ABSTRACT
Recent findings indicate that rainfall variability over West Africa is characterized by more positive anomalies in the last four decades. The authors demonstrate that the recent interannual rainfall variability is linked to an air–sea phenomenon that occurs in the tropical Atlantic and eastern Pacific Ocean, and then propose the Trans-Atlantic-Pacific Ocean Dipole (TAPOD) index as a measure for this tropical ocean phenomenon, which is found to be closely correlated with the West African summer rainfall anomalies. Using observational and reanalysis datasets, composite analysis suggests that enhanced precipitation in West Africa is associated with the positive phase of the TAPOD, which is characterized by warm sea surface temperature anomalies (SSTAs) in the tropical Atlantic and cool SSTAs in the tropical eastern Pacific Ocean. During the positive phase of the TAPOD, there are significant westerly anomalies over the tropical Atlantic Ocean, which drives anomalous water vapor convergence over West Africa, leading to enhanced precipitation in the region.
Graphical Abstract
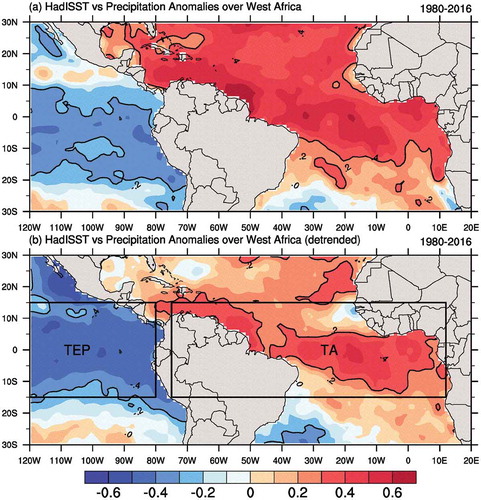
摘要
本研究基于资料统计分析发现西非地区夏季降水与热带大西洋和太平洋海温异常存在紧密的关系;在此基础上提出可表征热带大西洋-太平洋海温异常结构特征的跨大西洋-太平洋偶极型(TAPOD)指数,该指数与西非夏季降水异常存在显著正相关。合成分析表明,当TAPOD指数为正位相时,热带大西洋地区海温为正异常、热带东太平洋海温为负异常,此时热带大西洋地区存在着显著的西风异常,西非地区则存在水汽净幅合异常,有利于该地区夏季降水的异常偏多;反之亦然。
1. Introduction
In the past four decades or so, most locations in West Africa have continued to witness increasing precipitation variability with inundations of unprecedented magnitude. Some notable instances are the 2007, 2010, 2012, and 2017 flood events triggered by heavy precipitation (Paeth et al. Citation2011; Panthou, Vischel, and Lebel Citation2014). Remarkably, the record-breaking sequential rainfall of 2007 prompted disastrous flood events that caused hundreds of deaths and displaced nearly two million people from their homes along with considerable economic losses over sub-Saharan Africa (Paeth et al. Citation2011). Another recent case is the 2017 mudslide and flooding in Sierra Leone. Surging mudslides and floodwaters, triggered by heavy rainfall, killed more than 1100 people and displaced many more from their homes (World Bank Citation2017). The suggestion, therefore, is that this substantial rainfall recovery over West Africa and associated flood events is a reflection of the occurrence of extreme precipitation events being more prevalent over the entire region. Indeed, the magnitude of positive rainfall anomalies has increased, and this is largely homogenous over the entire West Africa (Sanogo et al. Citation2015; Nicholson, Fink, and Funk Citation2018).
The interannual rainfall variability over West Africa is generally modulated by the West African summer monsoon and evolves in a complex manner with notable swings between dry and wet conditions during the summer (June–July–August–September, JJAS) season (Lélé, Leslie, and Lamb Citation2015; Nnamchi and Li Citation2011; Sharon Citation2009). The West African Sahel is particularly notable for the severe drought that plagued the region in the 1970s and the early 1980s (Giannini Citation2015) that extended down to the Guinea Coast. A number of studies have reported the rainfall recovery over the Sahel and noted that the region has slid into wetter conditions with cases of very wet years (Nicholson Citation2005; Sanogo et al. Citation2015; Nicholson, Fink, and Funk Citation2018). Fontaine et al. (Citation2011) demonstrated that the frequency of deep convection has increased, with significant northward migration, since the mid-1990s. Moreover, the well-known rainfall ‘dipole’, with anomalies of opposite sign over the Sahel and Guinea Coast, seems to have disappeared (Losada et al. Citation2012). Stronger interannual rainfall variability and homogenous summer rainfall are now recurrent in the two regions.
It is generally accepted that the interannual precipitation variability in West Africa is influenced by sea surface temperature anomalies (SSTAs) in different ocean basins at different time scales. Sharon (Citation2009) distinguished primary factors that modulate rainfall variation over the regions, including the interhemispheric SST gradient with warmer SSTAs in the North Atlantic and colder SSTAs in the equatorial cold tongue of the Gulf of Guinea, which manifests itself as an anomalous quasi-symmetrical structure in the tropical Atlantic Ocean (Li Citation2001; Chiang and Vimont Citation2004). Moreover, domain-wide positive precipitation anomalies over West Africa are strongly linked with the interhemispheric positive SSTA in the tropical Atlantic. Relatively, El Niño events are mainly associated with larger east–west divergent circulation over the tropical Atlantic, which coincides with negative precipitation anomalies over West Africa, as in the case of composite El Niño episodes (Janicot et al. Citation1998). Meanwhile, La Liña events are closely linked with positive precipitation anomalies over West Africa (Paeth et al. Citation2011).
The interbasin connection between the equatorial eastern Pacific SST and tropical Atlantic SST variability has been the subject of many studies (Dippe, Greatbatch, and Ding Citation2017; Kajtar et al. Citation2016; Kucharski et al. Citation2016). These studies have demonstrated how the warm SSTAs over the Atlantic influence the dynamical processes that trigger the development of the Pacific La Niña, and vice versa. Li et al. (Citation2015) demonstrated that warm SSTAs over the Atlantic drive anomalous wind dynamics that induces a La Niña–type response in the tropical eastern Pacific. This shows that SSTAs in the tropical Atlantic are connected to the La Niña–like response over the tropical eastern Pacific, and seems to have been dominant in the last four decades. Moreover, Paeth et al. (Citation2011) observed that the combined effect of warm SSTAs in the tropical Atlantic Ocean and La Niña episodes in the tropical Pacific favors stronger than normal precipitation over sub-Saharan Africa. This is the case for the 2007 flood event over sub-Saharan Africa.
In this paper, we explore the inherent features of the SSTA pattern in the tropical Atlantic and Pacific Ocean basins, and further investigate the combined impact of the dipole-like SSTA structure on precipitation variability over West Africa, and the associated circulation features.
2. Data
Climate Research Unit (CRU) (New, Hulme, and Jones Citation2000; Mitchell and Jones Citation2005) monthly precipitation data, with a horizontal resolution of 0.5° × 0.5°, are applied. To evaluate the connection between sea surface temperature (SST) and precipitation anomalies, we utilize Hadley Centre Sea Ice and Sea Surface Temperature data, on 1° × 1° grids, based on EOF reconstruction of observations (Rayner et al. Citation2003). The atmospheric moisture circulation is explored with six-hourly NCEP reanalysis zonal and meridional wind fields, surface pressure, and specific humidity (Kalnay et al. Citation1996), with a spatial resolution of 2.5° × 2.5° resolution. The daily means are averaged to monthly means, and JJAS is selected for further analysis. The West African region in this study covers (4°–20°N, 20°W–20°E).
3. Trans-Atlantic-Pacific Ocean Dipole index and its relationship with West African summer precipitation anomalies
To investigate the possible mechanism responsible for the interannual variation of summer precipitation over West Africa, we first calculate the correlation coefficient between the precipitation anomalies over the entire West African region and the SSTAs in the tropical eastern Pacific Ocean and the Atlantic Ocean. ) shows the correlation spatial patterns between interannual JJAS precipitation variability over West Africa and the SSTA over the Trans-Atlantic-Pacific Ocean (TAPO) sector during 1980–2013. The correlation shows a distinctive pattern over the oceans basins, with significantly positive correlation coefficients over the tropical Atlantic and negative values over the eastern Pacific Ocean.
Figure 1. (a) Correlation between anomalous CRU precipitation anomalies over West Africa (5°–20°N, 20°W–15°E) during JJAS and SSTAs (1980–2013). (b) As in (a) but with detrended anomalies. The black contours indicate regions significant at the 95% confidence level, based on a t-test. The frames show the location of the tropical eastern Pacific (TEP: 15°S–15°N, 120°–80°W) and tropical Atlantic (TA: 15°N–12°S, 75°W–10°E) used for defining the TAPODI.
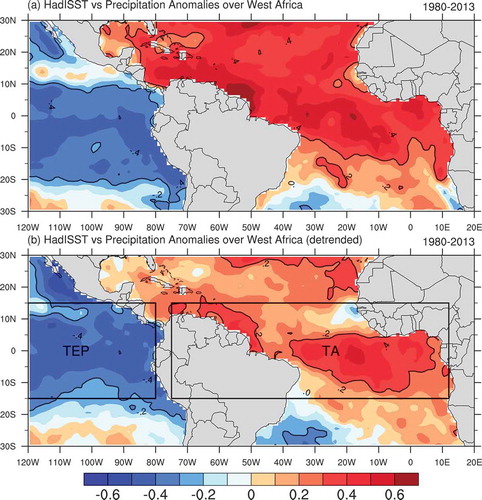
Previous studies have suggested that a significant warming trend in the Atlantic Ocean in recent decades (Li et al. Citation2015). We recalculate the correlation between West African summer rainfall (WASR) anomalies with the SSTA over the TAPO region, but with detrended SSTA and precipitation anomalies (as shown in )), the intention of which is to remove the impact of the warming trend of the Atlantic Ocean on the rainfall–SSTA relationship. A significant SSTA–WASR anomaly relationship can still be found in the tropical Atlantic Ocean and eastern Pacific Ocean, as shown in ), even though the high-correlation regions are smaller than in the un-detrended case.
Meanwhile, significant negative correlation can be found in the tropical eastern Pacific and significant positive correlation in the tropical Atlantic, suggesting the WASR is closely related with the dipole-like SSTA structure in the TAPO basin, i.e., the SSTA in the tropical Atlantic and tropical eastern Pacific domain evolves in an opposite fashion. The dipolar structure in the two ocean domains is in line with the phenomena described in Mcgregor et al. (Citation2014), Martín-Rey et al. (Citation2014) and Li et al. (Citation2015).
3.1. TAPOD index and its relationship with summer precipitation variability over West Africa
In order to measure the dipole-like SSTAs in the tropical eastern Pacific and Atlantic, the TAPOD Index (TAPODI) is proposed by differencing the domain-averaged normalized SSTA of the two regions with high correlation with the West African summer rainfall anomalies, which could be used for investigating the strength and connections of TAPOD events to larger-scale climate variability:
where the square brackets indicate domain averages, and the subscripts show the two regions over which the SSTA averages are computed. The two domains are described by their locations in the tropical Atlantic (i.e., TA) and tropical eastern Pacific (i.e., TEP) as the Atlantic pole (TA: 15°N–15°S, 75°W–10°E) and the Pacific pole (TEP: 15°S–15°N, 120°–80°W), as delineated in ).
Based on Equation (1), we derive a measure of the TAPOD. ) elucidates the interannual variability (time series) of this tropical ocean dipole index. Furthermore, the figure highlights that the SSTA over the tropical Atlantic and the tropical eastern Pacific is characterized by strong interannual variability, even though the warming and cooling trends are also found in the ocean domains. The time series of the normalized WASR anomaly is also presented in ), and it is found that the TAPODI and WASR anomaly are related with a correlation coefficient of r = 0.58. This suggests that the TAPODI is significantly correlated with domain-wide precipitation anomalies over West Africa during 1980–2013, and can be regarded as an efficient index for predicting the interannual variability of the WASR.
Figure 2. (a) Interannual variability of the TAPODI, tropical Atlantic, tropical eastern Pacific, and precipitation over West Africa (dotted line represents the trend line). The correlation coefficients between West African precipitation anomalies and the TAPODI, tropical Atlantic and tropical eastern Pacific are 0.58, 0.57, and −0.34, respectively. Composite precipitation anomalies based on the TAPODI for (b) positive TAPOD (wet) years and (c) negative TAPOD (dry) years, based on CRU precipitation.
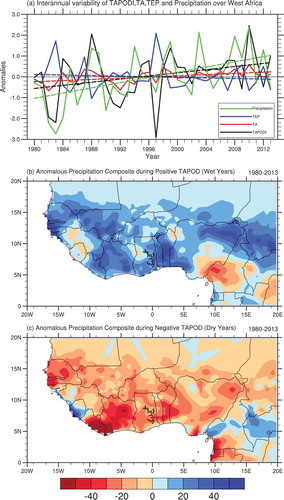
To further illustrate the relationship between the TAPODI and WASR, ,c) present the composite summer rainfall anomalies over West Africa for the positive and negative phase of the TAPOD, respectively. We compute the composites of precipitation based on typical cases of positive and negative TAPODI, defined as the ±0.5 standard deviation of the TAPODI series. This definition yields positive TAPODI years of 1988, 1989, 1995, 1996, 1999, 2003, 2007, 2008, 2010, 2011, and 2013, and negative years of 1982, 1983, 1991, 1992, 1993, 1994, 1997, and 2009, during 1980–2013. The figure shows that there is precipitation enhancement over West Africa during the positive phase of the TAPODI, vice versa for the negative phase of the dipole. The same composite rainfall pattern is reproduced using CRU and GPCC data (figure for GPCC not shown), suggesting the observed pattern is robust. ) shows that there is precipitation enhancement over West Africa, with a larger magnitude around 4°–18°N. Moreover, the Guinea coast, west coast, around Mauritania, Senegal, and its environs, are characterized by enhanced precipitation.
Similarly, the eastern extremity extending to the Nigerian Sahel and the Lake Chad basin experiences greater than normal precipitation during the positive phase of the TAPOD, and this enhanced precipitation is expected to increase more in the future (Dike et al. Citation2015). Conversely, during the negative phase of the TAPOD, the whole of West Africa is characterized by deficient precipitation, as shown in ), except for a small part of Sierra Leone and southern Cameroon.
To gain dynamical insights into how the TAPOD could induce West African precipitation anomalies, we also examine the atmospheric circulation and vertically integrated water vapor convergence associated with the phenomenon. Using NCEP reanalysis data, the anomalous horizontal wind field is firstly given in . The data illustrate the composite wind anomalies at 850 hPa for positive and negative TAPODI years. Strong westerly anomalies can be found throughout the tropical Atlantic Ocean, with maximum anomalies centered over 0°–10°N, for positive TAPODI years ()). As revealed by many previous studies, moisture transport by westerlies from the Atlantic Ocean to West Africa is the main moisture supply for the West African rainfall (Kidson Citation1977; Pu and Cook Citation2012), so the composite westerly anomaly will lead to very large quantities of moisture transport from the ocean to the Caribbean and West Africa and, hence, enhanced rainfall over West Africa. Undoubtedly, low-level westerly jets plays an important role in moisture transport (Lélé, Leslie, and Lamb Citation2015; Pu and Cook Citation2012). Meanwhile, ) shows converse characteristics during the negative phase of the TAPOD, showing a weakening of the westerlies. This is consistent with the fact that westerly moisture transport has a significant influence on the summer precipitation variability over West Africa.
Figure 3. Composites of horizontal wind field based on the TAPODI anomalies in the tropical Atlantic–Pacific Ocean basin at 850 hPa for (a) positive TAPOD years and (b) negative TAPOD years, based on NCEP reanalysis data (shading indicates the zonal component of wind anomalies).
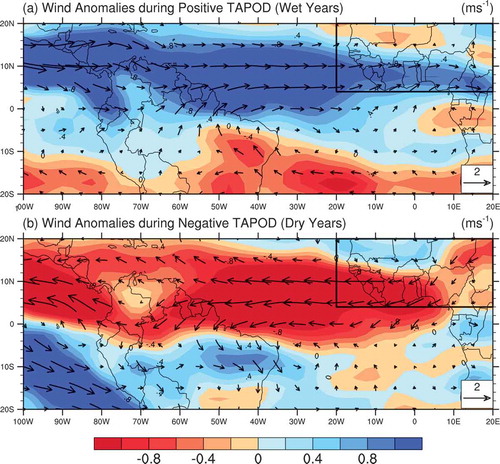
The total horizontal moisture flux components (zonal and meridional) are calculated by the vertically integrated water vapor flux for the positive and negative TAPODI years, as shown in . The total pressure column extends from the surface (1000 hPa) to 300 hPa. The positive TAPODI composites are characterized by strong moisture convergence to the Guinea Coast and the Sahel ()), and indeed the entire region. This results in the observed enhanced large-scale and homogeneous precipitation over West Africa. ) shows a converse pattern during negative TAPODI years. ) clearly demonstrates that the westerly anomalies are strengthened during the positive phase of the TAPOD. Moreover, Pu and Cook (Citation2010), Pu and Cook (Citation2012) described the westerly anomalies, as shown in ), as West African westerly jets and linked the jets with anomalous precipitation over the West African Sahel.
Figure 4. Composites of vertically integrated moisture flux based on (a) positive and (b) negative TAPODI anomalies, in which blue (red) indicates convergence (divergence), based on NCEP reanalysis data.
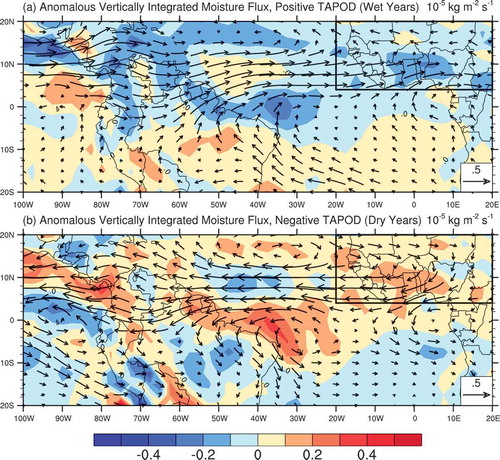
However, we hypothesize that the westerlies perhaps originate over the equatorial eastern Pacific due to the temperature gradient between the two ocean basins. These characteristic moisture dynamics may hold the key to understanding the recent spate of extreme precipitation over West Africa. This point is further strengthened by the correlation between the index and precipitation anomalies, as illustrated in ). This elucidates the influence of the TAPOD on West African precipitation.
4. Summary and discussion
In this study we evaluate the existence of a Trans-Atlantic-Pacific-Ocean Dipole and its possible impact on precipitation anomalies over West Africa. The correlations of the derived TAPODI with precipitation anomalies over West Africa suggest a strong relationship between the observed SSTA structure and precipitation anomalies over West Africa. Moreover, the West African Summer Monsoon Index (Li and Zeng Citation2003) is well correlated with the TAPODI and precipitation anomalies over West Africa (Nnamchi and Li Citation2011). This perhaps indicates that a significant proportion of the monsoon system is modulated by the TAPOD, which suggests enhanced (weakened) monsoonal flow during positive (negative) phases of the TAPOD. Nonetheless, this demonstrates that, during a positive TAPOD, the West African monsoon will be stronger, corresponding to the greater than normal rainfall over West Africa. Additionally, the Niño3.4 index is also known to be correlated with summer precipitation over West Africa. However, the TAPODI shows a stronger correlation with precipitation anomalies in the region. This suggests that the TAPODI could possibly be regarded as an indicator for the prediction of West African summer rainfall anomalies. During the positive phase of the dipole, the SST gradient between the two ocean basins drives anomalous westerly anomalies that are linked to water vapor convergence and greater than normal precipitation over West Africa. On the other hand, the negative phase of the dipole is characterized by divergent water vapor flux, with deficient precipitation over the region. At this point, one question that may arise is the apparent asymmetrical nature of the wind anomalies related to the TAPOD at the equator. It is imperative to note that the positive/negative phases of the TAPOD are usually accompanied by SSTA signals outside the tropical Atlantic–Pacific Ocean domain, especially in the tropical North Atlantic sector, which is not symmetrical at the equator. This could possibly explain why the wind anomalies related to the TAPOD are asymmetrical at the equator.
Furthermore, numerical experiments using a global climate system model are needed for verifying the mechanism proposed in this study, as well as to isolate the impact of the TAPOD from those induced by SSTAs in other parts of the ocean basins, and to further distinguish the relative importance of SSTAs in the tropical eastern Pacific and the Atlantic on West African summer rainfall.
Disclosure statement
No potential conflict of interest was reported by the authors.
Additional information
Funding
References
- Bank, W. 2017. “Sierra Leone Rapid Damage and Loss Assessment of August 14th, 2017 - Landslides and Floods in the Western Area.” https://reliefweb.int/sites/reliefweb.int/files/resources/19371_Sierra_Leone_DaLA_Web-forprinting.pdf
- Chiang, J. C. H., and D. J. Vimont. 2004. “Analogous Pacific and Atlantic Meridional Modes of Tropical Atmosphere-Ocean Variability.” Journal of Climate 17 (21): 4143–4158. doi:10.1175/JCLI4953.1.
- Dike, V. N., M. H. Shimizu, M. Diallo, Z. H. Lin, O. K. Nwofor, and T. C. Chineke. 2015. “Modeling Present and Future African Climate Using CMIP5 Scenarios in HadGEM2-ES.” International Journal of Climatology 35 (8): 1784–1799. doi:10.1002/joc.4084.
- Dippe, T., R. J. Greatbatch, and H. Ding. 2017. “On the Relationship between Atlantic Niño Variability and Ocean Dynamics.” Climate Dynamics 21: 1–16.
- Fontaine, B., P. Roucou, M. Gaetani, and R. Marteau. 2011. “Recent Changes in Precipitation, ITCZ Convection and Northern Tropical Circulation over North Africa (1979–2007).” International Journal of Climatology 31 (5): 633–648. doi:10.1002/joc.v31.5.
- Giannini, A. 2015. “Climate Change Comes to the Sahel.” Nature Climate Change 5 (8): 720–721. doi:10.1038/nclimate2739.
- Janicot, S., A. Harzallah, B. Fontaine, and V. Moron. 1998. “West African Monsoon Dynamics and Eastern Equatorial Atlantic and Pacific SSTA (1970–88.” Journal of Climate 11: 1874–1882.
- Kajtar, J. B., A. Santoso, M. H. England, and W. Cai. 2016. “Tropical Climate Variability: Interactions across the Pacific, Indian, and Atlantic Oceans.” Climate Dynamics 48 (7–8): 1–18.
- Kalnay, E., M. Kanamitsu, R. Kistler, W. Collins, D. Deaven, L. Gandin, M. Iredell et al. 1996. “The NCEP/NCAR 40-Year Reanalysis Project.” Bulletin of the American Meteorological Society 77 (3): 437–472. DOI:10.1175/1520-0477(1996)077<0437:TNYRP>2.0.CO;2.
- Kidson, J. W. 1977. “African Rainfall and Its Relation to the Upper Air Circulation.” Quarterly Journal of the Royal Meteorological Society 103: 441–456. doi:10.1002/qj.49710343705.
- Kucharski, F., A. Parvin, M. B. Rodríguez Fonseca, R. Farneti, M. Martín Rey, I. P. Sánchez, E. M. Harris, T. D. Losada, and C. R. Mechoso. 2016. “The Teleconnection of the Tropical Atlantic to Indo-Pacific Sea Surface Temperatures on Inter-Annual to Centennial Time Scales: A Review of Recent Findings.” Atmosphere 7 (2): 29. doi:10.3390/atmos7020029.
- Lélé, M. I., L. M. Leslie, and P. J. Lamb. 2015. “Analysis of Low-Level Atmospheric Moisture Transport Associated with the West African Monsoon.” Journal of Climate 28 (11): 150311135632008.
- Li, J., and Q. Zeng. 2003. “A New Monsoon Index and the Geographical Distribution of the Global Monsoons.” Advances in Atmospheric Science 20: 299–302. doi:10.1007/s00376-003-0016-5.
- Li, X., S. P. Xie, S. T. Gille, and C. Yoo. 2015. “Atlantic-Induced Pan-Tropical Climate Change over the past Three Decades.” Nature Climate Change 6 (3): 275–279. doi:10.1038/nclimate2840.
- Li, Z. X. 2001. “Thermodynamic Air-Sea Interactions and Tropical Atlantic SST Dipole Pattern.” Physics & Chemistry of the Earth Particle B Hydrology Oceans & Atmosphere 26 (2): 155–157. doi:10.1016/S1464-1909(00)00233-1.
- Losada, T., B. Rodriguez-Fonseca, E. Mohino, J. