Abstract
This study evaluated the effect of different enzymatic hydrolysis and different microwave pretreatment durations on the sugar yield of Chinese spirits distillers residues (CSDR). Addition of both α-amylase and cellulase in the enzymatic hydrolysis of distillers grains resulted in a higher yield of reducing sugar (145.74 ± 4.20 mg·g–1). Fourier transform infrared spectroscopy (FTIR) revealed that different enzyme treatments degraded different functional groups of the cellulose structure. Subsequently, the highest reducing sugar yield (181.33 ± 6.42 mg·g–1) was achieved at microwave pretreatment time of 6 min and was 1.29-fold higher than that of the untreated sample. The differences in the structural features of CSDRs under various microwave pretreatment durations were characterized by scanning electron microscopy, FTIR spectroscopy, and X-ray diffraction. The data revealed that the optimal microwave pretreatment time at 6 min could obviously remove lignin, and then obtain the highest CrI value, achieving the highest reducing sugar yield.
1. Introduction
Biorefinery of lignocellulosic biomass has beenregarded as one of the most important environmentally sustainable alternatives to fossil resources [Citation1]. The composition of crystalline cellulose nanofibrils embedded into an amorphous matrix base on cross-linked lignin and hemicellulose with lignocellulose forming a natural recalcitrance that impedes enzyme and microbial accessibility, thereby having a relatively low conversion into carbohydrate-derived fermentable sugars constitutes [Citation2]. Many pretreatment methods have been developed for efficiently utilizing lignocellulosic raw materials enriching in cellulose and semi-fibrous components, which are classified into four categories [Citation3,Citation4]: (1) physical pretreatments (e.g. high energy radiation, grinding, milling, etc.), (2) chemical pretreatments (e.g. dilute acid or alkalis boiling, ozone oxidation, etc.), (3) physiochemical pretreatments (e.g. stem explosion, hydrothermal and organosolv methods [Citation1]) and (4) biological pretreatments.
Distillers grains are the main by-products in Baijiu production in China, with millions of tons produced annually during the Baijiu brewing process [Citation5]. Because of the high moisture content and high acidity of fresh Chinese spirits distillers residues (CSDR), they spoil easily under natural environmental conditions, resulting in loss of resources and environmental pollution. Therefore, many methods for their utilization have been explored, such as producing surfactin and fumaric acid as the fermented substrates [Citation6,Citation7], fermentation for energy [Citation8] and producing fermented feed [Citation9]. CSDRs are rich in cellulose and semi-fibrous components, with hemicellulose and lignin components forming a covalently bonded network structure that serves as an anti-degradation barrier from harvesting sugar, thereby limiting its biorefinery by microbiology in the fermented process [Citation10].
Recently, microwave irradiation, as one of the physical pretreatments, has been paid more attention to the research of pretreatment in various lignocellulosic materials and to their consequent property changes, thereby enabling to increase yield of reducing sugar after enzymatic hydrolysis (Table , [Citation3,Citation4,Citation10–14]). However, few studies have been involved in the pretreatment of CSDR so far. Only one report, by Ren et al., described that the fermented sugar yield from enzymatic saccharification of CSDR could be improved by a combination of microwave-assisted hydrothermal irradiation and fungal pretreatment [Citation10]. However, this study did not analyse property alterations of CSDR under different microwave pretreatment durations.
Table 1. Research summary of microwave pretreatment or enzymatic saccharification of biomass materials in the most recent five years.
In terms of the enzymatic hydrolysis process, implementation of effective enzymatic hydrolysis method(s) has been regarded as an effective way in enhancing the yield of reducing sugar (Table , [Citation15–18]). However, the effect of the saccharification based on different enzymatic hydrolysis methods on the sugar yield from CSDR together with the mechanism of its property changes by the treatments has not been evaluated in the previous reports.
In the present study, the effects of hydrolysis treatments with the differences in individual and sequential orders of enzymes on the sugar yield of CSDR were evaluated to determine the optimum conditions, and the enzymatic products were analysed by Fourier transform infrared spectrophotometry (FTIR). Subsequently, the CSDRs were subjected to different durations of microwave pretreatment to determine the optimum sugar yield under the optimum enzymatic hydrolysis conditions. The resulting properties of CSDR under different durations of microwave pretreatment were investigated by FTIR, X-ray diffraction (XRD) and scanning electron microscopy (SEM).
