Abstract
This study investigates the anticancer potential of an Acyclovir-loaded cod liver oil nanoemulsion, combining the health benefits of cod liver oil with the therapeutic effects of Acyclovir, a drug traditionally used for treating viral infections but repurposed here for cancer therapy. The nanoemulsion demonstrated improved solubility of Acyclovir, with optimal physical properties conducive to effective drug delivery. Key findings include a particle size of 114 ± 5 nm, a zeta potential of −7 ± 3 mV and a high drug content of 98.5 ± 1.25%, with maintained stability over three months. Notably, the nanoemulsion induced significant apoptotic cell death and cell cycle arrest in the G2 phase across all tested cancer cell lines, highlighting its potent anticancer activity.
1. Introduction
In recent years, the search for innovative and more effective cancer therapies has intensified, leading researchers to explore not only new pharmaceutical compounds but also the repurposing of existing drugs and the use of natural products. Among natural products, cod-liver oil, rich in omega-3 fatty acids, vitamins A and D, has been traditionally celebrated for its multitude of health benefits [Citation1]. Emerging research suggests that cod-liver oil may possess anticancer properties, attributed to its ability to modulate inflammatory pathways, induce apoptosis in cancer cells, and potentially inhibit tumour growth. These promising attributes position cod-liver oil as a potential adjunct or primary agent in cancer therapy [Citation2, Citation3].
Simultaneously, Acyclovir, a well-established antiviral medication primarily used for the treatment of herpes simplex virus infections, has emerged as a candidate for drug repurposing in cancer therapy [Citation4]. The repurposing of Acyclovir is based on its potential anticancer effects, which are hypothesized to result from its ability to interfere with DNA synthesis in cancer cells, thereby inhibiting their proliferation [Citation5]. This novel application of Acyclovir could offer a strategic advantage in combating cancer, particularly in virus-associated cancers, where Acyclovir’s antiviral and anticancer mechanisms may synergistically contribute to therapeutic outcomes [Citation6].
However, the efficacy of both cod-liver oil and Acyclovir in cancer therapy can be limited by factors such as solubility, bioavailability, and targeted delivery. Nanoemulsion delivery systems represent a cutting-edge solution to these challenges, offering a means to enhance the solubility and stability of hydrophobic compounds, improve bioavailability, and enable targeted drug delivery [Citation7]. Nanoemulsions, characterized by their small droplet size and high surface area, can facilitate the efficient encapsulation and controlled release of therapeutic agents, reducing systemic side effects and improving therapeutic efficacy [Citation8].
This study aims to exploit the anticancer potential of cod-liver oil and repurpose Acyclovir for cancer therapy through the development of a cod-liver oil-based nanoemulsion. By incorporating Acyclovir into this novel delivery system, we seek to investigate the formulation’s physical characteristics, stability, and anticancer efficacy. The primary objectives include evaluating the nanoemulsion’s particle size distribution, zeta potential, and stability over time, alongside assessing its anticancer activity against selected cancer cell lines. This research endeavours to provide a foundational understanding of the synergistic effects of cod-liver oil and Acyclovir when formulated as a nanoemulsion, potentially paving the way for innovative strategies in cancer treatment.
2. Methodology
2.1. Assessing the solubility of Acyclovir in cod liver oil, surfactant and co-surfactant
Determining Acyclovir’s solubility involved its dispersion in cod liver oil, utilizing Tween 80 and Span 20 as the surfactant and co-surfactant, respectively. A surplus amount of Acyclovir powder was added to each oil solution (about 5 ml). The mixtures were stirred using a magnetic stirrer for 72 hours at ambient temperature to facilitate dissolution. Subsequently, to remove any residual undissolved particles, the mixtures were centrifuged at a speed of 10,000 rpm for a duration of 10 minutes. The resultant transparent supernatant was then mixed with methanol, and the absorbance levels were recorded with a spectrophotometer, tuned to an Acyclovir-specific wavelength. As reference points, methanol was also mixed with the oil solutions and measured as control blanks [Citation9, Citation10].
2.2. Creating Acyclovir-loaded cod liver oil nanoemulsion
To develop the Acyclovir-loaded cod liver oil nanoemulsion, a mixture comprising 6 ml of cod liver oil, 2.5 ml of Tween 80, and 1.5 ml of Span 20 was used. These ingredients were precisely measured and mixed thoroughly to form a homogeneous oil phase. Utilizing the phase inversion method, the nanoemulsion was then crafted. Initially, a quarter of the water phase, amounting to 22.5 ml, was combined with the oil pre-mixture. This step was followed by the careful addition and blending of the rest of the water phase (67.5 ml), ensuring a gradual and even incorporation. In the preparation of the nanoemulsion containing the medication, the process mirrored the above, with the distinction that 3 mg of Acyclovir was first dissolved into the oil pre-mixture before continuing with the remaining steps [Citation11].
2.3. Evaluating particle size, zeta potential, and polydispersity index (PDI):
The sizes of the particles, their charge (zeta potential), and the distribution uniformity (PDI) were assessed through dynamic light scattering (DLS) for size and a separate zeta potential analysis for charge, using specific settings tailored for each measurement. To prepare for these evaluations, the samples were initially diluted with ultra-pure water. These assessments were carried out under ambient conditions [Citation12].
2.4. Determining the concentration of Acyclovir in cod liver oil nanoemulsion
To ascertain the amount of Acyclovir present in the cod liver oil nanoemulsion, 1 ml of the mixture was dissolved in 10 ml of methanol. Following dissolution, the solution underwent centrifugation at a speed of 5000 rpm for 30 minutes. The resulting transparent supernatant was then gathered for analysis. The concentration of Acyclovir within the supernatant was measured using a spectrophotometer, with observations made at the peak absorbance wavelength of 254 nm [Citation11, Citation12].
2.5. Examining the stability of optimized Acyclovir-loaded cod liver oil nanoemulsion
Stability tests on the Acyclovir-loaded cod liver oil nanoemulsion were performed following the International Conference on Harmonisation (ICH) standards. In summary, the nanoemulsion samples were kept at a temperature of 4 ± 0.5°C over three months. To assess the stability of the formulations, parameters such as droplet size, zeta potential, and the concentration of Acyclovir in the nanoemulsions were measured and monitored [Citation11].
2.6. In vitro drug release
The drug release study was conducted using a dialysis membrane method. A pre-soaked dialysis membrane with a molecular weight cut-off of 12,000–14,000 Da was used. The nanoemulsion was placed in the dialysis bag and immersed in 100 ml of phosphate buffer (pH 7.4) at 37°C. Samples were taken at predetermined time intervals, and the Acyclovir concentration was analyzed using a spectrophotometer at 254 nm.
2.7. Cell lines preparation
Human breast adenocarcinoma (MCF-7), liver carcinoma cells (HePG2), and colon adenocarcinoma (HCT 116) cell lines sourced from the American Type Culture Collection (ATCC, Boston, MA, USA) were cultured in RPMI 1640 medium supplemented with 10% fetal bovine serum and 100 IU/mL penicillin/streptomycin [Citation13].
2.8. Cell viability assay
The anticancer efficacy of Acyclovir solution, cod liver oil nanoemulsion, and Acyclovir-loaded cod liver oil nanoemulsion was assessed using the sulforhodamine B (SRB) assay. Cells were plated at a density of 1X 104 cells per well in 96-well plates, treated with different concentrations of each formulation, and incubated for 72 hours at 37°C. Following treatment, cells were fixed, stained, and analyzed for optical density [Citation13].
2.9. Cell cycle analysis
Cells were plated at a density of 1 X 105 cells per well in 6-well plates, treated with the formulations at their IC50 values, and incubated for 48 hours. After incubation, cells were harvested, fixed, stained with propidium iodide (PI), and analyzed using flow cytometry [Citation13].
2.10. Apoptosis analysis
Annexin V-FITC/propidium iodide staining was used to analyze apoptosis induction. Cells were cultured, treated with formulations at their IC50 values, and incubated for 48 hours. Following treatment, cells were stained with Annexin V-FITC/PI and analyzed using flow cytometry [Citation13].
2.11. Statistical analysis
Experiments were performed in triplicate, and results were expressed as mean ± SD. Statistical significance was determined using Student’s t-test and one-way analysis of variance (ANOVA) with p values < 0.05 considered statistically significant.
