the absorption regions of as-fabricated materials. The graph is plotted between wavelength on the x-axis, and absorbance on the y-axis. The 200–800 nm wavelength range was used to take UV-Visible measurements. Figure shows the absorption spectra ofNi-Al-Cd and Ni-Al-Cd/CNTs.
4. Photocatalytic study
The photocatalytic performance of the ternary oxide of Ni-Al-Cd and ternary oxide of Ni-Al-Cd/CNTs nanocomposite was probed using methylene blue dye. The experiment was carried out by ternary oxide of Ni-Al-Cd and ternary oxide of Ni-Al-Cd /CNTs nanocomposite to compare the performance because of the addition of CNTs in the ternary metal oxide. Twenty milligrams of the photocatalyst was added to a 50 cm3 solution of 5 ppm organic dye (Methylene blue). When the photocatalyst and methylene blue established equilibrium in adsorption and desorption processes in the dark, the solution was exposed to light. The light used for the illumination was a 200 W low-pressure bulb. With time, the colour intensity of the solution decreased gradually and after 120 min of light exposure, the mixture of photocatalyst nanocomposite and dye became colourless ensuring the effective degradation ability of the photocatalyst. But the colour of the solution using photocatalyst ternary oxide of Ni-Al-Cd stays within 120 min. To explore the extent of reduction in absorption, a Carry 60 spectrophotometer was used. The percent photocatalytic efficiency was obtained by the following relation
(2)
(2) where At and Ao represent the absorbance at time “t min” and time “0 min”, respectively.
The spectra of photocatalytic degradation are provided in Figure . The ternary oxide of Ni-Al-Cd and nanocomposite of ternary oxide (Ni-Al-Cd/CNTs) give a percentage efficiency of 40.21% and 67.16%, respectively.
CNTs-based composites play a crucial role in photocatalytic study because of their intrinsic properties generated by the addition of CNTs into the composite. Functionalization of CNTs or development of agglomerates of metal oxides with CNTs further enhances the field of application of these nanosystems in photocatalysis. The larger surface area and charge separation are major causes of excellent photocatalytic performance after the addition of CNTs in a composite. The mixture of metal oxides has expanded surface area; in other words, it shows more reaction sites. The degradation rate can be increased by decreasing the electron–hole recombination rate; arresting the particles’ agglomeration; and increasing the adsorption capacity, as these are key factors in photocatalysis.
The nanocomposite has a high degradation rate because of the high concentration of electron-trapping species and induced efficient visible light-absorbing ability in the nanocomposite. The redox reaction started, and various radicals are formed. These radicals photocatalyzed the methylene blue into CO2 and H2O. The photodegradation of methylene blue is not a single-step reaction but occurs by multiple steps. Methylene blue comprises various functional groups along with ring structure. All the functional groups were converted into mild products by sequential and continuous attacks of hydroxyl free radicals. Carbon dioxide is a product of the Photo-Kolbe decarboxylation of acids produced during reactions. The chain of intermediate reaction breaks the methylene blue into simple species like water and carbon dioxide [Citation21,Citation26–29]. Figure exhibits the absorption profile and percentage. The kinetic plots of the degradation of methylene blue are shown in Figure . The degradation of methylene blue is exhibited in Figure .
5. Scavenging experiment
For the investigation of the basic mechanism involved in photodegradation, a scavenging experiment was carried out. To investigate the character of electrons, hydroxyl radicals and holes in photocatalytic experiments different scavengers were employed. 2-propanol, AgNO3 and EDTA were employed as trapping agents for hydroxyl radicals, electrons and holes, respectively. Figure exhibits the kinetics plots for degradation photocatalytically using a scavenging procedure. In the presence of 2-propanol, AgNO3 and EDTA, the outcomes of the scavenging experiment showed a significant decrease in the photodegradation rate. In comparison, electrons have the least role, while hydroxyl plays the maximum character in photodegradation.
