Abstract
Cardiac dysfunction can be prevented by addressing risk factors including unhealthy diet with other lifestyle modifications. The present study demonstrates the effect of diet mixed with sea and table salts on cardiac remodeling with respect to CKMB, renin, angiotensin II, interleukin-6 and 10. Animals divided into six groups. Normal control, Table salt (0.3%), Natural sea salt (0.3%), Doxorubicin-induced cardiac remodeling control (2 mg/kg), + Normal table salt (0.3%) and Doxorubicin + Natural sea salt (0.3%) . Serum analysis and histopathology of heart, liver and kidney was performed. Significant variation (P < .05) observed in in CKMB for Natural sea salt group compared to Normal. Significant (P < .05) variation in renin for Normal table salt group compared to Normal. Variation in interleukin-6, 10, renin and angiotensin II give new insight in these parameters with marked variation in cellular architecture of the heart, liver and kidney. Observations suggest diet with natural sea salt demonstrated significant beneficial effect on cardiac dysfunction.
1. Introduction
Cardiovascular diseases (CVDs) are the number one cause of death globally with an estimated 19.9 million deaths each year [Citation1]. Among the established predictors of CVD deaths, hypertension plays an important role and is a major contributor among all other factors [Citation2]. Hypertensive patients are often at risk of ventricular dilation, infarct thinning and extensive formation of collagen tissue in the damaged zone of the heart muscle [Citation3]. Subsequently, the shape of the ventricular chamber distorts in the non-infarct area and eccentric hypertrophy is developed. All these morphological variations in cardiac tissues are termed cardiac dysfunction [Citation4] leading to congestive cardiac failure followed by cardiac rupture [Citation5]. The suppression of early ventricular infarct expansion and reversion of cardiac dysfunction are the need of the hour and can be achieved by drugs and adjuvant pharmacological therapies [Citation6]. These drugs are not free from adverse effects and can lose their efficacy in due course of time [Citation7]. Hence immense interest is developed among researchers to look for a therapy that can minimize the dysfunction of cardiac tissue a significant cause of morbidity and mortality among the global population.
Cardiovascular morbidity and mortality are extensively and directly linked to the intake of excessive salt in diet [Citation8]. Clinical and experimental studies over the last decade have shown that an increase in the consumption or intake of salt, is concomitant with injurious and deleterious effects including endothelial malfunction [Citation9], increase blood pressure or hypertension [Citation10], cardiac or brain stroke [Citation11], heart failure [Citation12], and kidney ailment or diseases [Citation13]. In conclusion, high salt consumption is a powerful risk factor for all CVDs. Records of most population-based studies establish that salt consumption currently exceeds the normal limits prescribed by regulatory agencies [Citation14]. Reduction in salt consumption is advocated by regulatory agencies to reduce the incidence of CVDs the most common cause of death on the global platform. However, there is a growing concern among researchers over the recommendation of salt intake by the general population [Citation15]. The actual factor associated with this concern or low salt intake in the diet may activate the sympathetic nervous system along with the renin-angiotensin system (RAS). The two important factors play a major role in the long-term governance of cardiac function and its dysfunction in CVDs patients especially in the post-infarct period [Citation16]. Hence, low-salt diets could play a potentially harmful role in patients with hypertension or infarction paving the way to cardiac hypertrophy and cardiac fibrosis leading to the development of congestive cardiac failure.
It is important to note that apart from a standard pharmacological treatment, a reduction in salt intake is the first line of action after any acute CVDs including myocardial infarction. Based on facts of high salt intake, it is expected to attenuate left ventricular dysfunction through the reduction of salt intake that can prevent heart failure in CVD patients. Contrary to this hypothesis several researchers are not of the opinion that reducing salt intake can lower heart failure in such patients [Citation17] instead it was observed that hospitalization time was increased for cardiovascular alignment with increased mortality rate [Citation18], with detrimental renal and neurohormonal effects [Citation19]. This fact and knowledge of high salt consumption or in change, the salt chemical composition would reduce cardiac dysfunction, thus addressing a larger issue of heart failure.
Accordingly, as on date considering all factors, the objective of the present study was to validate the designed hypothesis on the biochemical, pharmacological and histopathological character of natural sea salt against table salt by the doxorubicin-induced cardiac dysfunction, via the regulation of the RAS system, proinflammatory and anti-inflammatory cytokines along with architectural variation in the heart, liver and kidney tissues.
2. Materials and methods
2.1. Experimental animals and groups
Male albino rats (n = 36; weight = 140–180 g) were obtained from King Fahad Medical Research Centre (KSA, Jeddah). Animals were acclimatized for seven days to standard laboratory conditions before the commencement of protocol at the animal house facility of the Biochemistry Department, Faculty of Science, King Abdulaziz University at 21 ± 1°C temperature, with relative humidity (60 ± 10%) on a 12-hour light/dark cycle with free access to food and water. The protocol was designed as per the King Abdulaziz University Research Ethics of Scientific Research at King Abdulaziz City for Science and Technology (Reference number 520-20).
Group I was designated as Normal control (N.C.), Group II was Table salt (0.3%) (N.T.S.) treated, Group III served as Natural sea Salt fed (0.3%) (N.S.S.), Group IV animals exposed to Doxorubicin (2 mg/kg) and served as Doxorubicin controls (D. C.), Group V animals served as D. C. + Table salt (0.3%) (D.T.S.) treated, while Group VI animals were D. C + Natural sea Salt (0.3%) (D.S.S.)