3. Result and discussion
3.1. Solubility Study
The solubility study of Acyclovir was conducted in three different solvents: Cod Liver oil, Tween 80, and Span 20. The solubility results are as follows: Cod Liver oil (10.1 ± 0.15 mg/mL), Tween 80 (20 ± 1.35 mg/mL), and Span 20 (21.6 ± 1.2 mg/mL) (Table ).
Table 1. Solubility of Acyclovir in Cod Liver Oil, Tween 80, and Span 20.
The solubility of Acyclovir in Cod Liver oil was sufficient for our preparation however the solubility was relatively low compared to the surfactants Tween 80 and Span 20. This could be attributed to Cod Liver oil, a natural oil with limited solubilizing capacity for hydrophilic compounds like Acyclovir [Citation14, Citation15].
On the other hand, both Tween 80 and Span 20 are nonionic surfactants known for enhancing the solubility of hydrophobic compounds. The higher solubility of Acyclovir in Tween 80 and Span 20 can be attributed to their surfactant properties, which allow them to form micelles and solubilize hydrophobic compounds like Acyclovir [Citation16].
3.2. Characterization and drug content of Acyclovir-loaded cod liver oil nanoemulsion
The zeta potential of the Acyclovir-loaded cod liver oil nanoemulsion was measured to be −7 ± 3 mV. This indicates that the nanoemulsion particles have a slight negative charge, which could contribute to their stability by preventing aggregation through electrostatic repulsion [Citation17].
The nanoemulsion’s particle size was 114 ± 5 nm, indicating that the particles are in the nanometer range. This is advantageous for drug delivery applications, as smaller particles have a larger surface area, which can enhance drug release and absorption [Citation18].
The nanoemulsion’s polydispersity index (PDI) was measured to be 0.42, indicating a moderate size distribution of the particles [Citation19].
The percentage of drug content in the nanoemulsion was determined to be 98.5 ± 1.25%. This high drug content indicates that the formulation is efficient in loading Acyclovir into the nanoemulsion, which is crucial for ensuring the effectiveness of the drug delivery system [Citation20].
Overall, the physical characterization results suggest that the Acyclovir-loaded cod liver oil nanoemulsion is a promising drug delivery formulation with favourable zeta potential, particle size, PDI, and drug content (Table ).
Table 2. Physical characterization and percentage of drug content for Acyclovir loaded cod liver oil Nanoemulsion.
3.3. Stability study
After 3 months of storage, the zeta potential of the Acyclovir-loaded cod liver oil nanoemulsion slightly decreased to −9 ± 1.45 mV compared to the fresh sample (−7 ± 3 mV). This change indicates a slight decrease in the stability of the nanoemulsion, as a more negative zeta potential is generally associated with better stability due to increased electrostatic repulsion between particles [Citation21]. The particle size of the stored nanoemulsion increased to 139 ± 3.8 nm from 114 ± 5 nm in the fresh sample. This increase suggests some degree of particle aggregation or coalescence over time, which could lead to reduced drug delivery efficiency [Citation19].
The polydispersity index (PDI) of the stored nanoemulsion also increased to 0.48 from 0.42 in the fresh sample. This indicates a broader size distribution of particles in the stored sample, further suggesting some degree of particle aggregation [Citation19].
The percentage of drug content in the stored nanoemulsion decreased to 92.4 ± 1.36% from 98.5 ±1.25% in the fresh sample. This decrease could be due to drug degradation or leaching from the nanoemulsion over time [Citation22].
Overall, the stability study results suggest that the Acyclovir-loaded cod liver oil nanoemulsion undergoes some changes in physical properties and drug content after 3 months of storage. These changes, such as the decrease in zeta potential, increase in particle size and PDI, and decrease in drug content, indicate a decrease in the stability and possibly the efficacy of the nanoemulsion over time (Table ). Further studies are needed to optimize the formulation and storage conditions to improve its stability and shelf life.
Table 3. Physical characterization and percentage of drug content for fresh and Stored Acyclovir loaded code liver oil Nanoemulsion.