6. Antibacterial activity
The antimicrobial activity of as-synthesized materials Ni-Al-Cd and CNTs-based nanocomposite of Ni-Al-Cd was analyzed by the disc diffusion method. The activity was tested against Staphylococcus aureus and Klebsiella pneumonia using ciprofloxacin as a standard. The fabricated materials were sonicated in distilled water first, and then they were dispersed on plates containing bacteria. After incubation for one day, the inhibition zones were seen on these plates. These zones of inhibition confirmed that the prepared samples are antibacterial agents. Figure shows the antibacterial activity of samples. The antimicrobial activity of nanocomposite for both S. aureus and K. pneumonia is higher than that of Ni-Al-Cd. Table shows that with the increase in the concentration of the sample the activity was increased. The large size of the inhibition zone means high antimicrobial activity. Hence Ni-Al-Cd/CNTs nanocomposite is an efficient antibiotic against S. aureus and K. pneumonia.
Table 1. The results of antibacterial activity by preparedsamples.
7. Conclusion
For the photocatalytic degradation of organic dyes like methylene blue (MB) and for antibacterial study: ternary oxides of Ni-Al-Cd and their composite with carbon nanotubes were fabricated by co-precipitation and ultrasonication method, respectively. The ternary oxides of Ni-Al-Cd with carbonaceous nanomaterials such as CNTs shorten the bandgap, magnify the surface area and control the recombination rate. The degradation of methylene blue by ternary oxides Ni-Al-Cd photocatalyst was 67.16%. This excellent increment in inefficiency was because of the various modifications offered by CNTs. The results revealed that ternary oxides of Ni-Al-Cd and their nano-composite with carbon nanotubes have an excellent ability for photocatalytic dye degradation and for antibacterial action against S. aureus and K. pneumonia.
Acknowledgements
This research work was funded by institutional fund projects under grant number IFP-A-2022-2-1-08. Therefore, theauthors gratefully acknowledge technical and financial support from the Ministry of Education (Saudi Arabia) and the University of Hafr Al Batin, Saudi Arabia.
Disclosure statement
No potential conflict of interest was reported by the author(s).
Additional information
Funding
References
- Irshad A, Farooq F, Farooq Warsi M, et al. Ag-doped FeCo2O4 nanoparticles and their composite with flat 2D reduced graphene oxide sheets for photocatalytic degradation of colored and colorless compounds. FlatChem. 2022;31:100325.
- Bashir B, Khalid MU, Aadil M, et al. CuxNi1-xO nanostructures and their nanocomposites with reduced graphene oxide: synthesis, characterization, and photocatalytic applications. Ceram Int. 2021;47(3):3603–3613.
- Rahman A, Warsi MF, Shakir I, et al. Fabrication of Ce3+ substituted nickel ferrite-reduced graphene oxide heterojunction with high photocatalytic activity under visible light irradiation. J Hazard Mater. 2020;394:122593.
- Irshad A, Warsi MF, Agboola PO, et al. Sol-gel assisted Ag doped NiAl2O4 nanomaterials and their nanocomposites with g-C3N4 nanosheets for the removal of organic effluents. J Alloys Compd. 2022;902:163805.
- Irshad A, Shahid M, El-Bahy SM, et al. Nickel doped CoAl2O4@CNT nanocomposite: synthesis, characterization, and evaluation of sunlight driven catalytic studies. Phys B. 2022;636:413873.
- Din SHU, Arshed MH, Ullah S, et al. Ag-doped nickel ferrites and their composite with rGO: synthesis, characterization, and solar light induced degradation of coloured and colourless effluents. Ceram Int. 2022;48(11):15629–15639.
- Warsi MF, Bashir B, Zulfiqar S, et al. Mn1-xCuxO2/reduced graphene oxide nanocomposites: synthesis, characterization, and evaluation of visible light mediated catalytic studies. Ceram Int. 2021;47(4):5044–5053.
- Fatima R, Warsi MF, Zulfiqar S, et al. Nanocrystalline transition metal oxides and their composites with reduced graphene oxide and carbon nanotubes for photocatalytic applications. Ceram Int. 2020;46(10):16480–16492.
- Mehrjouei M, Müller S, Möller D. A review on photocatalytic ozonation used for the treatment of water and wastewater. Chem Eng J. 2015;263:209–219.
- Alinsafi A, Evenou F, Abdulkarim E, et al. Treatment of textile industry wastewater by supported photocatalysis. Dyes Pigm. 2007;74(2):439–445.
- Grau P. Textile industry wastewaters treatment. Water Sci Technol. 1991;24(1):97–103.