2. Materials and methods
2.1. Materials
The wet CSDR, coming from distillers residues of the fermented mixture with sorghum, rice, rice husk, wheat, glutinous rice and corn, were obtained from Anhui Gujing Tribute Wine Co., Ltd and refrigerated at 4°C. Prior to use, the wet CSDRs were dried at 80°C until a constant weight was reached. The composition of CSDR was determined according to the Van Soest method [Citation19] containing 28.15% of cellulose (dry weight mass ratio), 12.73% of lignin (dry weight mass ratio), 25.34% of hemicellulose (dry weight mass ratio), and 10.42% of the ash (dry weight mass ratio).
For the enzymatic hydrolysis treatment, cellulase (30 U·mg–1, Hefei Bomei Biotech Co., Ltd, China), xylanase (250 U·mg–1, source: bovine liver, Hefei Bomei Biotech Co., Ltd, China), pectinase (30 U·mg–1, Hefei Bomei Biotech Co., Ltd, China), hemicellulase (2 U·mg–1, source: bovine liver, Hefei Bomei Biotech Co., Ltd, China), α-amylase (30 U·mg–1, Hefei Bomei Biotech Co., Ltd, China), glycase (80 U·mg–1, source: bovine liver, Hefei Bomei Biotech Co., Ltd, China) and ammonium acetate (≥98.0 wt.%, Sinopharm, China) were used.
The following reagents were used in the analytical experiments: Phloroglucinol (99% purity by HPLC analysis), 3,5-dinitrosalicylic acid (≥98.0 wt.%, Sinopharm, China), sodium potassium tartaric acid (≥99.0 wt.%, Sinopharm, China), D(+)-Glucose (≥99.9 wt.%, Sinopharm, China), D-Xylose (≥99.0 wt.%, Sino-pharm, China), fuminutesg HCl 37% (w/w) with AR purity grade (Sinopharm, China), Glacial acetic acid (99.5 wt.% Sinopharm, China). The glucose kit was purchased from Changchun Huili Biotechnology Co., Ltd.
2.2. Methods
Enzyme treatment: Ten grams of the dried distillers grains were added to either cellulase (0.67 g), xylanase (0.04 g), pectinase (0.13 g), hemiculase (0.5 g), α-amylase (0.33 g), or glycase (0.125 g), based on previous publications [Citation10] and the preliminary experimentation. The individual enzymes and the order of addition for the enzymatic hydrolysis of CSDR are shown in Table . The dried distillers grains (10 g) were packed into 250 mL conical flasks. The first enzyme with ammonium acetate buffer (pH 5.0) was added and mixed, prior to culturing in a shaking incubator at 50°C for 3 h. Then, the 2nd enzyme with ammonium acetate buffer (pH 5.0) was added and mixed to be cultured again in a shaking incubator at 50°C for a further 3 h. Subsequently, the enzymes were inactivated by immersion of the conical flask in a boiling water bath for 10 min followed by a filtration through eight layers of gauze and the filtrate was centrifuged at 5010×g for 15 min. The supernatant was used to determine the sugar yield. The residues on the filter (Figure (A,B)) were dried in an oven at 80°C until a constant weight was obtained, prior to analysis.
Figure 1. (A,B) Different enzymolysis samples. “1–16” refers to the different enzymatic hydrolysis methods in Table ; (C) Samples following microwave pretreatment for different durations.
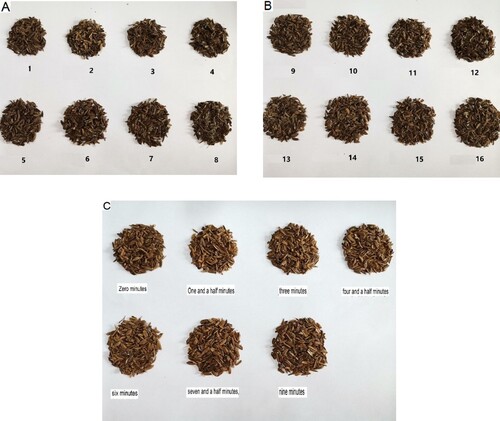
Table 2. Different enzymes or their order of addition forenzymatic hydrolysis of Chinese spirits distillers residues.