3.4. In vitro drug release
The in vitro drug release profile of Acyclovir-loaded cod liver oil nanoemulsion showed a gradual increase in the percentage of drug release over 24 hours. Initially, at 0.5 hours, the average release was 7.5%. This value steadily increased to 10.37% at 1 hour, 14% at 2 hours, and reached 22.4% by 3 hours. A more pronounced release was observed at 4 hours (28.67%), continuing to rise significantly to 39.67% at 5 hours and 49.57% at 6 hours. The release rate then sharply increased, reaching 80.3% by 12 hours, and plateaued around 83.67% from 14 to 24 hours. In comparison, the Acyclovir solution showed a faster initial release, starting at 19.6% at 0.5 hours and quickly escalating to 36.47% by 1 hour, 51.5% by 2 hours, and 70.07% by 3 hours. The release reached its peak at 4 hours (73.67%) and maintained this plateau from 5 to 24 hours (Figure ).
Figure 1. The cumulative release of Acyclovir from both the Acyclovir-loaded cod liver oil nanoemulsion and Acyclovir solution was measured through a dialysis membrane with a molecular weight cut-off of 12,000−14,000 Da over a 24-hour period.
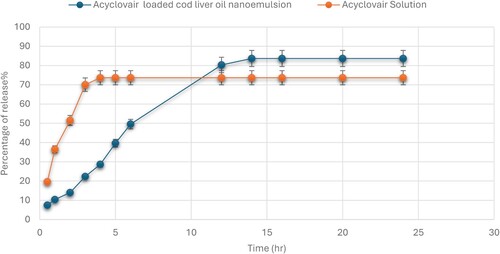
The Acyclovir-loaded cod liver oil nanoemulsion exhibited a controlled and sustained release pattern over the 24-hour period. The initial slower release rate compared to the Acyclovir solution suggests that the nanoemulsion formulation effectively encapsulates the drug, leading to a gradual release [Citation23]. This could be advantageous for maintaining therapeutic drug levels over extended periods, potentially reducing dosing frequency and improving patient compliance.
The rapid release observed with the Acyclovir solution indicates a quicker diffusion of the drug into the medium, which, while beneficial for immediate therapeutic action, may require more frequent dosing to maintain effective drug concentrations. The plateau in drug release observed for both formulations after certain time points suggests a balance between drug diffusion and medium saturation. For the nanoemulsion, the sustained release up to 24 hours highlights its potential for prolonged drug delivery, which is particularly beneficial for treatments requiring consistent drug levels [Citation24]. Overall, the results underscore the effectiveness of the cod liver oil nanoemulsion as a delivery system for Acyclovir, offering a controlled release that could enhance treatment outcomes for conditions requiring sustained drug availability.
3.5. In vitro cytotoxicity of Acyclovir loaded cod – liver oil nano emulsion
The sulforhodamine B (SRB) assay is commonly used to assess cell viability by measuring cellular protein content. This study used the SRB assay to evaluate the cytotoxic potential of Acyclovair-loaded cod liver oil nanoemulsion on MCF-7, HepG2, and HCT 116 cells. Acyclovir significantly reduced the viability of all tested cell lines in a dose-dependent manner, as shown in Figures x–x. The IC50 values for Acyclovir on MCF-7, HepG2, and HCT 116 cells were 1.3 ± 0.1 µg/mL, 8.3± 1.03 µg/mL, and 1.5 ± 0.03 µg/mL, respectively (Table ).
Table 4. The half-maximal inhibitory concentration (IC50) of various formulations on various human solid tumour cells.
Cod liver oil nanoemulsion exhibited a pronounced cytotoxic effect against all tested cell lines, with an IC50 of 3.6 ± 0.4 µg/mL for MCF-7 cells, 51.8± 1.2 µg/mL for HepG2 cells, and 14.2± 4.7 µg/mL for HCT 116 cells. Acyclovir-loaded cod liver oil nanoemulsion showed IC50 values of 0.4± 0.02 for MCF-7, 1.5 ± 0.2 for HepG2, and 0.5 ± 0.04 for HCT-116 cells. These results demonstrate the cytotoxic effects of the formulations on the cancer cell lines tested (Figures , , ).
Figure 2. The viability of MCF-7 cells was evaluated after 72 hours of administering varying concentrations (0–100 μg/mL) of Acyclovir solution, cod liver oil nanoemulsion, or Acyclovir-enriched cod liver oil nanoemulsion. The data are shown as mean ± standard deviation.
