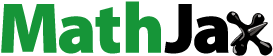
Abstract
We analyzed the dielectric properties of low density polyethylene (LDPE) grad (LA071) materials after different time intervals of contact with apple, pineapple, mango, and cow’s milk. Cole–Cole plots show the distribution of relaxation, depending on the time of contact and the type of food. Furthermore, chemical processes between LDPE and foods lead to significant changes in the dielectric properties of LDPE samples, relying on contact time and food type. In our study, contact with cow’s milk causes the most LDPE degradation, whereas contact with mango causes the least LDPE degradation. It is suggested that dielectric measurements be used as indicators of LDPE degradation and some other defects.
KEYWORDS:
1. Introduction
Polymeric materials play an important role in a wide range of applications and have long been employed to replace an extensive range of materials [Citation1–5]. This is most likely due to polymers’ advantages compared to traditional materials [Citation5–10]. Since then, polymer-based products have become ubiquitous; including clothing made from synthetic fibers, fiberglass, polyethylene cups, plastic bags, nylon bearings, epoxy glue, heart valves, polymer-based paints, and coated cookware [Citation11]. As a result, it is not strange that approximately all material scientists, as well as more than half of all chemists and chemical engineers, physicists, clothing technologists, mechanical engineers, pharmacists, mechanical engineers, and other scientific groups, are associated with polymer research and development projects [Citation12]. Low density polyethylene (LDPE) is the best material that is widely used in a variety of industrial applications, including packaging [Citation13]. It is known as an effective electrical insulator, with amazing properties such as low permittivity and cross-linked polyethylene, demonstrating greater stability and high solvent resistance [Citation14]. The dielectric properties of LDPE are supposed to change with sustained AC stress in high humidity due to increased conductivity caused by ionic products as well as injected and then localized space charges. Furthermore, the rate of water absorption in LDPE is affected by the nature of the surrounding medium [Citation15]. The movement of dissolved ions in the water and the injection of subsequent localization of space charge at the LDPE interface may cause significant ageing of the LDPE in the ambient medium [Citation16]. An analysis of the dielectric properties in polymeric materials over an extensive range of frequencies may provide an indicator of ageing. The consistent picture of the modifications in dielectric properties with progressive ageing could be regarded as a diagnostic indicator of the insulation’s operating properties. In that same sense, data from dielectric spectroscopy can then be functioned to produce appropriate electrical analogue models of the ageing process. This motivates us to conduct an extensive assessment of the dielectric properties of LDPE after various contact times in apple, cow’s milk, pineapple, and mango to gain a better understanding of the problem. Our goal is to use the dielectric properties of the LDPE after contact with these items as an indicator of polymer decomposition.
2. Experimentation methods
The Qatar Petrochemical Company’s LDPE grade(LA071) was used in this research. This LDPE was formed into sheets with a thickness of 50 ± 0.1 mm and then washed carefully. The LDPE materials come into contact with different foods (apple, pineapple, mango, and cow’s milk). The disc-shaped samples were then silver-painted and placed in a two-electrode sample holder. The surface area that was exposed was 1.4 cm2. The measurements were done in the open air with Hioki 3520 programmable automatic LCR metre (Hioki, Japan) set to room temperature, the frequency range of 100Hz-5 MHz, and operated at 0.80 V.
3. Result and discussion
The real part (ε′) and imaginary part (ε″) of permittivity are calculated for LDPE contact in apple, pineapple, mango, and cow’s milk. Figures show the Cole- Cole diagram for LDPE contact in apple, pineapple, mango, and cow’s milk, respectively, for different intervals of time. The ability to gain Cole–Cole plots suggests that the LDPE is ruled by complex polarization mechanisms with variable relaxation times.
Furthermore, the depression behaviour of the semicircle centres in LDPE (LA071) samples is directly linked to non-ideal capacitive properties. This non-ideal one implies that electrical conductivity or charge carrier movements are happening.
We can calculate the relative permittivity at zero frequency () and infinite frequency (
) by intersecting these circular arcs with the ε’ axis.
From Figure , significant parameters such as the oscillating strength of the dipoles L = –
, the macroscopic relaxation time (τ0), and the molecular relaxation time (τ) can be deduced.
τ0 could be determined as follows [Citation17]:
(1)
(1) since U is the distance between a specific experimental point and εo, and V is the distance between the same point and ε∞. The S value serves as an indicator for the dielectric relaxation time distribution width. If S = 0, there is only one dielectric relaxation time. For a distribution of relaxation time, S values lie between 0 and 1. τ could be assessed from [Citation18]
(2)
(2) Figures show the parameters ε∞, εo, and L clearly decreased exponentially with contact time of up to 7 days due to the formation of oxygenated groups such as carbonyl and hydroxyl groups. Furthermore, as contact time increases, chemical reactions between the LDPE and foods increase, resulting in a decrease in ε∞, εo, and L. However, as the time of contact increases, ε∞ and εo start to decrease slightly, which is attributing to the formation of the cross linking.
The calculated τ for the first relaxation mode vs. contact time in apple, cow’s milk, pineapple, and mango is shown in Figure . It is clear that τ in all cases, it follows the same trend. τ decreases with contact time up to 7 days, indicating that the molecules are oriented with the deriving ac field faster, resulting in smaller molecules. As a result, the LDPE contact causes LDPE degradation, which is the breakdown of a chemical compound into smaller molecules through photolysis, hydrolysis, or oxidization, in this range of contact time [Citation19–22]. Further increasing the LDPE’s contact with apple, cow’s milk, pineapple, and mango leads to an increase τ. This result is due to LDPE cross-link, which causes the molecules of LDPE to not pursue the field’s rapid oscillations at the same rapid oscillations [Citation23]. We can conclude from Figure that the degradation of LDPE when it comes into contact with cow’s milk is greater than that of other materials. However, when LDPE comes into contact with mango, it degrades the least. As result, the dielectric parameters of LDPE after contact with specific foods are significant indicators of LDPE degradation and other defects.