2.2. Doxorubicin dose, food, water, salt administration, kits and instruments
Doxorubicin (DOX) is an anthracycline anticancer or antineoplastic drug prescribed in the treatment of solid tumours and haematologic malignancies. Despite its anticancer benefits, clinically it is associated with acute and chronic cardiotoxicity. The toxicity is dose-dependent, cumulative and essentially irreversible [Citation20,Citation21]. DOX i.p at a dose of 2 mg/kg [Citation21] was administered in cardiac disease group rats in beginning and bottled water was administered in all group rats. The rats’ diet was mixed with water and salt by the following ratio: 1 kg of food: 500 ml of water: 3 g of salt [Citation22]. Table salt (Sasa) is a market product of Saudi salt refinery work. Jeddah. Saudi Arabia. Sea salt (Al Hayat). Jeddah Saudi Arabia (100% Natural Product). Generic vial of commercially available DOXORUBICIN 50 MG/25 ML VIAL from Pfizer, procured by Batterjee Medical. Jeddah. Rat Creatine Kinase MB Isozyme (CK MB) ELISA kit (Cat. No.: MBS705376; MyBioSource, San Diego, CA, USA), Rat Interleukin 6 (IL-6) Elisa kit (Competitive ELISA) (Cat. No.: MBS726707; MyBioSource, San Diego, CA, USA), Rat Angiotensin II (ANG-II) ELISA Kit (Cat. No.: CSB-E04494r; CUSABIO, Houston, TX, USA), Rat Renin (RENIN) ELISA kit (Cat. No.: MBS041519; MyBioSource, San Diego, CA, USA), Rat IL-10 ELISA Kit (Cat. No.: MBS355232; MyBioSource, San Diego, CA, USA) were used as source causative and therapeutic drug materials. Along with an automated microplate immunoanalyser from DSX best 2000. Microplate Spectrophotometer-BioTek instruments from Epoch™ 2 as important instruments for the detection of results in our protocol.
2.3. Determination of biochemical parameters
All the experimental animals were kept for overnight fasting before sacrificing under light anaesthesia of phenobarbital. A blood sample was drawn via the retro-orbital puncture method as per the given standards of the ethical committee. A blood sample was collected in anticoagulant/without anticoagulant tubes, centrifuge at 4500 rpm and plasma/serum was stored at 4°C. The samples were examined for the biochemical parameters including CKMB, Renin, Ang II, IL-6, and IL-10 by kits through enzymatic determination methods on an automated analyzer (Dimension-Clinical Chemistry System, USA). All the tests were performed through a kit-based assay technique as per the instructions of the manual [Citation23,Citation24].
2.4. Histopathology
Samples from the heart, liver and kidney, 2–3 mm were sliced and fixed in neutral buffered formalin (10% formaldehyde in phosphate-buffered saline v/v) overnight. After fixation, tissues were dehydrated in an ascending gradient of ethanol for one hour in each step (from 70%, 80%, 95%, 100%, 100% and 100%), after dehydration, tissues were infiltrated by xylene twice for one hour in each step. Finally, tissues were infiltrated by paraffin at 65°C twice, one hour for each step. Fixed tissues were placed in a stainless-steel mould and molten paraffin was poured into the mould after 5 s the cassata was placed on top of the mould. The mould with cassata was placed on the freezing table. After 1 h, the blocks were solidified and they were sectioned in microtome into 5-µm sections. Tissue sections were mounted on the slides and kept in a warm oven for 2 h at 60°C. Slides containing paraffin sections were placed on a slide holder. Slides were deparaffinized with xylene for 10 min. Then xylene was removed by absolute ethanol. Slides were rehydrated with 100%, 90% and 80% alcohol for 2–3 min in each, and put into the water for 3 min. Excess water on slides was blotted and slides were put into a haematoxylin stain for 5 min. Slides were removed from the haematoxylin stain and put into tap water to remove extra haematoxylin for 1–2 min. Slides were immersed into the Eosin stain for 30 s. After that, slides were dehydrated with 80%, 90%, 100% alcohol and finally with xylene for 20 min. A coverslip was placed on the slide using one drop of DPX, and the slides were dried overnight to make the permanent slide [Citation25]. The images were captured by comprehensive digital pathology solutions using IntelliSite Pathology Solution from Philips model number (FMT0225) at Al Borg Medical Laboratories.
2.5. Statistical analysis
The analysis was performed as per the standard protocol with the expression of data in mean ± SEM. GraphPad Prism 8 (Graph Pad Software, San Diego, CA, USA) was used for one-–way analysis of variance (ANOVA) to compare the difference. This was followed by Tukey multiple comparison tests used to compare the difference between the group that is normal table salt group (0.3%) (N.T.S.) and natural sea salt group (0.3%) (N.S.S.) versus normal control (N.C.) and doxorubicin-induced cardiac dysfunction table salt (0.3%) (D.T.S.) and doxorubicin-induced cardiac dysfunction natural sea salt (0.3%) (D.S.S.) versus doxorubicin-induced cardiac dysfunction (2 mg/kg) (D. C.). P-value was considered to be significant in the given order <.05(*), <.01(**) and <.001(***).
3. Results
The level of CKMB, Renin, Ang II, IL-6, and IL-10 in both normal and doxorubicin-induced cardiac dysfunction models of groups are represented in Figure and Table . Mean ± SEM for particular group and P-values of significance in variance analysis are depicted in parenthesis wherever applicable.