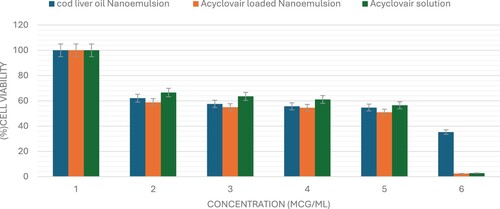
3.6. Cell cycle analysis
The study aimed to investigate the anticancer effects of Acyclovir-loaded cod liver oil nanoemulsion by examining its impact on the cell cycle phases of MCF-7, HepG2, and HCT 116 cancer cells after 48 hours of treatment. Flow cytometry was used to analyze the distribution of cell cycle phases. The results showed that the Acyclovir-loaded cod liver oil nanoemulsion formulation significantly arrested MCF-7 cells in the G2 phase, with a ratio of 38.1% ± 1.9%, and increased the number of HCT 116 cells arrested in the G2 phase by 40.08% ± 1.2%. Similarly, the formulation significantly increased the number of HepG2 cells arrested in the G2 phase, with a 42.2% ± 0.9 ratio compared to the control cells (Figure ).
Figure 5. The impact of Acyclovir-loaded cod liver oil nanoemulsion on the cell cycle distributions of MCF-7, HepG2, and HCT 116 cells was assessed. Cell cycle distribution was analyzed using flow cytometry after 48 hours of treatment. Results are shown as the mean ± SD. Statistical significance is denoted by * for p < 0.05 and ** for p < 0.01
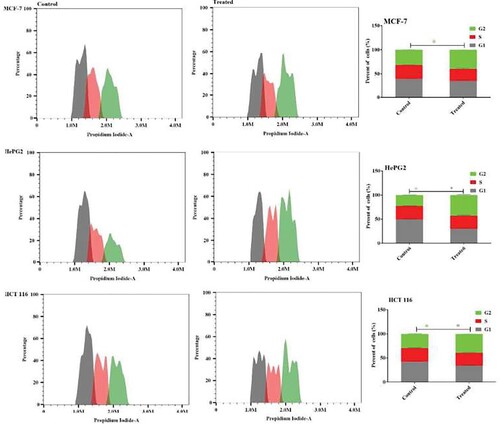
The study’s findings demonstrate the potent anticancer activity of Acyclovir-loaded cod liver oil nanoemulsion, highlighting its ability to induce cell cycle arrest in the G2 phase across various cancer cell lines, including MCF-7, HepG2, and HCT 116, after 48 hours of treatment. This G2 phase arrest suggests that the nanoemulsion disrupts the normal cell cycle progression, potentially preventing cancer cells from dividing and proliferating [Citation25]. The effectiveness of this formulation in inducing cell cycle arrest at a crucial phase of cell division underscores its potential as a therapeutic strategy against cancer, offering a promising avenue for further research and development.
3.7. Apoptosis analysis
To investigate the apoptosis mechanism induced by Acyclovir-loaded cod liver oil nanoemulsion, Annexin V-FITC/PI staining was utilized in MCF-7, HepG2, and HCT 116 cells, followed by flow cytometry analysis. treatment with Acyclovir-loaded cod liver oil nanoemulsion led to a significant increase in apoptotic cells, with a 55.9% ± 1.2 and 75.5% ± 2.1 increase in MCF-7 and HepG2 cells, respectively. The apoptotic effect on HCT 116 colon cancer cells was slightly reduced to 45.4% ± 1.7 after treatment with the acyclovair-loaded cod liver oil Nanoemulsion (Figure ).
Figure 6. Apoptosis induction in MCF-7, HePG2, and HCT 116 cells was assessed after 48 hours of treatment with Acyclovir-loaded cod liver oil nanoemulsion. Dot plots illustrate the distribution of apoptotic cells based on annexin V-FITC/PI staining. Column charts show the quantitative analysis of apoptotic cell percentage following treatment with Metformin-loaded nanoemulsion. Results are expressed as the mean ± SD.