Microwave pretreatment: The dried distillers grains were treated in a Galanz microwave oven at 900 W·h–1 for six different durations: 1.5, 3, 4.5, 6, 7.5, and 9 min. The different durations of microwave pretreatment were determined by our pre-experimental results. These microwave pretreated samples (Figure (C)) were then hydrolysed using the optimum enzymatic hydrolysis conditions. The enzymes were inactivated thermically in a boiling water bath for 10 min and the reactant was then filtrated through eight layers of gauze. The filtrate was then centrifuged at 5010×g for 15 min. The supernatant was used to analyse the sugar yield. The residues on the filter were dried in an oven at 80°C until a constant weight was obtained, prior to further analysis.
2.3. Characterization methods
2.3.1. The sugar yield
The glucose content was assayed using the glucose testing kit, according to the manufacturer’s instruction and reducing sugar was assayed using the 3,5-dinitrosalicylic acid (DNS) method [Citation20]. Briefly, the mixture of the reaction system consisted of enzyme solution (1 mL), DNS (1.5 mL), and distilled water (1 mL) was heated in a boiling water bath for 5 min, then cooled in an ice bath. The optical density (OD) of the cooled reaction liquid was determined at 540 nm using a visible–ultraviolet spectrophotometer and the content of reducing sugar was calculated according to the glucose standard curve. The yield of reducing sugar in the enzymatic solution was then calculated.
The xylose content was analysed using the inter-phenol colour control method [Citation21]. Briefly, phloroglucinol (1 g) was added into acetic acid (200 mL) and HCl (37%, 12 mL) to form the chromogenic agent. A sample (25 μL) was mixed with chromogenic agent (25 mL) in a tube, and double-distilled water replaced the sample in the blank control. The mixed liquid was heated for 8 min in boiling water and then cooled to room temperature in an ice bath. The OD value of the mixed liquid was analysed at 553 nm using a visible–ultraviolet spectrophotometer and the xylose yield (mg·/g–1) was then calculated.
2.3.2. SEM analysis
Following microwave pretreatment, the dried distillers grains were ground into powder and sprinkled onto a conductive adhesive located on a sample seat. Unbound sample powder was blown away using an ear ball and the sample seat was placed into the scanning electron microscope for observation. Images were taken at different resolutions.
2.3.3. Infrared spectral analysis
The analytical method was referred from the literature [Citation22] with a minor modification. The dried residues on the filter or dried distillers grains were ground using a ceramic mortar. Subsequently, 1–2 mg of the grounded sample was further grounded using an agate mortar and the resulting powder was mixed with dried potassium bromide and pressed into a sheet. The characterization was performed according to our previous method [Citation23] using an infrared spectrometer (Bruker Ltd., Germany) in the absorbance mode within the range from 4000 to 650 cm–1. A total of 32 scans at a resolution of 1.93 cm–1 were recorded for both the background and the samples. All analyses were completed within 1 h to avoid potential inaccuracies.
2.3.4. X-ray diffraction
The pretreated and dried distillers grains were ground into a powder in a ceramic mortar. The sample powder was then evenly distributed on the sample rack and flattened on a glass plate prior to analysis by an X-ray diffractometer (D/max-2004). The scanning interval was 0.02° and the scanning range was 5–50°. The relative crystallinity index (CrI) was calculated according to the Segal formula [Citation24,Citation25].
2.4. Data analysis methods
Experiments were performed in triplicate and significant differences (P < 0.05) were evaluated using one-way analysis of variance (ANOVA) followed by Turkey’s HSD tests. All analyses were carried out using SPSS 13.0 for windows (SPSS Inc., Chicago, IL, USA).
3. Results and discussion
3.1. Effects of different enzymes or orders of addition on the fermentable sugar yield of distillers grains
Different enzymes or orders of addition were trialled in the enzymatic hydrolysis of CSDR. The sugar yield (glucose, reducing sugar and xylitol) was determined, with the results displayed in Figure . As shown in Figure (A), the highest yield of reducing sugar (145.74 ± 4.20 mg·g–1) was obtained in Experiment 9. The highest reducing sugar yield in the present study was slightly lower than that in the previous report (162.90 mg·g–1 from the brewers spent grain) [Citation26] and was far lower than that obtained from the reactions with the brewers spent grain (228.25 mg·g–1) [Citation27] and CSDR (255.1 mg·g–1) [Citation10]. These different results could be due to the different raw material sources. The highest xylose yield of 8.07 ± 0.38 mg·g–1 was obtained in Experiment 15, which was likely due to an addition of xylanase. The highest glucose yield (25.25 ± 2.51 mg·g–1) was obtained in Experiment 5.