- Zhang J, Tian B, Wang L, et al. Mechanism of photocatalysis, photocatalysis. CT: Springer; 2018. p. 1–15
- Fatima R, Warsi MF, Sarwar MI, et al. Synthesis and characterization of hetero-metallic oxides-reduced graphene oxide nanocomposites for photocatalytic applications. Ceram Int. 2021;47(6):7642–7652.
- Şenocak A, Tümay SO, Makhseed S, et al. A synergetic and sensitive physostigmine pesticide sensor using copper complex of 3D zinc (II) phthalocyanine-SWCNT hybrid material. Biosens Bioelectron. 2021;174:112819.
- Şenocak A, Tümay SO, Ömeroğlu İ, et al. Crosslinker polycarbazole supported magnetite MOF@ CNT hybrid material for synergetic and selective voltammetric determination of adenine and guanine. J Electroanal Chem. 2022;905:115963.
- Akyüz D, Şenocak A, Köksoy B, et al. Coumarin bearing asymmetrical zinc (II) phthalocyanine functionalized SWCNT hybrid nanomaterial: synthesis, characterization and investigation of bifunctional electrocatalyst behavior for water splitting. J Electroanal Chem. 2021;897:115552.
- Karunakaran C, Magesan P, Gomathisankar P, et al. Photocatalytic degradation of dyes by Al2O3-TiO2 and ZrO2-TiO2 nanocomposites, materials science forum. Trans Tech Publ. 2013;734:325–333.
- Subhan MA, Fahim AMM, Saha PC, et al. Structural study, photoluminescence and photocatalytic properties of La2O3⋅ Fe3O4⋅ ZnO, AgO⋅ NiO⋅ ZnO and La2O3⋅ AgO⋅ ZnO nanocomposites. Nano-Struct Nano-Objects. 2017;10:30–41.
- Yousaf S, Zulfiqar S, Din MI, et al. Solar light irradiated photocatalytic activity of ZnO–NiO/rGO nanocatalyst. J Mater Res Technol. 2021;12:999–1009.
- Rahman A, Zulfiqar S, Musaddiq S, et al. Facile synthesis of Ce1-xFexO2/NiO/rGO ternary hybrid heterostructures with enhanced visible light mediated photocatalytic activity for waterborne pollutants. J Photochem Photobiol A. 2020;397:112583.
- Rahman A, Aadil M, Zulfiqar S, et al. Fabrication of binary metal substituted CdO with superior aptitude for dye degradation and antibacterial activity. Ceram Int. 2021;47(6):8082–8093.
- Karthik K, Dhanuskodi S, Gobinath C, et al. Multifunctional properties of microwave assisted CdO–NiO–ZnO mixed metal oxide nanocomposite: enhanced photocatalytic and antibacterial activities. J Mater Sci: Mater Electron. 2018;29(7):5459–5471.
- Balamurugan S, Balu A, Usharani K, et al. Synthesis of CdO nanopowders by a simple soft chemical method and evaluation of their antimicrobial activities. Pac Sci Rev A Nat Sci Eng. 2016;18(3):228–232.
- JCPDS FN. 46-1212. JCPDS-International Center forDiffraction Data, ICDD; 2001.
- Shaheen N, Yousuf MA, Shakir I, et al. Wet chemical route synthesis of spinel oxide nano-catalysts for photocatalytic applications. Phys B. 2020;580:411820.
- Khan I, Saeed K, Zekker I, et al. Review on methylene blue: Its properties, uses, toxicity and photodegradation. Water (Basel). 2022;14(2):242.
- Bayahia H. Green synthesis of activated carbon doped tungsten trioxide photocatalysts using leaf of basil (Ocimum basilicum) for photocatalytic degradation of methylene blue under sunlight. J Saudi Chem Soc. 2022;26(2);101432.
- Motamedi M, Yerushalmi L, Haghighat F, et al. Recent developments in photocatalysis of industrial effluents: a review and example of phenolic compounds degradation. Chemosphere. 2022;296:133688.
- Rong P, Jiang Y-F, Wang Q, et al. Photocatalytic degradation of methylene blue (MB) with Cu1-ZnO single atom catalysts on graphene-coated flexible substrates. J Mater Chem A. 2022;10:6231–6241.