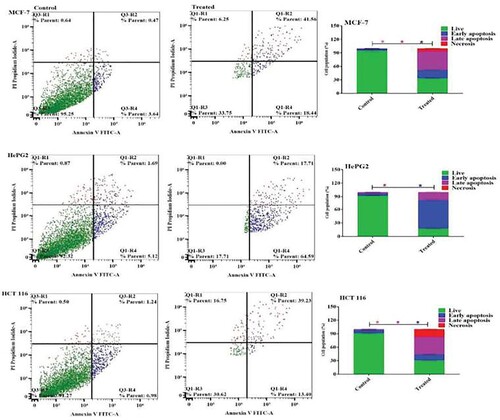
Acyclovir’s anticancer mechanism is hypothesized to involve its ability to interfere with DNA synthesis in cancer cells, similar to its antiviral action. This interference can inhibit cancer cell proliferation, particularly in virus-associated cancers where Acyclovir’s antiviral and anticancer mechanisms may work synergistically [Citation5]. The study’s findings reveal that treatment with Acyclovir-loaded cod liver oil nanoemulsion significantly promotes apoptosis in cancer cell lines, demonstrating its potential as an anticancer agent. Specifically, MCF-7 and HepG2 cells showed substantial increases in apoptosis rates, highlighting the formulation’s effectiveness in inducing programmed cell death in these cell lines. Although the apoptotic effect in HCT 116 cells was slightly lower, it still represents a significant increase compared to untreated controls. The use of Annexin V-FITC/PI staining and flow cytometry provided clear evidence of the nanoemulsion’s capacity to disrupt cell survival pathways, underscoring the therapeutic potential of Acyclovir-loaded cod liver oil nanoemulsion in cancer treatment, particularly by leveraging apoptosis as a mechanism to hinder cancer cell proliferation.
4. Conclusion
The formulation of an Acyclovir-loaded cod liver oil nanoemulsion presents a novel approach to cancer therapy, leveraging the therapeutic potentials of both cod-liver oil and Acyclovir. The nanoemulsion demonstrated favourable physical characteristics, high drug content, and stability, alongside a controlled drug release profile. Notably, the nanoemulsion induced significant cell cycle arrest in the G2 phase across MCF-7, HepG2, and HCT 116 cell lines, alongside a marked increase in apoptosis, indicating its potent anticancer activity. These findings underscore the potential of this innovative nanoemulsion as a strategy for cancer treatment, meriting further investigation and development. The research highlights the importance of drug repurposing and the utilization of natural products in developing more effective cancer therapeutics.
Acknowledgment
This work was also supported by Princess Nourah bint Abdulrahman University researchers supporting project number (PNURSP2024R205), Princess Nourah bint Abdulrahman University, Riyadh, Saudi Arabia.
Disclosure statement
No potential conflict of interest was reported by the author(s).
Data availability statement
The datasets generated for this study are available upon request to the corresponding author.
Additional information
Funding
References
- Huang M, Lu JJ, Ding J. Natural products in cancer therapy: past, present and future. Nat Prod Bioprospect. 2021;11(1):5–13. doi:10.1007/s13659-020-00293-7
- Tojjari A, Choucair K, Sadeghipour A, et al. Anti-inflammatory and immune properties of polyunsaturated fatty acids (PUFAs) and their impact on colorectal cancer (CRC). prevention and treatment. Cancers (Basel). 2023;15(17):4294.
- Mohi-Ud-Din R, Chawla A, Sharma P, et al. Repurposing approved non-oncology drugs for cancer therapy: a comprehensive review of mechanisms, efficacy, and clinical prospects. Eur J Med Res. 2023;28(1):345. doi:10.1186/s40001-023-01275-4
- Nunes P, Santiago PHDO, da Silva CCP, et al. Drug repurposing of the antiviral drug Acyclovir: new pharmaceutical salts. Crystals (Basel). 2023;13(5):782. doi:10.3390/cryst13050782
- Yao J, Zhang Y, Ramishetti S, et al. Turning an antiviral into an anticancer drug: nanoparticle delivery of Acyclovir monophosphate. J Controlled Release. 2013;170(3):414–420. doi:10.1016/j.jconrel.2013.06.009
- Shaimerdenova M, Karapina O, Mektepbayeva D, et al. The effects of antiviral treatment on breast cancer cell line. Infect Agents Cancer. 2017;12:1–10. doi:10.1186/s13027-017-0128-7
- Sánchez-López E, Guerra M, Dias-Ferreira J, et al. Current applications of nanoemulsions in cancer therapeutics. Nanomaterials. 2019;9(6):821. doi:10.3390/nano9060821
- Tayeb HH, Felimban R, Almaghrabi S, et al. Nanoemulsions: formulation, characterization, biological fate, and potential role against COVID-19 and other viral outbreaks. Colloid Interface Sci Commun. 2021;45:100533. doi:10.1016/j.colcom.2021.100533
- Date AA, Nagarsenker MS. Design and evaluation of self-nanoemulsifying drug delivery systems (SNEDDS) for cefpodoxime proxetil. Int J Pharm. 2007;329(1–2):166–172. doi:10.1016/j.ijpharm.2006.08.038
- Gupta S, Kesarla R, Omri A. (2013). Formulation strategies to improve the bioavailability of poorly absorbed drugs with special emphasis on self-emulsifying systems. International Scholarly Research Notices, 2013.