Figure 2. Fermentable sugar yield from Baijiu distillers grains following treatment with different enzymes or different addition orders. (A) Reducing sugar; (B) Glucose; (C) Xylos. Different capital letters in columns indicate statistically significant differences (p < 0.05).
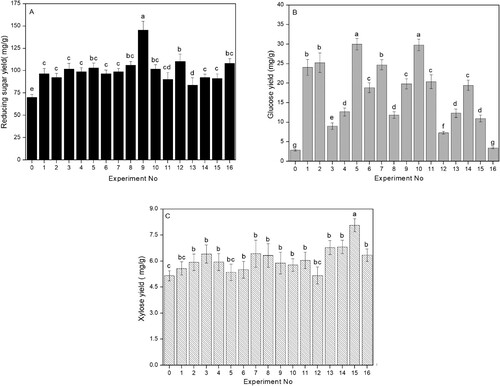
Reducing sugars, which mainly include glucose, fructose, galactose, lactose, and maltose, can be readily used by microorganisms in the fermentation process. Additionally, in the previous literature reports [Citation10,Citation27], the content of reducing sugar has been regarded as the key index for evaluating the enzymatic effect on saccharification. Therefore, the reducing sugar yield was chosen as the key index for evaluating results in the present study.
Experiment 9 involved the addition of both cellulase and α-amylase for enzymatic hydrolysis, resulting in the highest reducing sugar yield compared to those of other enzymatic treatments (Figure (A), P < 0.05). The yields of sugar-glucose, reducing sugar, and xylitol were 19.85, 145.74 and 5.89 mg·g–1, respectively. Significantly, our results provided a pioneer report on enhancing the sugar yield of CSDR by screening enzymes.
In the enzymatic hydrolysis treatment of the brewers spent grain, it was found that an addition of the mono-component of cellulases (EG I and CBH I) resulted in a less release of sugar than that obtained with Celluclast, indicating that the additional enzymes present in this cellulase preparation were required to improve the solubilization of brewers spent grain [Citation28]. Thus, the results of the present study were somewhat similar to those previously reported [Citation28]. Additionally, the characteristics of the residue of enzymatic hydrolysis were not significantly different (Figure (A,B)).
3.2. Effects of microwave pretreatment for different durations on the sugar yield of distillers grains
Considerable efforts have been devoted to the study of biomass pretreatment by microwave irradiation for improving bioconversion efficiency, with the duration of microwave pretreatment regarded as a very important factor [Citation10,Citation29]. In the present study, the dried distillers grain samples were pretreated under different durations in a microwave oven. Subsequently, the samples were treated under the optimum enzymatic hydrolysis conditions. The sugar yield of the resulting samples was investigated. As shown in Figure , the yield of reducing sugar was initially increased and then decreased as the microwave duration increased. After microwave pretreatment for 4.5 and 6 min, the reducing sugar yield (166.79 ± 8.4 and 181.33 ± 6.42 mg·g–1, respectively) increased to 18.5% and 29% respectively compared to the untreated sample (140.73 ±7.28mg·g–1; P < 0.05). The microwave pretreated CSDR yielded a reducing sugar content of 181.33 ±6.42mg·g–1 which was 2.58-fold higher compared to that of the native CSDR (Table (A), 70.28 ± 3.31 mg·g–1; P < 0.05). In previous related research, a 2.86-fold enhancement in total reducing sugar yield (228.25 mg·g–1) could be obtained through the microwave assisted and alkali pretreated method after enzymatic hydrolysis compared to the native brewer’s spent grain (79.67mg·g–1). Thus, our work presented here could become a promising method for applications of downstream fermentation. The xylitol and glucose yield following microwave pretreatment for 1.5 and 6 min was slightly increased compared to the untreated sample (P > 0.05). Considering the reducing sugar yield, an optimal microwave pretreatment time of 6 min was selected.
Figure 3. Fermentable sugar yield from distillers grains following different microwave pretreatment durations. Different capital letters in columns indicate statistically significant differences (p < 0.05).