4. Conclusions
Chemical reactions between LDPE and foods cause significant changes in the dielectric properties of LDPE samples, depending on contact time and food type. Interestingly, the contact with cow’s milk causes the most LDPE degradation, whereas contact with mango causes the least LDPE degradation. It is advised that the results of the measured dielectric data be employed to predict LDPE degradation and other defects. More comprehensive dielectric investigations for many types of polymers after contact with many food items are desirable in future work.
Disclosure statement
No potential conflict of interest was reported by the author(s).
References
- Abutaleb A, Hussain S, Imran M. Systematic exploration of electrospun polyvinylidene fluoride (PVDF)/multi-walled carbon nanotubes’ (MWCNTs) composite nanofibres for humidity sensing application. J Taibah Univ Sci. 2021;15:257–266.
- Khalil MM, El-Sayed AH, Masoud MS, et al. Synthesis and optical properties of alizarin yellow GG-Cu(II)-PVA nanocomposite film as a selective filter for optical applications. J Mater Res Technol. 2021;11:33–39.
- Elghnam SM, Abdelalim YH, Hamad MAJ. Enhancement of the dielectric properties of low density polyethylene grad (LA071) via γ irradiation. Mater Res Technol. 2021;11:247–251.
- Razzaq S, Akhtar M, Zulfiqar S, et al. Adsorption removal of Congo red onto L-cysteine/rGO/PANI nanocomposite: equilibrium, kinetics and thermodynamic studies. J Taibah Univ Sci. 2021;15:50–62.
- Finner SP, Schilling T, van der Schoot P. Connectivity, not density, dictates percolation in nematic liquid crystals of slender nanoparticles. Phys Rev Lett. 2019;122:097801.
- Elghnam SM, Abdelalim YH, Hamad MA. Influence of weathering exposure on UV light absorption in low density polyethylene LDPE grad-LA071. J Mater Res Technol. 2022;19:1493–1496.
- El-Sayed AH, Hemeda OM, Tawfik A, et al. Remarkable magnetic enhancement of type-M hexaferrite of barium in polystyrene polymer. AIP Adv. 2015;5:107131.
- El-Sayed AH, Hemeda OM, Tawfik A, et al. Simulation of wasp-waisted magnetic hysteresis loop for NiCoP-coated BaFe12O19–polystyrene bilayer composite film. J Supercond Nov Magn. 2016;29(9):2451–2453.
- Hamad MA, El-Sayed AH, Hemeda OM, et al. Strong coercivity reduction and high initial permeability in NiCoP coated BaFe12O19–polystyrene bilayer composite. Mater Res Express. 2016;3(3):036104.
- El-Sayed AH, El-Shamy F, Hossien Y, et al. Optical and magnetic properties of polyvinyl alcohol films filled with CoCl2, NiCl2, and FeCl3. J Supercond Nov Magn. 2017;30(10):2927–2931.
- Namazi H. Polymers in our daily life. BioImpacts. 2017;7:73–74.
- Pillai O, Panchagnula R. Polymers in drug delivery. Curr Opin Chem Biol. 2001;5(4):447–451.
- Raj B. Low density polyethylene/starch blend films for food packaging applications. Adv Polym Technol. 2004;23:32–45.
- Scarpa PCN, Svatik A, Das-Gupta DK. Dielectric spectroscopy of polyethylene in the frequency range of 10−5 Hz to 106 Hz. Polym Eng Sci. 1996;36(8):1072–1080.
- Zeller HR. Breakdown and prebreakdown phenomena in solid dielectrics. IEEE Dam El Ins. 1987;EI-22:115–122.
- Bamji SS, Bulinski AT, Densley RJ, et al. Degradation mechanism at XLPE/semicon interface subjected to high electrical stress. IEEE Trans EL Ins. 1991;26:278–284.
- Raistrick ID, Macdonald JR, Franceschetti DR. Theory, Experiment, and Applications. In: E Barsoukov, JR Macdonald, editors. Impedance spectroscopy. New York: John Wiley; 2005. p. 27.
- Cole RH, Cole KS. Infra-red and Raman spectra of polyatomic molecules XIII. Nitromethane. J Chem Phys. 1941;9:314–318.
- Sharif J, Abdul Aziz S, Hashim K. Radiation effects on LDPE/EVA blends. Radiat Phys Chem. 2000;58:191–195.
- Seifali Abbas-Abadi M, Nekoomanesh Haghighi M,McDonald AG, et al. Estimation of pyrolysis product of LDPE degradation using different process parameters in a stirred reactor. Polyolefins J. 2015;2:39–47.
- Raut S, Raut S, Sharma M, et al. Enhancing degradation of low density polyethylene films by Curvularia lunata SG1 using particle swarm optimization strategy. Indian J Microbiol. 2015;55:258–268.
- Andersson T, Holmgren MH, Nielsen T, et al. Degradation of low density polyethylene during extrusion. IV. Off-flavor compounds in extruded films of stabilized LDPE. J Appl Polym Sci. 2005;95:583–595.
- Mohammed AZ, Kassem ME. Weather ageing characterization of the mechanical properties of the low density polyethylene. Polym Degrad Stabil. 1998;62:105–109.