- Alotaibi HF, Khafagy ES, Abu Lila AS, et al. Anticancer potentials of metformin loaded coconut oil nanoemulsion on MCF-7, HepG2 and HCT-116 cell lines. Artif Cells Nanomed Biotechnol. 2023;51(1):419–427. doi:10.1080/21691401.2023.2246145
- Alotaibi HF, Khafagy ES, Alfaifi MY, et al. Cytotoxic potential of clarithromycin-loaded pumpkin seed oil-based nanoemulsion on human breast, hepatic and colorectal cancer cells. Sci Adv Mater. 2023;15(9):1199–1207. doi:10.1166/sam.2023.4523
- Alotaibi HF, Khafagy E-S, Elbehairi SE, et al. Enhancing the anticancer efficacy of ciprofloxacin towards three cancer cell lines via avocado oil nanoemulsion formulation. Int J Pharmacol. 2024;20(1):128–138. doi:10.3923/ijp.2024.128.138
- Loftsson T, Thormar H, Ólafsson JH, et al. Fatty acid extract from cod-liver oil: activity against herpes simplex virus and enhancement of transdermal delivery of Acyclovir in-vitro. Pharmacy Pharmacol Commun. 1998;4(6):287–291.
- Fox LT, Gerber M, Plessis JD, et al. Transdermal drug delivery enhancement by compounds of natural origin. Molecules. 2011;16(12):10507–10540. doi:10.3390/molecules161210507
- Kumar GP, Rajeshwarrao P. Nonionic surfactant vesicular systems for effective drug delivery—an overview. Acta Pharmaceut Sin B. 2011;1(4):208–219. doi:10.1016/j.apsb.2011.09.002
- Li X, Qi J, Xie Y, et al. Nanoemulsions coated with alginate/chitosan as oral insulin delivery systems: preparation, characterization, and hypoglycemic effect in rats. Int J Nanomedicine. 2012;8:23–32.
- Sambhakar S, Malik R, Bhatia S, et al. Nanoemulsion: an emerging novel technology for improving the bioavailability of drugs. Scientifica.. 2023;2023:1–25.
- Danaei MRMM, Dehghankhold M, Ataei S, et al. Impact of particle size and polydispersity index on the clinical applications of lipidic nanocarrier systems. Pharmaceutics. 2018;10(2):57. doi:10.3390/pharmaceutics10020057
- Priya S, Koland M, Kumari S. Nanoemulsion components screening of quetiapine fumarate: effect of surfactant and co surfactant. Asian J Pharm Clin Res. 2015;8(6):136–140.
- Clogston JD, Patri AK. Zeta potential measurement. In: McNeil SE, editor. Characterization of nanoparticles intended for drug delivery, Methods in Molecular biology. Totowa, NJ: Springer; 2011. p. 63–70.
- Patel S, Pandey G, Yadav SK. Review on nano emulsion based drug delivery system. Asian J Pharmaceutical Educ Res. 2018; 7 (2):17–27.
- Mohammadi M, Elahimehr Z, Mahboobian MM. Acyclovir-loaded nanoemulsions: preparation, characterization and irritancy studies for ophthalmic delivery. Curr Eye Res. 2021;46(11):1646–1652. doi:10.1080/02713683.2021.1929328
- Bahri-Najafi R, Mostafavi A, Tavakoli N, et al. Preparation and in vitro-in vivo evaluation of Acyclovir floating tablets. Res Pharm Sci. 2017;12(2):128–136. doi:10.4103/1735-5362.202451
- Park C, Cha HJ, Lee H, et al. Induction of G2/M cell cycle arrest and apoptosis by genistein in human bladder cancer T24 cells through inhibition of the ROS-dependent PI3k/Akt signal transduction pathway. Antioxidants. 2019;8(9):327. doi:10.3390/antiox8090327