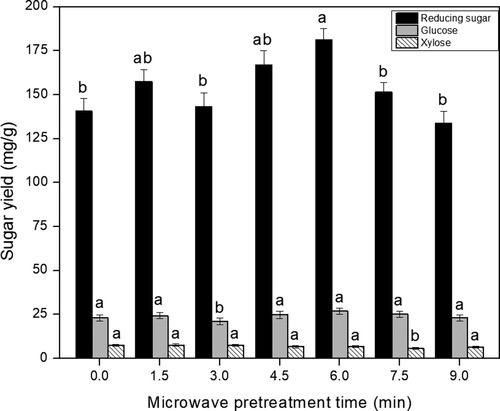
The colour of the distillers grains treated bymicrowave for 7.5 and 9 min was slightly darker than that of the samples subjected to shorter microwave pretreatment durations (Figure (C)). This may have been due to the carbonization of distillers grains.
3.3. SEM analysis of microwave pretreatment for different durations
During microwave pretreatment, different processes may occur that can affect the content of chemical components. Alteration of microstructure of the raw materials, therefore, can affect the efficiency of enzymatic hydrolysis. SEM is an important method that is commonly used to observe the surface structure or morphology of lignocellulose [Citation14,Citation22].
In the present study, the SEM images of CSDR following different microwave pretreatment durations were analysed, with the results shown in Figure . Figure (A) reveals that the surface of the untreated raw material had a compact particulate state. After microwave pretreatment for 1.5 (Figure (B)) and 3 min (Figure (C)), the compact particular structure gradually becomes disintegrated. Extension of the microwave pretreatment time to 4.5 min (Figure (D)) resulted in a complete loss of the particulate structures to form multiple clear gully structures. After 6 min of pretreatment (Figure (E)), the gully structures were damaged, and after 7.5 min (Figure (F)) the gully structures disappeared and the surface became blurred. The structural alteration was due to carbonization (Figure (C)). After pretreatment for 9 min (Figure (G)), the surface became flaked and fractured. These results showed that microwave pretreatment destroyed the apparent structure of distillers grains, with longer treatment duration resulting in more serious alteration. The disruption of grain stillage structures and the presence of porosity may increase the accessibility of enzyme molecules to the degradable strictures of grain stillage and improve the subsequent enzymatic hydrolysis.
3.4. The comparison and analysis of FTIR spectra
Recently, FTIR spectral analysis has been performed to compare the functional groups of various biomass raw materials subjected to different pretreatment methods [Citation10,Citation12,Citation14]. In the present study, 17 infrared spectra of CSDR following different enzymatic hydrolysis treatments were analysed with the results shown in Figure . Spectra were interpreted by referencing to previous literature [Citation10,Citation12].
Figure 5. FTIR spectra of Chinese spirits distillers residues following different enzymatic hydrolysis (A) and microwave pretreatment durations (B). A: “0” refers to the Chinese spirits distillers residues without enzymatic hydrolysis; “1–16” refers to the different enzymatic hydrolysis methods in Table ; “0–6” refers to Chinese spirits distillers residues following microwave pretreatment for different durations of 0 min (0), 1.5 min (1), 3 min (2), 4.5 min (3), 6 min (4), 7.5 min (5) and 9 min (6).
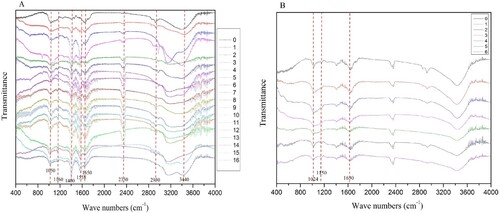
The main absorbances in the samples were broad peaks around 3440, 2930, 2350, 1650, 1558, 1400, 1160 and 1050 cm–1 (Figure (A)). The broad band at 3436 cm−1 was related to the stretching vibrations of H-bonded –OH groups in the cellulose structure [Citation10]. The sharp absorption peak at 2930 cm−1 was attributed to C–H stretching vibrations of lignin [Citation10,Citation12]. The absorption peak at 1650 cm−1 was due to the vibration of carbonyl and carboxyl C–O bonds in lignin [Citation10,Citation12]. The absorption peak at 1558 cm−1 was attributed to aromatic skeletal vibration or C=O stretching of lignin [Citation12]. The absorption peak at 1400 cm−1 corresponded to the twisting vibrations of C–H bonds within the cellulosic components [Citation12]. The peak at 1050 cm−1 corresponded to vibrations of the pyranose ring (C–O–C) structures of lignin, while the band at 1150 cm–1 was due to asymmetric elongation of C–O–C bonds within cellulose [Citation10].
