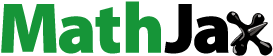
Abstract
The study aimed to evaluate the impact of UV-C dry seed priming at two doses (0.85 and 3.42 kJ.m−2), on tomato under salt stress (NaCl, 100 mM). Results show that salinity causes a regression of tomato growth by 63%, an accumulation of Na+ causing a reduction in K+ uptake and therefore an increase of photosynthetic processes (28, 28 and 23% for Chl. a, Chl. tot and Car., respectively), also an augmentation in soluble protein contents and a decrease or an increase in oxidative stress indicators and secondary metabolites depending on organs. UV-C seed priming has been shown to improve tomato growth by reducing the adverse effects of salinity on physiological and biochemical parameters. This research revealed that UV-C seed applications are an efficient approach to controlling the aggressiveness of salt stress on tomato roots and leaves. Specific doses of UV-C are required to trigger the physiological control of plants against salt stress.
1. Introduction
Salinization in arid and semi-arid ecosystems results from high water evaporation from the soil and irregular and insufficient rainfall [Citation1]. Consequently, plant growth and development can be limited by this constraint. Several studies have been carried out on several plant species, both spontaneous and cultivated, in order to specify their tolerance threshold to such a constraint, on the one hand, and to identify the mechanisms involved in their ability to grow under these conditions, on the other [Citation2]. Another more recent approach has been developed in recent years to address this major constraint to agricultural production. This approach is known as priming.
Priming in agriculture is a form of seed planting preparation in which seeds are first subjected to pre-treatment. In general, most seeds used in experiments have shown an overall advantage over seeds that are not pre-treated [Citation3]. Generally, seed priming leads to more rapid and regular germination when pre-treated seeds are subsequently planted [Citation3]. It is a technique that has been used to treat seeds and induce an increase in the germination rate to enhance the emergence of seedlings, especially under adverse conditions [Citation4]. In several previous studies, priming was hormonal or saline solutions but recently a new priming technique is used by ultraviolet C-band radiation (UV-C) on dry seeds.
The visible light produced by the sun can be broken down into several types of electromagnetic radiation such as visible radiation, IR (infrared radiation) or UV (ultraviolet radiation). Stratospheric ozone acts as a real filter since it absorbs almost all UV-B radiation (wavelength varies from 280 nm to 315 nm) and completely blocks UV-C radiation (wavelength is less than 280 nm) [Citation5]. UV-B light is widely studied in the context of stimulating plant defences [Citation6]. Unfortunately, exploiting UV-B poses practical problems. In general, prolonged exposure is required to be effective and they are difficult to implement in practice [Citation6]. It is in this sense that the idea of exploiting UV-C was born, as being highly energetic, it requires shorter treatment times and is, therefore, easier to manage in the case of a field application.
An important idea emerges: if radiation can be used to increase the concentrations of micronutrients in plants, which a number of studies clearly show, it should also be possible to use this same radiation to stimulate the plants’ defences. And, indeed, there are a few articles showing that plant defences can be stimulated by exposing them to certain wavelengths, to UV, for example, or better, UV-C [Citation7–9].
Clearly, the latter emerges from the various studies with remarkable potential, at least equal to that of chemical stimulants of plant defences [Citation10]. Studies on lettuce suggest that seeds exposed to UV-C radiation induce in the plant an ability to grow under high salinity conditions [Citation7]. Initial findings indicate that UV-C radiation may trigger adaptation mechanisms to abiotic constraints at an early stage of treatment on the seed. UV-C radiation is perhaps the least studied of the electromagnetic radiations, but this needs to change as it appears capable, in the right dose, of generating many very positive effects for plants and crops.
In this work, this challenge will be achieved by studying the effects of UV-C, applied in different forms to tomato seeds, on plant growth under NaCl, 100 mM. We were interested in the induction of plant defence reactions by UV-C radiation on dry tomato seeds. We, therefore, examined the impact of UV-C light when applied to ungerminated seeds on (i) the sensitivity level of plants to different UV-C primed seed doses, (ii) the impact of UV-C priming on the ability of tomato to grow in a saline environment.
2. Material and methods
2.1. Seed irradiation with UV-C
The UV-C dose depends on the irradiation frequency of the UV-C lamp, the distance to the light source and the time of exposure to the radiation. The lamp used emits quasi-monochromatic UV radiation at 254 nm. All tomato seeds except those used as a control were irradiated with two lamps with a normal output power of 60 W. The applied doses were simply obtained by multiplying the power by the exposure time.
Tomato (Solanum lycopersicum) seeds were divided into three batches: a control batch that did not receive UV-C irradiation, a batch treated with a dose of 0.85 kJ.m−2 and a third batch exposed to 3.42 kJ.m−2. In a previous study on lettuce (Lactuca sativa) [Citation7], several doses of UV-C were tried and two doses were selected: one deleterious and one optimal (called hormic). In this work, we used these two doses to study the growth of tomato plants after seed irradiation with different doses of UV-C, on the one hand, and on the other hand, its behaviour under salt stress.
2.2. Germination in Petri dishes
Tomato seeds were divided into three 200 ml beakers, the first contains the control seeds (not irradiated with UV-C), the second contains those irradiated at a dose of 0.85 kJ.m−2 and the third contains those irradiated at a dose of 3.42 kJ.m−2. These seeds were disinfected with calcium hypochlorite at a rate of 3 ml per beaker. After two successive rinses with distilled water, they were soaked in three small beakers for two hours, then transferred to Petri dishes covered with filter paper imbibed with distilled water, at a rate of 25 seeds per dish. They were subsequently incubated in an oven at 30°C in the dark for 7 days. Germination was indicated by the emergence of a 3–5 mm long radicle.
2.3. Plant material and culture conditions
After 7 days of incubation, the dishes were transferred to a culture chamber with a photoperiod of 16 h during the day/8 h at night and an effective radiation of 150 µmol. m−2. s−1. The means temperature and relative humidity were 22 ± 2° C and 60 ± 5% during the day and 18 ± 2°C and 80 ± 5% at night, respectively. The transplanting, which consists of transferring seedlings into 330 ml pots at a rate of one plant per pot, was carried out two days later. The culture was carried out in hydroponics.
At the age of 30 days, plants from each batch of seeds were divided into two batches. The first batch, serving as a control, was irrigated with Hoagland and Arnon [Citation11] nutrient solution diluted four times (T/4 solution) (Based on preliminary experiments [Citation12]). The second was irrigated with the same solution, but with 100 mM NaCl added. Hoagland solution was renewed weekly.
After a 15-day treatment, the plants were then harvested and divided into shoots and roots. Several parameters were determined before (root elongation, stem elongation, leaf number, etc.) and after final harvest of plants from three treatments (fresh and dry weights, leaf area, water content and Na+, K+ contents of different organs).
Morphogenesis was monitored by counting leaf numbers. The plant lengths were determined using a graduated ruler and expressed in centimetres (cm). Leaf area was measured using Optimas software. The specific leaf area was calculated as the ratio of the total leaf area to the leaf dry weight and the leaf thickness was calculated as the ratio of leaf water content and the total leaf area.
The degree of leaf succulence and the degree of leaf sclerophylly were determined as the ratio of the amount of water and dry weight to the total leaf area, respectively.
Water content (ml g−1 DW) was estimated by the difference between the fresh weight (FW) and dry weight (DW), referred to the unit mass of DW.
Shoot and root fresh weight (FW) was measured using an analytical balance, whereas dry weight (DW) was determined after drying plant samples in an oven under at 75°C for 48 h. For Na and K measurements, the dry matter was treated with 5 ml of 0.1 N HNO3 for 48 h. Na+ and K+ contents in the clear extracts were measured by flame photometry (Jenway PFP7, United Kingdom) using a butane flame.
Six plants from each treatment were harvested and used for the determination of photosynthetic pigments, anthocyanins, proteins, MDA and H2O2 measurements and total polyphenols and tannins.
For all parameters studied in this work, six different plants were used as replicates.
2.4. Quantification of pigments (chlorophylls and carotenoids)
Leaf fragments were incubated in an 80% acetone solution. After 72 h in the dark and at a temperature of 4°C, the acetone extracts were measured with a “Beckman” spectrophotometer at 470, 646 and 663 nm to calculate the contents of chlorophyll a, chlorophyll b, total chlorophyll and total carotenoids according to the equation proposed by Lichtenthaler [Citation13]:
A: absorbance
The contents are expressed in µg/g fresh matter.
2.5. Determination of anthocyanins
The leaf anthocyanin content was assayed following the methodology of Murray and Hackett [Citation14]: 0.2 g of leaf fragments were incubated in the presence of a solvent mixture: HCl-H2O-MeOH (1/3/16) in the dark and at 4°C for 72 h. Two different wavelengths were used for the absorbance reading, namely 653 and 530 nm. The anthocyanin level was calculated as follows: Anthocyanins (µg/ml) = A530 − (0.24 × A653). The soluble anthocyanins in this solution show a maximum absorption spectrum at 530 nm, the subtraction of (0.24 × A653) compensates for the excess absorption at 530 nm due to the presence of chlorophylls [Citation15].
2.6. Protein content
Frozen roots and leaves of tomato plants were crushed in poly-vinyl-polypyrrolidone (PVP) and homogenized in 50 mM potassium phosphate buffer (pH 7), containing 0.1 mM Ethylenediaminetetraacetic (EDTA), 0.1 mM phenyl-methyl-sulfonyl-fluoride (PMSF) acid and 1 mM DTT. The mix was centrifuged at 12,000 g at 4°C for 30 min. The individual extract protein content was estimated by Bradford [Citation16] reaction using BSA (bovine serum albumin) as a reference standard.
2.7. Determination of malondialdehyde (MDA)
Lipid peroxidation was measured by the use of the TBA (Thiobarbituric Acid) reaction to determine the tissue level in MDA according to the method of Hodges et al. [Citation17].
Samples of 200 mg each of tomato roots and leaves were ground in the presence of liquid nitrogen in a porcelain mortar. The powder obtained was suspended in 1 ml of trichloroacetic acid (TCA, 0.1%). The mixture was centrifuged for 15 min at 15,000 g at 4°C.
To a volume of 0.5 ml of supernatant was added a volume of 1 ml of thiobarbituric acid (TBA, 0.5%) prepared in 20% TCA. This was incubated for 30 min in a water bath at 100°C. Under these conditions, the aldehyde compounds, mainly malondialdehyde, will react with TBA to form the TBA-MDA complex. The reaction was stopped by immediate cooling in crushed ice for 10 min. The supernatant was collected for colorimetric determination of the lipoperoxides. The absorbance was read at 532 and 600 nm against a blank containing a mixture of TBA-TCA. After subtracting the non-specific abundance read at 600 nm, the concentration of malondialdehyde (expressed in µmol g−1 FW) was calculated using its extinction coefficient (ϵ = 155 mM−1.cm−1) according to the following equation:
V(cuve): volume of the cuve = 1 ml; V(supernatant): volume of the supernatant extract in ml; V(TBA + supernatant): total volume of TBA and supernatant extract in ml; FW: root or leaf fresh weight in g
2.8. Determination of hydrogen peroxide (H2O2)
Hydrogen peroxide contents were measured as described by Junglee et al. [Citation18].
Fresh samples (200 mg) of each of the roots and leaves were homogenized in 1 ml of 0.1% (w:v) trichloroacetic acid (TCA) at 4°C. The homogenate is centrifuged at 15,000 g for 15 min at 4°C. Three 50 µl aliquots of each tube were placed in a 96-well Elisa microplate and 50 µl of phosphate buffer (pH = 7) and 100 µl of KI are added to each well. Each plate also contains increasing amounts of H2O2 to generate a calibration curve. The plate was briefly shaken, incubated at room temperature for 30 min and then the absorbance of each well was determined at 390 nm in a microplate reader (Power Wave HT Microplate Spectrophotometer from Bio Tek). The H2O2 contents of the different samples were determined using the calibration curve.