Figure 1. Effect of table salt and natural sea salt in different groups: the normal control (N.C.) group; the normal table salt (N.T.S.) group; the normal sea salt (N.S.S.) group; the doxorubicin-induced cardiac dysfunction control (D.C.) group; the ddoxorubicin-induced cardiac dysfunction control + table salt (D.T.S.) group; the ddoxorubicin-induced cardiac dysfunction control + sea salt (D.S.S.) group on Renin, Ang II, CKMB, IL-6 and IL-10 levels. N.T.S and N.S.S. groups are compared to the N.C. group, D.T.S and D.S.S. groups are compared to D.C., and the D.C group is compared to the N.C. group; values are mean ± SEM; n = 6; <.05(*), <0.01(**) and <.001(***).
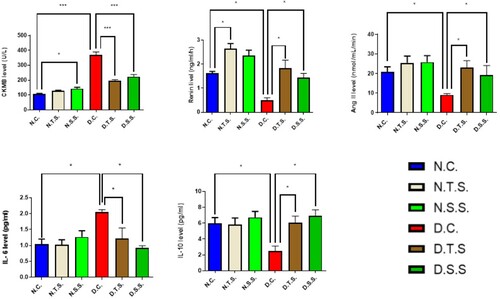
Table 1. Comparative analysis of salts with/without disease in terms of mean and SEM for creatine kinase myocardial band (CKMB), renin, angiotensin II (Ang II), interleukin-6 (IL-6) and interleukin-10 (IL-10).
3.1. Creatine kinase myocardial band
CKMB exhibited increased value in N.T.S. and significant one (P < .05) for N.S.S. compared to N.C. (126.7 ± 5.04), (143 ± 9.29) and (107.5 ± 3.50), respectively. In the doxorubicin-induced cardiac dysfunction groups, the levels of CKMB for both D.T.S and D.S.S. decreased significantly (P < .001) when compared to the D.C. group (195.7 ± 8.45), (223.7 ± 14.50) and (368.7 ± 20.54), respectively. The D.C. group showed a high increase (P < .001) compared to the N.C. group (Figure ).
3.2. Renin
The N.T.S. (2.65 ± 0.20) group exhibited a significant variation, i.e., increase (P < .05) in renin value compared to the N.C. group (1.63 ± 0.07 whereas the N.S.S. group 2.36 ± 0.23) exhibited normal values compared to the N.C. group. While in the case of doxorubicin-induced cardiac dysfunction groups, both D.T.S. (1.83 ± 0.34) and D.S.S. (1.44 ± 0.18) exhibited a significant increase (P < .05) in the levels of rennin compared to the D.C. group (0.51 ± 0.10). In contrast, the D.C. group decreased significantly (P < .05) compared to the N.C. group (Figure ).
3.3. Angiotensin II
There are normal values for both N.T.S. and N.S.S (25.47 ± 3.53) and (25.87 ± 3.27), respectively, compared to N.C. (20.75 ± 2.75). Whereas, in protocol II, both D.T.S and D.S.S. have a significant increased (P < .05) in the levels of Ang II compared to the D.C. group (23.17 ± 3.49); (19.37 ± 4.80); (9.133 ± 0.63), respectively. On the other hand, the D.C. group exhibited a significant decrease (P < .05) compared to the N.C. group (Figure ).
3.4. Interleukin-6
The level of IL – 6 in protocol I did not have significant values in both the N.T.S. (1.05 ± 0.16) and the N.S.S. group (1.03 ± 0.15) compared to the N.C. group (1.05 ± 0.16). In the doxorubicin-induced cardiac dysfunction groups, both the D.T.S. group (1.22 ± 0.33) and D.S.S. group (0.92 ± 0.07) have decreased significant values (P < .05) compared to the D.C. group (2.05 ± 0.08) (Figure ).
3.5. Interleukin-10
The level of IL – 10 in the normal groups did not have significant values in both the N.T.S (5.83 ± 0.83) and N.S.S group (6.73 ± 0.75) compared to the N.C. group (6 ± 0.70). In the doxorubicin-induced cardiac dysfunction groups, both the D.T.S. group (6.03 ± 0.86) and D.S.S. group (6.93 ± 0.74) have increased significant values (P < .05) compared to the D.C. group (2.47 ± 0.66) towards the normal control group (Figure ).
3.6. Histopathology
Sections were evaluated for pathological changes in the heart, liver and kidney (Figures , and , respectively).
Figure 2. Heart histopathology of random images in different groups: (A) the Normal control (N.C.) group; (B) the Normal table salt (N.T.S.) group; (C) the Normal sea salt (N.S.S.) group; (D) the Doxorubicin-induced cardiac dysfunction control (D.C.) group; (E) the Doxorubicin-induced cardiac dysfunction control + Table salt (D.T.S.) group; (F) the Doxorubicin-induced cardiac dysfunction control + Sea salt (D.S.S.) group. The figures define the following characteristics: loose cardiac myocyte (yellow arrow), fat deposition (grey arrow), fibrinogen deposition (green arrow), eosinophilic infiltration (black arrow), ventricular cells arrangement (blue arrow) and intracellular gaps (red arrow). Original magnification – 40×; Scale = 50 µm.
3.6.1. Heart
The morphological changes of the heart were recorded for loose cardiac myocytes, fat deposition, fibrinogen deposition, eosinophilic infiltration, ventricular arrangement of cells, and intracellular gaps. Details are described in Table .
Table 2. Variations of the characteristics of heart histopathology of random images in different groups: the Normal control (N.C.) group; (B) the Normal table salt (N.T.S.) group; (C) the Normal sea salt (N.S.S.) group; (D) the Doxorubicin-induced cardiac dysfunction control (D.C.) group; (E) the Doxorubicin-induced cardiac dysfunction control + Table salt (D.T.S.) group; (F) the Doxorubicin-induced cardiac dysfunction control + Sea salt (D.S.S.) group. The results are depicted in Figure . (−) Normal effect; (+) Slight effect (++) Mild effect; (+++) Severe effect.