In comparison of the absorbances of samples following different enzymatic hydrolysis treatments to the untreated sample, the peaks at around 3440 cm–1 were only found in some of the samples that were subjected to enzymatic hydrolysis (Experiment 0, 1, 2, 3, 7, 15, and 16) (Figure (A)). This was because the other enzymatic hydrolysis treatments destroyed the cellulose structure.
The absorbances at about 3200, 2360, and 2930 cm–1 were not present in the samples resulting from Experiments 0, 1, 3, 4 and 5; Experiments 14 and 15; and Experiments 2, 7, and 8, respectively (Figure (A)). The absorbances at about 1560 in a non-enzymatic sample and 1160 cm–1 in an enzymatic sample (Experiments 1–4) were not present (Figure (A)). These results indicated that some enzymatic hydrolysis treatments destroyed the structure of cellulose, such as cellulase degraded cellulose, resulting in lignin exposure.
The samples following different durations ofmicrowave pretreatment were analysed by FTIR spectroscopy (Figure (B)). The absorbances at about1150 cm–1 disappeared in the samples that had been subjected to microwave pretreatment for 4.5 and 6 min, while the absorbances at about 1630 and 1024 cm–1 decreased in intensity in all samples that had been subjected to microwave pretreatment for more than 4.5 min. These results suggested, as expected, that some of the lignin structure was destroyed by a suitable microwave pretreatment duration.
3.5. XRD analysis of CSDR following different durations of microwave pretreatment
The XRD patterns of CSDR following microwave pretreatment for different durations are shown in Figure . The results revealed that the typical diffraction peaks at 2θ of about 16–18°, 20–22°, and 26–28°, which corresponded to cellulose I crystals, did not change following microwave pretreatment, indicating that the crystal form was unchanged.
Figure 6. XDR results of Chinese spirits distillers residues following different microwave pretreatment durations: 0 min (0), 1.5 min (1), 3 min (2), 4.5 min (3), 6 min (4), 7.5 min (5) and 9 min (6).
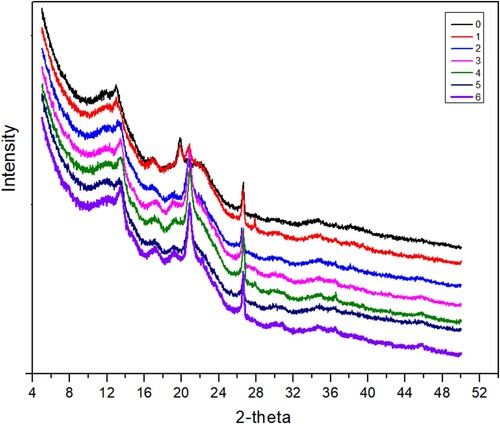
The inherent components in lignocellulosic biomass can be crystalline or amorphous in nature [Citation26]. Crystallinity in any plant biomass is dependent on cellulose [Citation26], while hemicellulose and lignin are amorphous [Citation26]. The crystallinity index (CrI) expresses the ratio of crystalline cellulose to the amorphous region, often being lignin and hemicellulose. The CrI values of CSDR following microwave pretreatment for 3, 4.5, 6, 7.5 and 9 min were higher than for the untreated distillers grains (Table , whereas the CrI values of CSDR following microwave pretreatment for 1.5 min were lower than that of the untreated distillers grains (Table ). The increased CrI values might imply that there was more damage to the amorphous lignin area than to the cellulose molecules as the exposure proportion of the crystallization area increased. In contrast, the lower CrI value indicated the opposite.
Table 3. Relative crystallization index of microwave pretreatments for different times.
Overall, the results demonstrated that microwave treatment could destroy lignin. Microwave treatments (4.5 and 6 min) could achieve higher CrI values (26.32% and 25.14%). It has been proposed that an increase in crystallinity may facilitate the formation of levoglucosan [Citation3]. Therefore, an increased yield of reducing sugar maybe attribute to a higher crystallinity (Figure ). However, XRD analysis indicated that the higher CrI value (25.14%) of microwave treatment at 6 min (Table ) yielded a lower reducing sugar containment (Figure ). This might be attributed to carbonization of sugar (Figure (C)) and a lower hydrolysis rate of biomass due to an overhigh CrI value [Citation14].
4. Conclusions
This study optimized the enzymatic and microwave pretreatments for maximizing sugar yield from CSDR and investigated the corresponding structural alterations under various conditions. Of 17 enzymatic hydrolysis methods, the optimum methods treated with both cellulase and α-amylase achieved a 2.07-fold higher yield in reducing sugar contain from CSDR. FTIR spectroscopy revealed that the enzyme treatments could differentially degrade cellulose functional groups.