2.9. Total phenolic content determination
The total phenolic level of root and leaf extract was estimated based on the Folin–Ciocalteu reagent amended by Cicco et al. [Citation19] using gallic acid as a standard. An aliquot of sample extract was mixed with 0.5 and 0.125 ml of distilled water and the Folin–Ciocalteu reagent, respectively. The mixture was stirred and allowed to stand for 6 min before the addition of 1.25 ml Na2CO3, 7%. The resultant solution was then supplemented to a final volume of 3 ml with distilled water, mixed thoroughly and maintained for 90 min at room temperature. After incubation in the dark, the absorbance at 760 nm was determined. Total phenolic activity was calculated as mg gallic acid equivalents per g dry weight (mg GAE g−1 DW) based on the standard gallic acid calibration curve.
2.10. Total condensed tannin determination
The proanthocyanidins (or condensed tannins) were measured using spectrophotometer [Citation20]. Three millilitres of methanol vanillin, 4% and 1.5 ml of H2SO4 were mixed with 50 µl of well-diluted sample. The absorbance was measured at 500 nm relative to the solvent as a blank.
2.11. Statistical analyses
Analysis of all data was first performed for a normal allocation using Fisher's Student's t-test, then by variance analysis (two-way ANOVA) using Statistica® software. Means (±standard error) were segregated and classified using NewmaneKeuls for the post hoc test (P = 0.05).
3. Results
3.1. Effects of UV-C seed priming on growth and development of plants
Morphological parameters. Morphogenesis was monitored by counting leaf numbers, leaf stage numbers and by measuring leaf area, stem length and root elongation. Degrees of leaf sclerophylly and leaf succulence were deduced from different growth parameters measured.
Plants grown from UV-C primed seeds without saline treatment show a difference in growth of some parameters that were dependent on UV-C doses. Indeed, dose of 0.85 kJ m−2 showed a decrease in root elongation, total leaf area and individual leaf area (9, 15 and 21%, respectively, in comparison to plants from UV-C seeds non-primed (NPr) and without NaCl) and augmentation in leaf thickness and leaf succulence. The opposite was observed for plants grown from UV-C, 3.42 kJ m−2 except for individual leaf area and leaf succulence parameters (Table ).
Table 1. Morphological parameters. Thirty-day-old plants from UV-C seeds primed (0, 0.85 and 3.42 kJ m−2). Plants were grown for 15 days in absence or presence of NaCl, 100 mM. Mean of six plants and confidence interval for P = 0.05. Mean values with the same letter are not significantly different at P = 0.05 (ANOVA and mean comparison with Newman-Keuls post hoc test).
For other parameters, results do not show any significant difference compared to control plants (non-priming and without NaCl).
Exposition to salinity conditions caused a statistically important diminution of most morphological parameters except for root elongation, leaf stage number and leaf thickness. This decrease was reported on stem elongation, leaf numbers, total leaf area, individual leaf area, leaf sclerophylly and leaf succulence which was about 43, 11, 25, 16, 50 and 59%, respectively, compared to control (NPr and 0 mM NaCl).
Plants cultivated from seeds primed with various doses of UV-C, exhibit a reduction or augmentation of these parameters, but are affected less under salt stress, particularly with UV-C, 0.85 kJ m−2 that, in some cases, values registered were similar to those of seeds primed and grown in the absence of NaCl (Table ).
Plant growth and water content. Examination of Figure shows a statistically significant decrease in dry biomass production of control plants from UV-C primed seeds (51 and 36% for roots and 10 and 25% for leaves, with 0.85 and 3.42 kJ m−2 UV-C, respectively). Presence of 100 mM NaCl leads to a diminution of the dry weight mass of roots and leaves by 63% in comparison to control (NPr and 0 mM NaCl). The effect of UV-C seed priming of tomato was very significant on biomass production of roots and even of photosynthetic organs of plants cultivated under salt in culture medium. Indeed, a clear improvement on the growth of these organs was noticed, in particular with 0.85 kJ m−2 UV-C at the roots level.
Figure 1. Dry weights (DW) and water content of roots and leaves of tomato. Thirty-day-old plants from UV-C seeds non-primed (NPr) or primed (0.85 and 3.42 kJ m−2). Plants were grown for 15 days in absence (C) or presence (S) of NaCl, 100 mM. Mean of six plants and confidence interval for P = 0.05. Mean values with the same letter in each panel are not significantly different at P = 0.05 (ANOVA and mean comparison with Newman-Keuls post hoc test).
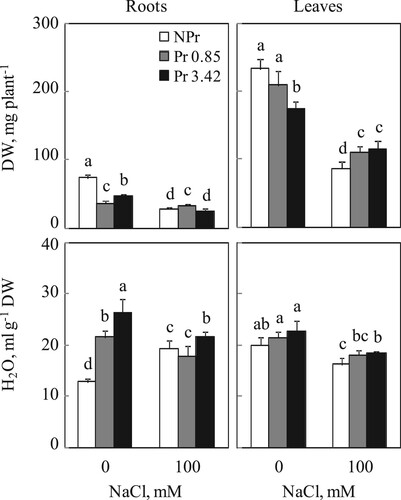
Water contents of roots and leaves of NPr and Pr plants developed without or with NaCl, 100 mM were summarized in Figure . There was a significant increase in hydration of underground organs for plants grown from UV-C primed seeds. This augmentation was much higher with the 3.42 kJ m−2 UV-C, in which a double value was recorded compared to the control plants (NPr and 0 mM NaCl). Whereas no disturbance of this parameter was recorded in photosynthetic organs. Salinity has a positive effect on hydration of roots and a negative effect on hydration of leaves of NPr plants. On the other hand, no significant difference in water content was recorded for Pr plants (0.85 kJ m−2 UV-C), but a slight increase in water content was recorded for Pr plants (3.42 kJ m−2 UV-C) compared to NPr plants grown in the presence of NaCl 100 mM (Figure ).
3.2. Effects of UV-C seed priming on ionic characteristics
Examination of Figure shows that photosynthetic organs accumulate more Na+ than below-ground organs, while indicating a clear difference between plants from UV-C primed seeds (Pr) and NPr plants grown in the presence of NaCl. Indeed, UV-C priming lowers Na+ content in roots and leaves. This decrease was estimated to be 33 and 20% in roots and 30 and 40% in leaves with 0.85 and 3.42 kJ m−2 UV-C, respectively.
Figure 2. Sodium and potassium contents in roots and leaves of tomato. Plants were grown as described in the legend of Figure . Mean of six plants and confidence interval for P = 0.05. Mean values with the same letter in each panel are not significantly different at P = 0.05 (ANOVA and mean comparison with Newman-Keuls post hoc test).
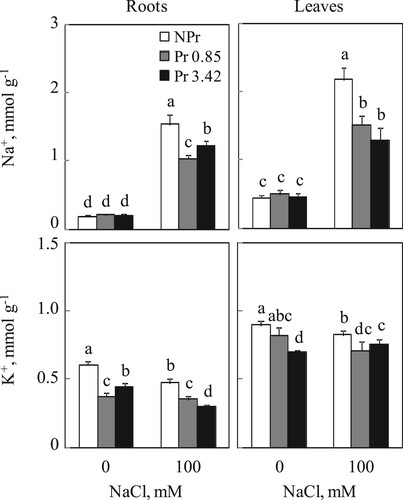
For potassium contents, Figure shows that exposure of seeds with various doses of UV-C lead to a decrease in K+ accumulation, particularly with 0.85 kJ m−2 UV-C in roots (38% compared to NPr plants) and with 3.42 kJ m−2 UV-C in leaves (22% compared to NPr plants) of plants grown in absence of salt. In presence of NaCl, potassium content was reduced in NPr plants both in below-ground and photosynthetic organs. This reduction was partially compensated in roots and leaves of plants primed with 0.85 and 3.42 kJ m−2 UV-C, respectively.
3.3. Effects of UV-C seed priming on chlorophyll, carotenoid and anthocyanin contents
In the present study, contents of photosynthetic pigments, such as chlorophylls a, b, total and carotenoids expressed in mg. g−1 of FW, as well as anthocyanin content of tomato were reported in Table . It was clear that chlorophyll a content dominates that of chlorophyll b for NPr and Pr plants cultivated in both absence and presence of NaCl, 100 mM. Thus, contents of this pigment, generally for all treatments, were 2 times higher than those of chlorophyll b (Table ).
Table 2. Amount of chlorophyll a (Chl. a), chlorophyll b (Chl. b), total chlorophyll (Chl. tot), total carotenoids (Car.) and anthocyanins (Anth.) in leaves of tomato. Plants were grown as described in the legend of Table . Mean values with the same letter are not significantly different at P = 0.05 (ANOVA and mean comparison with Newman-Keuls post hoc test).
Table shows that chlorophyll a, b, total and carotenoid contents of Pr plants were clearly influenced by the high dose of UV-C 3.42 kJ m−2 applied to the dry seeds. Measurements show high values compared to control (NPr) and even compared to Pr plants from UV-C primed seeds (0.85 kJ m−2), which show nearly the same values to the NPr plants.
The availability of 100 mM NaCl in NPr plants, leads to an increase in total chlorophylls content due to that of fraction a and even carotenoids (28, 28 and 23% compared to the control, NPr without NaCl, for Chl. a, Chl. tot and Car, respectively). On the other hand, plants from UV-C primed seeds (0.85 kJ m−2) restored the level of photosynthetic pigment accumulation in salt-treated plants. Thus, values remained close to those of plants NPr and Pr grown in absence of NaCl. However, results reveal a decrease in these contents in salt-treated plants (Pr plants from UV-C primed seeds 3.42 kJ m−2) compared to those of control plants (Pr without NaCl).
As for anthocyanin contents, Table shows that no disturbance of these contents was recorded in NPr and Pr plants grown in absence of salt. In contrast, NaCl increased anthocyanin levels in NPr plants, but UV-C seed priming restored anthocyanin contents especially in Pr plants from seeds primed with UV-C 0.85 kJ m−2 than 3.42 kJ m−2. This shows a positive regulatory effect of UV-C priming with NaCl on anthocyanin contents.
3.4. Effects of UV-C seed priming on protein contents
In the absence of NaCl, roots of plants from primed seeds show high protein contents that even exceed those of control plants (NPr). The same was observed in leaves, except that UV-C 3.42 kJ m−2 resulted in a more pronounced enhancement of the protein content of plants from seeds primed with UV-C. Thus, these contents were 1.25, 1.46 and 1.44 mg g−1 FW in roots and 1.33, 1.65 and 2.10 mg g−1 FW in leaves for the NPr, 0.85 and 3.42 kJ m−2 treatments of plants grown in the absence of NaCl, respectively (Figure ).
Figure 3. Proteins contents in roots and leaves of tomato. Plants were grown as described in the legend of Figure . Mean of six plants and confidence interval for P = 0.05. Mean values with the same letter in each panel are not significantly different at P = 0.05 (ANOVA and mean comparison with Newman-Keuls post hoc test).
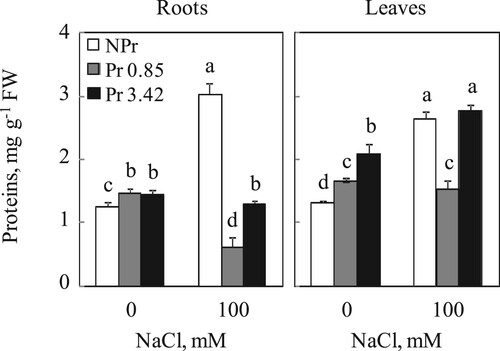
Sodium chloride treatment increased protein contents of both underground and photosynthetic organs. UV-C seed priming restored this increase in both organs, except that the high dose of UV-C (3.42 kJ m−2) did not restore these levels which were similar to those of NPr plants treated with NaCl, 100 mM (Figure ).
3.5. Effects of UV-C seed priming on MDA and H2O2 contents
In control medium for both NPr and Pr plants, MDA levels in roots were comparable, around 0.3 µmol. g−1 FW. On the other hand, in leaves, high values (0.9 µmol. g−1 FW) were recorded in NPr control plants compared to Pr plants. A remarkably elevated MDA content was noted in roots of NPr and Pr salt-treated plants (UV-C 0.85 kJ m−2), except for the UV-C seeds primed 3.42 kJ m−2 which exhibited a decrease of MDA content, 71% compared to control (Pr without salt) (Figure ). As for leaves, MDA contents decreased significantly for NPr and Pr plants (UV-C 0.85 kJ m−2), but an increase was detected for Pr plants (UV-C 3.42 kJ m−2) (Figure ).
Figure 4. Lipid peroxidation (MDA) and Hydrogen peroxide (H2O2) contents in roots and leaves of tomato. Plants were grown as described in the legend of Figure . Mean of six plants and confidence interval for P = 0.05. Mean values with the same letter in each panel are not significantly different at P = 0.05 (ANOVA and mean comparison with Newman-Keuls post hoc test).
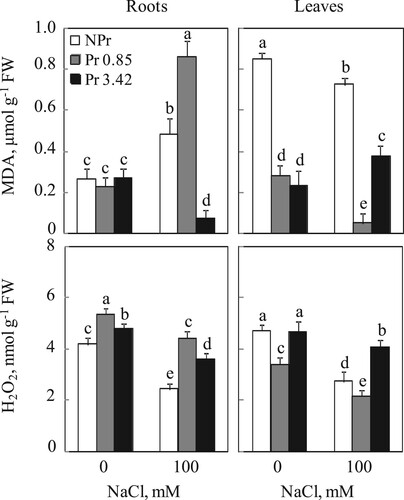
Concerning hydrogen peroxide contents, it was clear that UV-C irradiation on dry seeds has influenced the contents of this parameter in roots as well as in leaves independently of salt treatment. Indeed, the low dose of UV-C leads to a significant increase of H2O2 in roots (28% compared to NPr control without salt) but decreases it in leaves. High UV-C dose also increase H2O2 levels in roots (16% compared to NPr without salt) but they remained comparable to those of NPr plants without salt in leaves (Figure ). Salinity induced a decrease in H2O2 levels in both underground and photosynthetic organs. This decrease clearly varied with UV-C priming doses (Figure ).
3.6. Effects of UV-C seed priming on polyphenol total and tannin contents
Determination of total polyphenols was carried out on a dry matter basis (Figure ). In the control conditions (0 mM NaCl), the root levels of these components exhibited comparable values (with a significant slight variation) for both treatments (NP and Pr). While for foliar concentrations, UV-C seed priming 0.85 kJ m−2 showed high values of these compounds. The levels reached 2 mg GAE g−1 DW. Under 100 mM NaCl, a reduction in total polyphenol levels was recorded in plant roots from NPr and Pr seeds (UV-C, 0.85 kJ m−2) (43 and 45% compared to plants grown without NaCl, respectively). UV-C seeds primed 3.42 kJ m−2 did not alter these contents compared to those of control plants (Pr with 100 mM NaCl). For leaves, only UV-C primed seeds 0.85 kJ m−2 which led to an increase in polyphenol total content reached 3 mg GAE g−1 DW, compared to NPr and Pr (UV-C, 3.42 kJ m−2) where the values were comparable to those of control plants (without NaCl) (Figure ).
Figure 5. Total phenolic and total condensed tannin contents in roots and leaves of tomato. Plants were grown as described in the legend of Figure . Mean of six plants and confidence interval for P = 0.05. Mean values with the same letter in each panel are not significantly different at P = 0.05 (ANOVA and mean comparison with Newman-Keuls post hoc test).
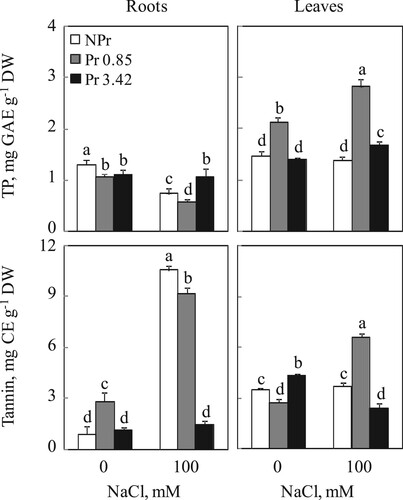
Fluctuations in tannin contents of roots and leaves were shown in Figure . On control medium (without NaCl), root extracts from Pr seeds (UV-C 0.85 kJ m−2) showed higher tannin contents than those from NPr or Pr seeds (UV-C 3.42 kJ m−2). In roots of salt-treated plants (NPr and Pr seeds, UV-C 0.85 kJ m−2), we found a significant positive impact on tannin content reached 10.5 and 9.1 respectively mg CE g−1 DW. While no difference was recorded in UV-C primed seeds 3.42 kJ m−2 compared to control (without NaCl). Regarding leaf tannin content, in contrast to the roots, control plants from UV-C primed seeds (0.85 kJ m−2) showed the lowest tannin contents. The presence of salt in the growing medium had no effect on the foliar tannin content of NPr plants, but increased and decreased that of UV-C primed seeds, 0.85 and 3.42 kJ m−2, respectively.
4. Discussion
Initially, UV-C radiation was commonly used because of its germicidal power on microorganisms [Citation5]. This disinfection power is very interesting but can, however, be harmful to plants.
Interest in studying the impact of UV radiation on plants is growing, given the adverse effects, it can have on plant physiology and, consequently, on growth of plants. In spite of these negative effects, UV-C radiation can have protective effects against biotic and protective effects against biotic and abiotic stresses that plants are subjected to. For example, it can be used to stimulate the defence reactions of horticultural products against post-harvest pathogens [Citation10]. In addition, UV-C light treatments can be used to exploit to increase the storage life of vegetables and fruits [Citation10]. Post-harvest treatment of some vegetables and fruits can also increase their content of bioactive compounds beneficial to human health [Citation10].
A major challenge for us in the current study was to identify UV-C doses that were both effective in inducing tomato resistance to NaCl, without being deleterious to the plants, and at the same time improving the ability to defend against salt stress. We used data obtained from published literature [Citation7] to identify the doses of UV-C that we chose for our assays. Ouhibi et al. [Citation7] documented that, plants of Lactuca sativa from primed seeds exposed to non-deleterious doses of UV-C showed a decrease in NaCl, 100 mM sensitivity. The strategy of stimulating plant defences by UV-C radiation in order to effectively combat abiotic stress could perhaps come back to studying the phenomenon of “priming”. “Priming” or potentialization is therefore the preparation of the plant to a physiological status that allows the plant to induce its defence mechanisms quicker and or more intensely when subjected to abiotic constraints [Citation21]. It is with this hypothesis that we have oriented our working ideas.
The underlying mechanisms of the control of abiotic constraints after UV-C seed treatments are unknown. To establish if UV-C exposure has an impact on plant development, a treatment of 0.85 kJ m−2 was applied with the dose of 3.42 kJ m−2 to determine if increased doses of UV-C adversely affect plant development. A reduced effect was noted on root elongation, total leaf area and individual leaf area with the 0.85 kJ m−2 dose, and on leaf stage number, leaf number, leaf sclerophylly and leaf succulence with the 3.42 kJ m−2 dose.
UV-C primed seeds, 0.85 kJ m−2, resulted in statistically significant bioinhibition of root growth and K+ levels compared to NPr plants (0 mM NaCl), while the 3.42 kJ m−2 dose exhibited reduced growth and potassium levels in both roots and leaves. This is supported in part by the results found by Ouhibi et al. [Citation7] for dry biomass of lettuce plants, but not for tissue potassium loading. Indeed, Ouhibi et al. [Citation7] showed high leaf and root potassium contents compared to control plants (NPr). This suggests that the dose and mode of application of a UV-C treatment must be defined for each plant species and variety in order to define a favourable (enhanced levels of resistance) and non-deleterious (enhanced in susceptibility) effect. Some previous research has also found that optimal dose of UV-C to obtain positive benefits on plants depends on species of plant [Citation9]. In our information, there are few works showing the impact of plant variety on the efficiency of UV-C radiation treatment.
UV-C has even more deleterious effects on plants and microorganisms, but can also create more beneficial effects at low doses [Citation9]. The application of UV-C radiation to the plant at the vegetative stage has an impact on the efficiency of photosynthesis by reducing the rate of CO2 assimilation, stomatal opening and intracellular CO2 concentration [Citation22]. The parameter measuring chlorophyll a fluorescence, Fv/Fm, confirms this loss of photosynthetic efficiency. Strawberry leaves treated with UV-C during their cultivation phase have a lower Fv/Fm and a reduction in leaf dry matter [Citation22]. In this work, UV-C was applied to dry, ungerminated seeds. Therefore, it is necessary to know what happened in the seeds after this irradiation. UV-C has been reported to induce phytoalexin accumulation in many crops, including grapes [Citation23], carrots [Citation24] and tomatoes [Citation25]. Stimulation of the synthesis of other defence molecules can also take place under UVC, particularly in tomato, such as PR-proteins [Citation26] or molecules constituting structural barriers (cellulose, hemicellulose and pectin) in strawberry [Citation27]. Other diverse effects have also been observed, such as the slowing down of maturation and the softening of tissues in tomatoes, or the delaying of senescence, and stimulate the accumulation of secondary metabolites, especially phenolic compounds [Citation28].
These phenomena may be in part explained by the fact that UV-C leads to a slowing down of respiration and ethylene production in tomatoes, thus delaying ripening. UV-C also maintains a higher level of putrescine in the tissues (a polyamine with a physiological effect opposite to ethylene) in tomato, which will delay senescence [Citation29].
Growing tomato on saline medium (100 mM) led to a significant reduction in stem length, leaf number, leaf area, degree of succulence and degree of sclerophylly after 15 days of treatment. These results are in agreement with those found by Oztekin and Tuzel [Citation30] who showed that the presence of 200 mM NaCl reduced tomato height by 29% compared to the control. Attia [Citation31] also show that salinity causes a decline in growth, which is indicated by a decrease in leaf area. Aldesuquy et al. [Citation32] and Rebah et al. [Citation12] also showed a diminution of the degree of succulence and sclerophyll following salt treatment in two wheat cultivars and two tomato introgression lines. This can be explained by a lower water retention in leaves during growth in the presence of NaCl [Citation32]. These negative impacts of salinity on these parameters were compensated in plants grown from UV-C primed seeds, to different degrees depending on the UV-C dose applied to seeds. This was confirmed by Ouhibi et al. [Citation7] in lettuce.
The consequences of salt stress were manifested by a restriction of root and leaf dry matter compared to control and a perturbation of the nutritional status, thus restricting the uptake, transport and the accumulation of essential ions for plant growth. Our results showed that NaCl, 100 mM leads to a higher preferential accumulation of sodium in leaves than in underground organs, this is due to the specific structures including the sodium secreting glands. These results are in accordance with the work of Rebah et al. [Citation12] who discovered that the impact of NaCl, 100 mM on the growth of tomato plants was manifested by a significant diminution of dry biomass and a decrease in the absorption of K+. Pre-treatment of tomato seeds improved growth for both roots and leaves, only a UV-C dose effect is present. In salt-treated plants, UV-C priming lowers sodium levels in roots and leaves. The capacity of plants to neutralize salt stress depends strongly on the state of their potassium nutrition. Any change in K+ status (K+ deficit) affects the growth by limiting cell expansion and restricting photosynthetic functions [Citation33]. To avoid cell damage, plant cells need to maintain high K+ nutrition in the cytosol.