3.6.2. Liver
The liver histopathological variations were defined for the central vein, portal vein, bile duct, hepatic artery, fibroblast, endothelial cells, sinusoid, binucleated cells, steatosis, Kupffer cells, eosinophil infiltration, and damaged hepatocytes. Details are described in Table .
Table 3. Variations of the characteristics of liver histopathology of random images in different groups: (A) the Normal control (N.C.) group; (B) the Normal table salt (N.T.S.) group; (C) the Normal sea salt (N.S.S.) group; (D) the Doxorubicin-induced cardiac dysfunction control (D.C.) group; (E) the Doxorubicin-induced cardiac dysfunction control + Table salt (D.T.S.) group; (F) the Doxorubicin-induced cardiac dysfunction control + Sea salt (D.S.S.) group. The results are depicted in figures N. (−) Normal effect; (+) Slight effect (++) Mild effect; (+++) Severe effect.
3.6.3. Kidney
Renal histopathological changes were recorded for the distribution of renal corpuscle, glomerular congestion, tubular casts, proximal convoluted tubule, distal convoluted tubule, macula densa cells, basal lamina, glomerular basement membrane, podocyte, eosinophilic infiltration, and mesangial cells. Details are described in Table .
Table 4. Variations of the characteristics of kidney histopathology of random images in different groups: the Normal control (N.C.) group; (B) the Normal table salt (N.T.S.) group; (C) the Normal sea salt (N.S.S.) group; (D) the Doxorubicin-induced cardiac dysfunction control (D.C.) group; (E) the Doxorubicin-induced cardiac dysfunction control + Table salt (D.T.S.) group; (F) the Doxorubicin-induced cardiac dysfunction control + Sea salt (D.S.S.) group. The results are depicted in Figure . (−) Normal effect; (+) Slight effect (++) Mild effect; (+++) Severe effect.
4. Discussion
There are several risk factors linked with the development of cardiac remodelings, such as myocardial infarction [Citation26], hypertension [Citation27], dyslipidaemia [Citation28], diabetes [Citation29], obesity [Citation30], smoking [Citation31], etc. There is growing interest in deciphering the role of a high salt diet on CVD [Citation32] as the average salt intake in most people in most nations often exceeds the physiological requirement [Citation33]. A salt diet is associated with many pathological changes in organ function that are independent of blood pressure regulation [Citation34]; the most common among these changes are associated with vascular injury [Citation35], endothelial dysfunction [Citation36] that may promote high impact on the progression of cardiac remodeling. However, as per the recommendation of the World Health Organization (WHO) reduction in a daily reduction of dietary salt can prevent CVD but reports are available where the salt reduction is associated with increased cholesterol and triglyceride levels [Citation37], insulin resistance [Citation38], increased sympathetic activity [Citation39] and variation in RAS activation [Citation40] along with an increase in atherogenesis activity [Citation41]. Accordingly, the present study was designed to investigate the hypothesis that how natural sea salt may differ from table salt on cardiac dysfunction in drug-induced disease models through the RAS system and both pro-inflammatory and anti-inflammatory cytokines along with other parameters.
Cardiac muscle is primarily rich in CKMB an isoenzyme from the CK category, that is released rapidly after myocardial variation either by injury or by the change in physiological activity [Citation42]. CKMB plays an important role in defining the normal state of cardiac tissue [Citation43]. In the present study, CK-MB marker levels were elevated significantly in all groups compared to normal control, N.T.S. and N.S.S. were able to control the elevated level of CKMB level in DOX that induces remodeled rats’ concept of the beneficial role of salt in reducing the remodeled state of the heart. The underlying mechanism may be associated with down-regulating the genes associated with TGFβ-dependent signalling pathway in the left ventricle and kidneys [Citation44] that can reverse the heart dysfunction and improves the function of CVS.
The RAS is one of the most studied systems in diseases associated with the cardiovascular system. This system in long run is directly associated with cardiac dysfunction that maintains haemodynamic homeostasis [Citation45] through sympathetic activity and sodium water retention [Citation46]. Furthermore, the RAS system is associated with and responsible for intracrine AngII that controls myocyte hypertrophy in pathophysiological conditions [Citation47]. Our study demonstrates that N.T.S. significantly elevates the renin level compared to N.C. experimental animals, the crux of the study deciphers that DOX does not always increase renin in experimental animals, it can also decrease to a significant level. The increase in AngII level is significantly higher in D.T.S. compared to D.S.S. suggesting the importance of normal sea salt in regulating the AngII level in the remodeled heart. Our results are concurrent with the study proposed by Rosa et al. in 2020 [Citation48,Citation49]. These results may highlight some alternative mechanisms that might be working other than the pure RAS pathway.
It is evident and established that circulating IL-6 has a close correlation with geometrical changes of the left ventricle [Citation50] in the cardiac remodeled patient [Citation51]. IL-6 plays a more important role in inflammation associated with left ventricular dysfunction especially after re-perfused myocardial infarction compared to other circulating cytokines [Citation52]. Furthermore, reports are available that establish the presence of inflammatory cytokines along with acute-phase proteins such as CRP in cardiac toxicity and dysfunction [Citation53]. Our data demonstrate that the increased level of IL-6 in the D.C. group compared to the N.C. group is significantly high. On the other side, the level of IL-6 decreased significantly in both D.T.S. and D.S.S. groups compared with the D.C. group establishing the role of these salts on the cardiovascular system. However, the effect on IL-6 was more profuse in D.T.S. as compared to D.S.S.