Under the optimum enzymatic hydrolysis conditions, the best microwave processing time for the highest reducing sugar yield (1.29-fold higher than for the untreated sample) was 6 min. SEM indicated that the surface structure of CSDR was more seriously damaged with longer treatment times, FTIR revealed that microwave treatment could destroy lignin, and X-ray diffraction analysis showed that suitable processing times enhanced crystallinity of the structure.
Our results identified the optimal enzymatic hydrolysis method and microwave treatment duration for enhancing a maximal yield of reducing sugar from CSDR, and contributed the fundamental mechanism at the structural and molecule levels. This work, hopefully, could be helpful in future research on enzymatic hydrolysis and microwave treatment of CSDR and its application to biorefinery process technology.
Disclosure statement
No potential conflict of interest was reported by the author(s).
Correction Statement
This article has been corrected with minor changes. These changes do not impact the academic content of the article.
Additional information
Funding
References
- Morais A-R-C, Pinto J-V, Nunes D, et al. Imidazole: prospect solvent for lignocellulosic biomass fractionation and delignification. ACS Sustain Chem Eng. 2016;4(3):1643–1652. doi:10.1021/acssuschemeng.5b01600.
- Bernardo JR, Gírio FM, Łukasik RM. The effect of the chemical character of ionic liquids on biomass pre-treatment and posterior enzymatic hydrolysis. Molecules. 2019;24(4):808. doi:10.3390/molecules24040808.
- Jiang LQ, Wu YX, Wu NN, et al. Selective saccharification of microwave-assisted glycerol pretreated corncobs via fast pyrolysis and enzymatic hydrolysis. Fuel. 2020;265:116965. doi:10.1016/j.fuel.2019;116965.
- Silveira MHL, Morais ARC, da Costa Lopes AM, et al. Current pretreatment technologies for the development of cellulosic ethanol and biorefineries. Chem Sus Chem. 2015;8(20):3366–3390. doi:10.1002/cssc.201500282.
- He F, Yang H, Zeng L, et al. Production and characterization of bacterial cellulose obtained by Gluconacetobacter xylinus utilizing the by-products from Baijiu production. Bioproc Biosyst Eng. 2020;43(5):927–936. doi:10.1007/s00449-020-02289-6.
- Liu H, Yue X, Jin Y, et al. Preparation of hydrolytic liquid from dried distillers grains with solubles and fumaric acid fermentation by Rhizopus arrhizus RH 7–13. J Environ Manage. 2017;201:172–176. doi:10.1016/j.jenvman.2017.05.054.
- Zhi Y, Wu Q, Xu Y. Production of surfactin from waste distillersgrains by co-culture fermentation of two Bacillus amyloliquefaciens strains. Bioresour technol. 2017;235:96–103. doi:10.1016/j.biortech.2017.03.090.
- Ao T, Li R, Chen Y, et al. Anaerobic thermophilic digestion of maotai-flavored distillers grains: process performance and microbial community dynamics. Energ Fuel. 2019;33(9):8804–8811. doi:10.1021/acs.energyfuels.9b02582.
- Huang RL, Yin YL, Wang KP, et al. Nutritional value of fermented and not fermented material of distillers grains in pig nutrition. J Anim Feed Sci. 2003;12(2):261–270. doi:10.22358/jafs/67702/2003.
- Ren H, Sun W, Wang Z, et al. Enhancing the enzymatic saccharification of grain stillage by combining microwave-assisted hydrothermal irradiation and fungal pretreatment. ACS Omega. 2020;5(22):12603–12614. doi:10.1021/acsomega.9b03681.
- Aminutesi N, Haritos V-S, Tanksale A. Microwave assisted pretreatment of eucalyptus sawdust enhances enzymatic saccharification and maximizes fermentable sugar yield. Renew Energy. 2018;127:653–660. doi:10.1016/j.renene.2018.05.001.
- Anita S-H, Solihat N-N, Sari F-P, et al. Optimization of microwave-assisted oxalic acid pretreatment of oil palm empty fruit bunch for production of fermentable sugars. Waste Biomass Valor. 2020;11(6):2673–2687. doi:10.1007/s12649-018-00566-w.
- Moodley P, Kana EG. Microwave-assisted inorganic salt pretreatment of sugarcane leaf waste: effect on physiochemical structure and enzymatic saccharification. Bioresour Technol. 2017;235:35–42. doi:10.1016/j.biortech.2017.03.031.