From the results reported in Table , it was clear that a significant enhancement of Chl a, Chl b and carotenoid contents in plants grown from UV-C primed seeds at the doses of 0.85 and 3.42 kJ m−2. It may be that higher carotenoid levels contributed to protecting chloroplasts from photooxidative stress [Citation8,Citation34]. The increases were coherent with increased protein levels observed in plants Pr (0.85 and 3.42 kJ m−2). This was shown by Rai et al. [Citation35] specifying antioxidant enzymes as soluble proteins. In addition, we noted an elevation of total polyphenolic level in leaves of plants issued from UV-C primed seeds at the doses of 0.85 kJ m−2compared to control plants (NPr, without NaCl). Comparable results were reported by Forges et al. [Citation9]. These findings are coherent with the development of phenolic compounds, like suberin and lignin, which are deposited on the wall of cells and strengthen the protection against pathogenic attacks [Citation9]. In parallel to these results, we note that only H2O2 contents, as an indicator of oxidative stress, increased significantly in the roots of plants grown from UV-C primed seeds.
UV-C radiation causes the induction of phenolic compounds [Citation28]. These compounds have a major potential role in plant photoprotection because of their antioxidant characteristics [Citation36]. The enzyme PAL (phenylalanine ammonia-lyase) catalyses the conversion of L-phenylalanine to trans-cinnamic acid in the phenylpropanoid pathway which, together with the shikimate pathway, is the origin of all phenolic compounds. In our work, UV-C radiation causes an augmentation of the content of phenolic compounds. Among all phenolics, UV-C treatments stimulate the synthesis of flavonoids, including anthocyanins and tannins, which are derived from p-coumaric acid of the phenylpropanoid pathway via the action of CHS (chalcone synthase) [Citation29]. In tomato, the carotenoid content is increased following application of UV-C radiation [Citation37].
Salt stress caused an elevation of chlorophylls, carotenoids, anthocyanins, proteins, MDA in leaves, and tannins content in roots and a decrease in foliar MDA content, H2O2 and in roots total polyphenol contents. These impacts have been attenuated in UV-C primed seed plants.
Seeds with greater antioxidant content may contain biochemical characteristics that help their seedlings survive in adverse conditions [Citation38].
It may be proposed that UV-irradiated tomato seeds in this research exhibited enhanced activity of antioxidant plant responses that can reflect the enhanced plant development displayed by seedlings grown from seeds irradiated with UV-C in comparison to unirradiated seeds under salt stress conditions.
The passage from seed dormancy to seedling via germination is an important stage of development in the lifecycle of a plant. It is the equilibrium state between the abscisic acid, that leads to the dormant state and germination-promoting gibberellins [Citation39].
5. Conclusions
Our observations suggest that exposure of tomato seeds to UV-C light at pre-germination improves the seedling tolerance against salinity stress. Nevertheless, the application of two different doses influenced the degree of resistance of plants to salinity constraints. Indeed, the 0.85 kJ m−2 treatment mitigated NaCl-induced stress by keeping root biomass, root potassium supply and leaf protein contents comparable to those of the control (Pr, without NaCl). The 3.42 kJ m−2 treatment stimulated tomato resistance to NaCl by keeping protein, total polyphenol and tannin contents of the roots comparable to those of the control (Pr, without NaCl).
This study suggests, moreover, that UV-C radiations can trigger mechanisms of acclimation to abiotic stress in plants, and this as early at the seed stage. Our observations rise several questions: (i) which strategies of salt tolerance are involved and how is quality impacted? (ii) which role plays the age of seeds at the time of treatment and how long does the treatment holds its effect? (iii) how are UV-C radiation perceived by seeds and involved in priming mechanisms that confer salt tolerance to plants much after germination?
Acknowledgements
The authors acknowledge with thanks DSR for technical and financial support. K. H. A. and H. A. are indebted to Dr. Chayma Ouhibi, Dr. Fedia Rebah and Dr. Walid Zorrig for their help.
Disclosure statement
No potential conflict of interest was reported by the author(s).
Additional information
Funding
References
- Youssef AM, Pradhan B, Sabtan AA, et al. Coupling of remote sensing data aided with field investigations for geological hazards assessment in Jazan area, Kingdom of Saudi Arabia. Environ Earth Sci. 2012;65:119–130.
- Attia H, Ouhibi C, Ellili A, et al. Analysis of salinity effects on basil leaf surface area, photosynthetic activity, and growth. Acta Physiol Plant. 2011;33:823–833.
- Hossinifarahi M, Moazen HA, Amiri A, et al. Evaluation of seed priming and culture media to improve the germination performance and quality of sweet pepper and eggplant seedlings. Int J Hortic Sci Technol. 2022;9(4):415–428.
- Hussain M, Farooq M, Sattar A, et al. Mitigating the adverse effects of drought stress through seed priming and seed quality on wheat (Triticum aestivum L.) productivity. Pak J Agric Sci. 2018;55:313–319.
- Tran HDM, Boivin S, Kodamatani H, et al. Potential of UV-B and UV-C irradiation in disinfecting microorganisms and removing N-nitrosodimethylamine and 1,4-dioxane for potable water reuse: a review. Chemosphere. 2022;286:131682.
- Meyer P, Van de Poel B, De Coninck B. UV-B light and its application potential to reduce disease and pest incidence in crops. Hortic Res. 2021;8:194.
- Ouhibi C, Attia H, Rebah F, et al. Salt stress mitigation by seed priming with UV-C in lettuce plants: growth, antioxidant activity and phenolic compounds. Plant Physiol Bioch. 2014;83:126–133.
- Vàsquez H, Ouhibi C, Forges M, et al. Hormetic doses of UV-C light decrease the susceptibility of tomato plants to Botrytis cinerea infection. J Phytopathol. 2020;168:524–532.
- Forges M, Vàsquez H, Charles F, et al. Impact of UV-C radiation on the sensitivity of three strawberry plant cultivars (Fragaria × ananassa) against Botrytis cinerea. Sci Hortic. 2018;240:603–613.
- Urban L, Chabane Sari D, Orsal B, et al. UVC light and pulsed as alternatives to chemical and biological elicitors for stimulating plant natural defenses against fungal diseases. Sci Hortic. 2018;235:452–459.