IL-10 is a well-established anti-inflammatory cytokine that counters the production of proinflammatory cytokine. The deficiency of IL-10 exaggerates LV dysfunction and inflammation [Citation54]. Researchers have proved that IL-10 deficiency can inhibit dysfunction even after an elevated level of myocardial TNF-α. Elevated [Citation55]. Our study demonstrated that the level of IL-10 was significantly low in the D.C. group as compared to elevated levels in D.T.S and D.S.S. groups. The elevated level was near normal in this group as that of the normal healthy group (N.C.). There was no significant difference between N.T.S. and N.S.S. on IL-10 confirming the positive role of these salts on cardiac remodeling.
Excess deposition of extra-cellular matrix in cardiomyocytes is one of the important characteristics of cardiac fibrosis, playing an important role in most of the cardiopathological variation including remodeling [Citation56]. The present scientific advancement is still unable to decipher the nongenerative nature of adult mammalian myocardial tissue, particularly in the biventricular fibrotic modelling of the heart. The inflammatory reaction is activated after the rapid loss of cardiac muscle in response to acute myocardial infarction, in the end, causing the replacement of lost cardiac muscle with a collagen-based scar Myocytes of ventricles are closely organized in bands [Citation57]; separation of adjacent layers is visualized by cleft. Cardiac muscle architecture is characterized by a complex network of extracellular matrix proteins, with fibrillar collagen in abundance. Along with mast cells, eosinophils from the category of granulocyte leukocytes play an important function in allergic reactions such as asthma, parasitic infection and internal inflammation [Citation58]. A decrease in peripheral eosinophils is linked to an increased risk of mortality in Myocardial infarct patients, decrease in the chance of repair is associated with a low count of eosinophils due to the presence of preformed IL-4 in these cells, a mechanism is associated with their anti-inflammatory response. Still, this is a topic of debate among health scientists whether eosinophil plays any constructive role in the repair of infarct tissue [Citation59]. Our study demonstrates that the D.C. group exhibited an increase in the diameters of cardiac myocytes along with a reduction in the flexibility of muscle which is a result of increased metabolic demands of heart cells, that in turn, influence the performance of the heart. Furthermore, interstitial spaces, distorted intercalated discs, irregular cell arrangement with an increase in fat and fibrinogen deposition along with irregular and increased distribution of eosinophilic infiltration were also observed (Figure (D)). Similar morphological variations were demonstrated in the D.T.S. group with reduced fat and fibrinogen deposition (Figure (E)). In contrast, the D.S.S. group exhibited maintaining the majority of the characteristics similar to the N.C. group with minor variation in fibrinogen deposition moderately affected (++) as compared to the D.C. group (Figure (F)).
Liver histopathological changes were studied for the normal central vein (CV) in the N.C. group, sinusoids that penetrate the wall of the vein and the paucity of surrounding connective tissue. In the N.C. group, CV appears as a circular, the hepatic cells appear as cords radiating from the central vein. Kupffer cells (KC) are the more prominent and they exhibit a large nucleus and a substantial amount of cytoplasm. The endothelial cell (EN) has a smaller nucleus, attenuated cytoplasm and a smooth surface contour. In addition to the normal shape of the portal vein (PV), bile duct (BD), hepatic artery (HA) along with the normal availability of fibroblast and binucleated cells a slight eosinophilic infiltration was also studied. Additionally, there is a normal deposition of fat or steatosis (Figure (A)). In this experiment, the D.C. group exhibits significant pathological changes among characteristics compared to the N.C. group. However, in the D.T.S. group, the severity of injury increased in all markers except the presence of sinusoids which was more regular than those in the D.C. group (Figure (E)). On the contrary, the D.S.S. group compared to the D.C. group shows the recovery of the CV and PV in parallel with the normal structure of BD. Nonetheless, the distortion of HA and damage of hepatic cells compared to the D.C. group were few in the D.S.S. group along with very less fibroblast, EN, binucleated cells, steatosis, KC, eosinophilic infiltration, as well as, regular distribution of fat deposition or sinusoid in this group (Figure (F)), which suggest the role of sea salt in maintaining the normal structure of the liver. Furthermore, the alteration in D.T.S & D.S.S on the hepatic artery can be associated with multiple factors including vascular disease, hypertension, diabetes, etc. [Citation60]. The metabolism of doxorubicin in the presence of sea salt or table salt might have altered which could have led to changes in the hepatic artery of these group animals. Furthermore, it is well established the metabolism of doxorubicin in kidneys and the liver alters the Cytochrome P450 Expression and Arachidonic Acid Metabolism [Citation61]. Salt is known to induce micro vascularization of the organ that becomes a critical factor in hypertension-mediated organ damage [Citation62]. The combined effect of Doxorubicin and salt may be the reason behind inflammation of the hepatic artery.
Figure 3. Liver histopathology of random images in different groups: (A) the Normal control (N.C.) group; (B) the Normal table salt (N.T.S.) group; (C) the Normal sea salt (N.S.S.) group; (D) the Doxorubicin-induced cardiac dysfunction control (D.C.) group; (E) the Doxorubicin-induced cardiac dysfunction control + Table salt (D.T.S.) group; (F) the Doxorubicin-induced cardiac dysfunction control + Sea salt (D.S.S.) group. The figures define the following characteristics: Central vein (black star), portal vein (blue star), bile duct (green star), hepatic artery (red star), fibroblast (light green arrow), endothelial cells (orange arrow), sinusoid (purple arrow), binucleated cells (green arrow), steatosis (grey arrow), Kupffer cells (blue arrow), eosinophil infiltration (black arrow), damaged hepatocytes (red arrow). Original magnification was – 20×; Scale = 50 µm.