- Rajeswari G, Arutselvy B, Jacob S. Delignification of aloe vera rind by mild acid associated microwave pretreatment to persuade enhanced enzymatic saccharification. Waste Biomass Valorization. 2020;11(11):5965–5975.doi:10.1007/s12649-019-00830-7.
- Silva T-P, Ferreira AN, de Albuquerque FS, et al. Box–Behnken experimental design for the optimization of enzymatic saccharification of wheat bran. Biomass Convers Biorefinery. 2021:1–8. doi:10.1007/s13399-021-01378-0.
- Romero-Borbón E, Oropeza-González AE, González-García Y, et al. Thermochemical and enzymatic saccharification of water hyacinth biomass into fermentable fugars. Processes. 2022;10(2):210. doi:10.3390/pr1002-0210.
- Alias NH, Abd-Aziz S, Yee Phang L, et al. Enzymatic saccharification with sequential-substrate feeding and sequential-enzymes loading to enhance fermentable sugar production from sago hampas. Processes. 2021;9(3):535. doi:10.3390/pr9030535.
- Mukasekuru MR, Kaneza P, Sun H, et al. Fed-batch high-solids enzymatic saccharification of lignocellulosic substrates with a combination of additives and accessory enzymes. Ind Crop Prod. 2020;146:112156. doi:10.1016/j.indcrop.2020.112156.
- Van Soest PV, Robertson JB, Lewis BA. Methods for dietary fiber, neutral detergent fiber, and nonstarch polysaccharides in relation to animal nutrition. J Dairy Sci. 1991;74(10):3583–3597. doi:10.3168/jds.S0022-0302(91)78551-2.
- Miller GL. Use of dinitrosalicylic acid reagent for determinutesation of reducing sugar. Anal Chem. 1959;31(3):426–428. doi:10.1021/ac60147a030.
- Xu L, Liu L, Li S, et al. Xylitol production by Candida tropicalis 31949 from sugarcane bagasse hydrolysate. Sugar Tech. 2019;21(2):341–347. doi:10.1007/s12355-018-0650-y.
- Alam A, Zhang R, Liu P, et al. A finalized determinutesant for complete lignocellulose enzymatic saccharification potential to maximize bioethanol production in bioenergy Miscanthus. Biotechnol Biofuels. 2019;12(1):1–22. doi:10.1186/s13068-019-1437-4.
- Zeng H, Shuai Y, Zeng X, et al. Evaluation of health-related composition and bioactivity of five fruit juices following Lactobacillus plantarum fermentation and simulated digestion. Int J Food Sci Tech. 2021;56(2):648–660. doi:10.1111/ijfs.14713.
- Wu XQ, Liu PD, Liu Q, et al. Production of cellulose nanofibrils and films from elephant grass using deep eutectic solvents and a solid acid catalyst. RSC Adv. 2021;11(23):14071–14078. doi:10.1039/d1ra02259h.
- Li F, Xie G, Huang J, et al. Os CESA 9 conserved-site mutation leads to largely enhanced plant lodging resistance and biomass enzymatic saccharification by reducing cellulose DP and crystallinity in rice. Plant Biotechnol J. 2017;15(9):1093–1104. doi:10.1111/pbi.12700.
- Rr A, Cs A, Sj B, et al. Improving enzymatic hydrolysis of brewer spent grain with nonthermal plasma. Bioresour Technol. 2019;282:520–524. doi:10.1016/j.biortech.2019.03.071.
- Ravindran R, Jaiswal S, Abu-Ghannam N, et al. A comparative analysis of pretreatment strategies on the properties and hydrolysis of brewers, spent grain. Bioresour Technol. 2018;248:272–279. doi:10.1016/j.biortech.2017.06.039.
- Niemi P, Faulds CB, Sibakov J, et al. Effect of a milling pre-treatment on the enzymatic hydrolysis of carbohydrates in Brewer's spent grain. Bioresour Technol. 2012;116:155–160. doi:10.1016/j.biortech.2012.04.043.
- Dawid M, Grzegorz K. Microwave-assisted hydrotropic pretreatment as a new and highly efficient way to cellulosic ethanol production from maize distillery stillage. Appl Microbiol Biotechnol. 2021;105(8):3381–3392. doi:10.21203/rs.3.rs-36466/v1.