- Hoagland DR, Arnon DI. The water culture method for growing plants without soil. Cal Agr Exp Stat Cir. 1950;347.
- Rebah F, Ouhibi C, Alamer KH, et al. Comparison of the responses to NaCl stress of three tomato introgression lines. Acta Biol Hung. 2018;69(4):464–480.
- Lichtenthaler HK. Applications of chlorophyll fluorescence. Dordrecht, Boston: Kluwer Acad Publ; 1988.
- Murray JR, Hackett WP. Leaf anthocyanin content changes in Zea mays L. grown at low temperature: significance for the relationship between the quantum yield of PSII and the apparent quantum yield of CO2 assimilation. Photosynthesis Res. 1998;58(3):213–219.
- R’him T, Tlili I, Hnan I, et al. Effet du stress salin sur le comportement physiologique et métabolique de trois variétés de piment (Capsicum annuum l.). J Appl Biosci. 2013;66:5060–5069.
- Bradford MM. A rapid and sensitive method for the quantitation of microgram quantities of protein utilizing the principle of protein-dye binding. Anal Biochem. 1976;72:248–254.
- Hodges DM, DeLong JM, Forney CF, et al. Improving the thiobarbituric acid-reactive-substances assay for estimating lipid peroxidation in plant tissues containing anthocyanin and other interfering compounds. Planta. 1999;207:604–611.
- Junglee S, Urban L, Sallanon H, et al. Optimized assay for hydrogen peroxide determination in plant tissue using potassium iodide. Am J Anal Chem. 2014;5:730–736.
- Cicco N, Lanorte M, Paragio M, et al. A reproductible rapid and inexpensive Folin-Ciocalteu micro- method in determining phenolics of plant methanol extracts. Microchem J. 2009;91:107–110.
- Sun J, Chu YF, Wu XZ, et al. Antioxidant and antiproliferative activities of common fruits. J Agric Food Chem. 2002;50(25):7449–7454.
- Pastor V, Balmer A, Gamir J, et al. Preparing to fight back: generation and storage of priming compounds. Front Plant Sci. 2014;5:295.
- de Oliveira IR, Crizel GR, Severo J, et al. Preharvest UV-C radiation influences physiological, biochemical, and transcriptional changes in strawberry cv. Camarosa. Plant Physiol Biochem. 2016;108:391–399.
- Hasan MM, Bae H. An overview of stress induced resveratrol synthesis in grapes: perspectives for resveratrol-enriched grape products. Molecules. 2017;22(2):294.
- Surjadinata BB, Jacobo-Velázquez DA, Cisneros-Zevallos LUVA. UVB and UVC light enhances the biosynthesis of phenolic antioxidants in fresh-cut carrot through a synergistic effect with wounding. Molecules. 2017;22(4):1–13.
- Charles MT, Benhamou N, Arul J. Physiological basis of UV-C induced resistance to Botrytis cinerea in tomato fruit: III. Ultrastructural modifications and their impact on fungal colonization. Postharvest Biol Technol. 2008;47:27–40.
- Charles MT, Tano K, Asselin A, et al. Physiological basis of UV-C induced resistance to Botrytis cinerea in tomato fruit. V. Constitutive defence enzymes and inducible pathogenesis-related proteins. Postharvest Biol Technol. 2009;51:414–424.
- Langer SE, Oviedo NC, Marina M, et al. Effects of heat treatment on enzyme activity and expression of key genes controlling cell wall remodeling in strawberry fruit. Plant Physiol Biochem. 2018;130:334–344.
- Umagiliyage AL, Choudhary R. Postharvest ultraviolet light treatment of fresh berries for improving quality and safety. Int J Food Sci Nutr Eng. 2018;8:60–71.
- Tiecher A, Paula LAD, Chaves FC, et al. UV-C effect on ethylene, polyamines and the regulation of tomato fruit ripening. Postharvest Biol Technol. 2013;86(4):230–239.
- Oztekin GB, Tuzel Y. Comparative salinity responses among tomato genotypes and rootstocks. Pak J Bot. 2011;6(43):2665–2672.
- Attia H. Physiological responses of pea plants to salinity and gibberellic acid. Phyton-Int J Exp Bot. 2022;92(1):149–164. DOI:10.32604/phyton.2022.022363.
- Aldesuquy HS, Abbas MA, Abohamed SA, et al. Glycine betaine and salicylic acid induced modification in productivity of two different cultivars of wheat grown under water stress. J Stress Physio Bioch. 2012;2(8):72–90.
- Xu X, Du X, Wang F, et al. Effects of potassium levels on plant growth, accumulation and distribution of carbon, and nitrate metabolism in apple dwarf rootstock seedlings. Front Plant Sci. 2020;11:904.
- Sankari M, Hridya H, Sneha P, et al. Effect of UV radiation and its implications on carotenoid pathway in Bixa orellana L. J Photochem Photobiol B. 2017;176:136–144.
- Rai R, Meena RP, Smita SS, et al. UV-B and UV-C pre-treatments induce physiological changes and artemisinin biosynthesis in Artemisia annua L. – an antimalarial plant. J Photochem Photobiol B. 2011;105(3):216–225.
- Sheng K, Zheng H, Shui SS, et al. Comparison of postharvest UV-B and UV-C treatments on table grape: changes in phenolic compounds and their transcription of biosynthetic genes during storage. Postharvest Biol Technol. 2018;138:74–81.
- Bravo S, García-Alonso J, Martín-Pozuelo G, et al. Effects of postharvest UV-C treatment on carotenoids and phenolic compounds of vine-ripe tomatoes. Int J Food Sci. 2013;48(8):1744–1749.
- Sahitya UL, Krishna MSR, Deepthi RS, et al. Seed antioxidants interplay with drought stress tolerance indices in Chilli (Capsicum annuum L) seedlings. BioMed Res Int. 2018;2018:1605096–1605096.
- Boccaccini A, Lorrai R, Ruta V, et al. The DAG1 transcription factor negatively regulates the seed-to-seedling transition in Arabidopsis acting on ABA and GA levels. BMC Plant Biol. 2016;16:198.