Figure 4. Kidney histopathology of random images in different groups: (A) the Normal control (N.C.) group; (B) the Normal table salt (N.T.S.) group; (C) the Normal sea salt (N.S.S.) group; (D) the Doxorubicin-induced cardiac dysfunction control (D.C.) group; (E) the Doxorubicin-induced cardiac dysfunction control + Table salt (D.T.S.) group; (F) the Doxorubicin-induced cardiac dysfunction control + Sea salt (D.S.S.) group. The figures define the following characteristics: Distribution of renal corpuscle (black star), glomerular congestion (blue star), tubular casts (grey star), proximal convoluted tubule (light green arrow), distal convoluted tubule (purple arrow), macula densa cells (grey arrow), basal lamina (green arrow), glomerular basement membrane (orange arrow), podocyte (red arrow), eosinophilic infiltration (black arrow) and mesangial cells (blue arrow). Original magnification – (A1–F1) 10×; Scale = 100 µm & (A2–F2) 40×; Scale = 50 µm.
The bowman or renal corpuscles (RC) appear as relatively large spherical structures, surrounded by proximal and distal convoluted tubules. The proximal convoluted tubules (PC) have a slightly large diameter than the distal convoluted tubules (DC). The PC have a brush border, whereas the DC have a clear, sharper luminal surface. The lumen of the PC is often star-shaped; this is not the case with DC. Typically, fewer nuclei appear across the section of PC than in an equivalent segment of a DC. Additionally, the RC is divided into two parts: (1) a parietal layer (basal lamina), (2) a visceral layer (glomerular basement membrane) consisting of cells called podocytes (Pod) that lie on the outer surface of the glomerular capillary. The mesangial cells are also a component of the glomerulus. The cells of the distal tubule are more crowded on one side. These crowded cells constitute the macula densa (MD) that lies adjacent to the afferent arteriole. In our study, both D.C. and D.T.S. groups exhibited a similar pattern in terms of various observed altered histopathological variations in renal tissue. Mild to moderate effect of renal corpuscle distribution, and distal tubule structure with the maintenance of macula densa cells in the D.T.S. group compared to the D.C. group was noted (Figure (D,E)). In the D.S.S. group, most of the markers were in a normal state with a slightly irregular distribution of renal corpuscle, podocyte and mesangial cells compared to the D.C. group. Additionally, a moderate effect was also observed in glomerular congestion and proximal convoluted tubule (Figure (F)).
Cardiac hypertrophy and an increase in collagen deposits are the two major changes associated with heart failure after cardiac injury. Collagen levels in non-infarct cardiac tissue are a critical step towards cardiac contractile malfunction and structural dysfunction [Citation63]. Many studies have demonstrated the role of the cardiac RAS system after cardiac injury or toxicity [Citation64]. There is no clear demarcation between the role of RAS and collagen deposits but it may be the hypothesis that the collagen deposits alter with variation in the RAS system [Citation65]. The present protocol highlights that despite a much low level of renin in the DOX control group the salts were able to restore the level of rennin in D.T.S. and D.S.S. groups opening new frontiers in cardiac studies. Furthermore, the RAS has decrease interstitial fibrosis and interstitial dysfunction, which can reduce the hypertrophy of LV and myocardial oxidative damage, improve endothelial function and can reduce the formation of thrombosis with improved vascular functions [Citation66] factors associated with low salt diet in relation to reducing collagen deposit despite the altered RAS system [Citation67].
5. Conclusion
The results of the present study deciphered the therapeutic advantage of sea salt over table salt (refined) in doxorubicin-induced cardiac toxicity model. The change in the Renin-Angiotensin System is independent of pro- and anti-inflammatory markers like IL-6 and IL-10, respectively. Simultaneously the toxicity induced by Dox has a profound effect on the biochemical parameters of the heart via CKMB, kidneys via renin and the liver via Angiotensin II, respectively. The histopathological changes in sea salt-treated animals represent the marked effects on the architectural pattern of the heart, liver and kidney tissue further confirming the beneficial effects of sea salt over table salt. The additives used in refining the salt may have altered the true nature of table salt, which need further investigation.
Acknowledgements
The authors acknowledge with thanks to DSR for technical and financial support.
Disclosure statement
No potential conflict of interest was reported by the author(s).
Additional information
Funding
References
- Kaptoge S, Pennells L, De Bacquer D, et al. World Health Organization cardiovascular disease risk charts: revised models to estimate risk in 21 global regions. Lancet Glob Health. 2019;7(10):e1332–e1345.
- Verdecchia P, et al. Sudden cardiac death in hypertensive patients. Hypertension. 2019;73(5):1071–1078.
- Al-Orabi AS, et al. Levocetirizine and amlodipine restores hepato-cardiac function in the forced swim-induced cardiac remodelling Rat model. J Pharmaceut Res Int. 2018: 1–10.
- Cokkinos, D.V., Cardiac remodeling: the course toward heart failure–I. General concepts. In Myocardial preservation. Springer; 2019. p. 215–245.
- Matsumura K, et al. Cardiac rupture due to reinfarction in the early phase of apical myocardial infarction. Int Heart J. 2019;60(4):974–978.
- Gelosa P, et al. Drug repurposing in cardiovascular diseases: opportunity or hopeless dream? Biochem Pharmacol. 2020;113894.
- Skjødt MK, Frost M, Abrahamsen B. Side effects of drugs for osteoporosis and metastatic bone disease. Br J Clin Pharmacol. 2019;85(6):1063–1071.
- Ha SK. Dietary salt intake and hypertension. Electrolytes Blood Press. 2014;12(1):7–18.
- Choi HY, Park HC, Ha SK. Salt sensitivity and hypertension: a paradigm shift from kidney malfunction to vascular endothelial dysfunction. Electrolytes Blood Press. 2015;13(1):7–16.
- Rust P, Ekmekcioglu C. Impact of salt intake on the pathogenesis and treatment of hypertension. In Hypertension: from basic research to clinical practice. Springer; 2016. p. 61–84.
- Lackland DT, Weber MA. Global burden of cardiovascular disease and stroke: hypertension at the core. Can J Cardiol. 2015;31(5):569–571.
- Doukky R, et al. Impact of dietary sodium restriction on heart failure outcomes. JACC: Heart Failure. 2016;4(1):24–35.
- Humalda JK, Navis G. Dietary sodium restriction: a neglected therapeutic opportunity in chronic kidney disease. Curr Opin Nephrol Hypertens. 2014;23(6):533.
- Trieu K, et al. Salt reduction initiatives around the world – a systematic review of progress towards the global target. PLoS One. 2015;10(7):e0130247.
- Mente A, et al. Urinary sodium excretion, blood pressure, cardiovascular disease, and mortality: a community-level prospective epidemiological cohort study. Lancet. 2018;392(10146):496–506.
- Wang J, et al. Long-term low salt diet increases blood pressure by activation of the renin-angiotensin and sympathetic nervous systems. Clin Exp Hypertens. 2019;41(8):739–746.
- He FJ, Burnier M, MacGregor GA. Nutrition in cardiovascular disease: salt in hypertension and heart failure. Eur Heart J. 2011;32(24):3073–3080.
- Klein L, et al. Lower serum sodium is associated with increased short-term mortality in hospitalized patients with worsening heart failure: results from the outcomes of a prospective trial of intravenous milrinone for exacerbations of chronic heart failure (OPTIME-CHF) study. Circulation. 2005;111(19):2454–2460.
- Schroten NF, et al. Effect of additive renin inhibition with aliskiren on renal blood flow in patients with chronic heart failure and renal dysfunction (additive renin inhibition with aliskiren on renal blood flow and neurohormonal activation in patients with chronic heart failure and renal dysfunction). Am Heart J. 2015;169(5):693–701.e3.
- Carvalho FS, et al. Doxorubicin-induced cardiotoxicity: from bioenergetic failure and cell death to cardiomyopathy. Med Res Rev. 2014;34(1):106–135.
- Timm KN, et al. Early detection of doxorubicin-induced cardiotoxicity in rats by its cardiac metabolic signature assessed with hyperpolarized MRI. Commun Biol. 2020;3(1):1–10.
- Forechi L, et al. Effects of high and low salt intake on left ventricular remodeling after myocardial infarction in normotensive rats. J Am Soc Hypertens. 2015;9(2):77–85.
- Ikonomidis I, et al. Multimarker approach in cardiovascular risk prediction. Dis Markers. 2009;26(5, 6):273–285.
- Dastan F, et al. The potential effect of intravenous calcitriol on the ischemia-reperfusion injury and inflammatory biomarkers in patients following percutaneous coronary intervention (PCI). Iran J Pharm Res. 2019;18(Special Issue):282–290.
- Hasan KMM, Tamanna N, Haque MA. Biochemical and histopathological profiling of Wistar rat treated with Brassica napus as a supplementary feed. Food Sci Hum Wellness. 2018;7(1):77–82.
- Wang J, et al. Proline improves cardiac remodeling following myocardial infarction and attenuates cardiomyocyte apoptosis via redox regulation. Biochem Pharmacol. 2020: 114065.
- Mendes AS, et al. Verapamil decreases calpain-1 and matrix metalloproteinase-2 activities and improves hypertension-induced hypertrophic cardiac remodeling in rats. Life Sci. 2020;244:117153.
- Puato M, et al. Lipid profile, inflammatory cytokines and vascular remodeling in dyslipidemic subjects treated with nutraceuticals. J Hypertens. 2019;37:e187.
- Yap J, et al. Association of diabetes mellitus on cardiac remodeling, quality of life, and clinical outcomes in heart failure with reduced and preserved ejection fraction. J Am Heart Assoc. 2019;8(17):e013114.
- Piché M-E, Tchernof A, Després J-P. Obesity phenotypes, diabetes, and cardiovascular diseases. Circ Res. 2020;126(11):1477–1500.
- Khoramdad M, et al. Association between passive smoking and cardiovascular disease: a systematic review and meta-analysis. IUBMB Life. 2020;72(4):677–686.
- Lampuré A, et al. Relationship between sensory liking for fat, sweet or salt and cardiometabolic diseases: mediating effects of diet and weight status. Eur J Nutr. 2020;59(1):249–261.
- Khan MS, Jones DW, Butler J. Salt, no salt, or less salt for patients with heart failure? Am J Med. 2020;133(1):32–38.
- Catena C, et al. Salt, aldosterone, and parathyroid hormone: what is the relevance for organ damage? Int J Endocrinol. 2017;2017.
- Cole NI, et al. The association between serum sodium concentration, hypertension and primary cardiovascular events: a retrospective cohort study. J Hum Hypertens. 2019;33(1):69–77.
- Cheng P, et al. Xin-Ji-Er-Kang alleviates myocardial infarction-induced cardiovascular remodeling in rats by inhibiting endothelial dysfunction. BioMed Res Int. 2019;2019.
- Kang HJ, et al. Low salt and low calorie diet does not reduce more body fat than same calorie diet: a randomized controlled study. Oncotarget. 2018;9(9):8521.
- Oh H, et al. Low salt diet and insulin resistance. Clin Nutr Res. 2016;5(1):1–6.
- Baqar S, et al. Effect of salt supplementation on sympathetic activity and endothelial function in salt-sensitive type 2 diabetes. J Clin Endocrinol Metab. 2020;105(4):e1187–e1200.
- de Borst MH, Navis G. Sodium intake, RAAS-blockade and progressive renal disease. Pharmacol Res. 2016;107:344–351.
- Fusco FB, et al. Low-sodium diet induces atherogenesis regardless of lowering blood pressure in hypertensive hyperlipidemic mice. PLoS One. 2017;12(5):e0177086.
- Savic-Radojevic A, Pljesa-Ercegovac M, Matic M, et al. Novel biomarkers of heart failure. Adv Clin Chem. 2017;79:93–152.
- Shah S, et al. EGFR tyrosine kinase inhibition decreases cardiac remodeling and SERCA2a/NCX1 depletion in streptozotocin induced cardiomyopathy in C57/BL6 mice. Life Sci. 2018;210:29–39.
- Zhang Y, Wei W, Shilova V, et al. Monoclonal antibody to marinobufagenin downregulates TGFß profibrotic signaling in left ventricle and kidney and reduces tissue remodeling in salt-sensitive hypertension. J Am Heart Assoc. 2019;8(20):e012138.
- Chirakarnjanakorn S, et al. Cardiovascular impact in patients undergoing maintenance hemodialysis: clinical management considerations. Int J Cardiol. 2017;232:12–23.
- Gafane-Matemane LF, et al. Relation of the renin–angiotensin–aldosterone system with potential cardiac injury and remodelling: the SABPA study. Blood Press. 2020;29(1):31–38.
- Reyes S, et al. Novel cardiac intracrine mechanisms based on Ang-(1-12)/chymase axis require a revision of therapeutic approaches in human heart disease. Curr Hypertens Rep. 2017;19(2):16.
- Rosa G, et al. High salt intake during puberty leads to cardiac remodelling and baroreflex impairment in lean and obese male Wistar rats. Br J Nutr. 2020;123(6):642–651.
- Allen LA, et al. High salt diet impairs cerebral blood flow regulation via salt-induced angiotensin II suppression. Microcirculation. 2019;26(3):e12518.
- Butt N, et al. Pentraxin 3 in primary percutaneous coronary intervention for ST elevation myocardial infarction is associated with early irreversible myocardial damage: kinetic profile, relationship to interleukin 6 and infarct size. Eur Heart J Acute Cardiovasc Care. 2020;9(4):302–312.
- Ohtsuka T, et al. Relation of circulating interleukin-6 to left ventricular remodeling in patients with reperfused anterior myocardial infarction. Clin Cardiol. 2004;27(7):417–420.
- Bartekova M, et al. Role of cytokines and inflammation in heart function during health and disease. Heart Fail Rev. 2018;23(5):733–758.
- Altara R, et al. CXCL10 is a circulating inflammatory marker in patients with advanced heart failure: a pilot study. J Cardiovasc Transl Res. 2016;9(4):302–314.
- Sikka G, et al. Interleukin 10 knockout frail mice develop cardiac and vascular dysfunction with increased age. Exp Gerontol. 2013;48(2):128–135.
- Yue Y, et al. Interleukin-10 deficiency alters endothelial progenitor cell–derived exosome reparative effect on myocardial repair via integrin-linked kinase enrichment. Circ Res. 2020;126(3):315–329.
- Berk BC, Fujiwara K, Lehoux S. ECM remodeling in hypertensive heart disease. J Clin Invest. 2007;117(3):568–575.
- Leonard BL, Smaill BH, LeGrice IJ. Structural remodeling and mechanical function in heart failure. Microsc Microanal. 2012;18(1):50.
- Blanchard C, Rothenberg ME. Biology of the eosinophil. Adv Immunol. 2009;101:81–121.
- Toor IS, et al. E Eosinophils have an essential role in cardiac repair following myocardial infarction. Heart. 2017;103(Suppl 5):A152–A152.
- Rosenberg A, et al. Management of hepatic artery aneurysm: a case series. Ann Hepato-Biliary-Pancreatic Surg. 2020;24(3):333–338.
- Zordoky BN, et al. Acute doxorubicin toxicity differentially alters cytochrome P450 expression and arachidonic acid metabolism in rat kidney and liver. Drug Metab Dispos. 2011;39(8):1440–1450.
- Marketou ME, et al. Salt-induced effects on microvascular function: a critical factor in hypertension mediated organ damage. J Clin Hypertens. 2019;21(6):749–757.
- Sant’Ana PG, et al. Heart remodeling produced by aortic stenosis promotes cardiomyocyte apoptosis mediated by collagen V imbalance. Pathophysiology. 2018;25(4):373–379.
- Huang BS, Leenen FH. The brain renin-angiotensin-aldosterone system: a major mechanism for sympathetic hyperactivity and left ventricular remodeling and dysfunction after myocardial infarction. Curr Heart Fail Rep. 2009;6(2):81–88.
- Lang CC, Struthers AD. Targeting the renin–angiotensin–aldosterone system in heart failure. Nat Rev Cardiol. 2013;10(3):125.
- Wu Q-Q, et al. Mechanisms contributing to cardiac remodelling. Clin Sci. 2017;131(18):2319–2345.
- Urabe A, et al. Effects of eplerenone and salt intake on left ventricular remodeling after myocardial infarction in rats. Hypertens Res. 2006;29(8):627–634.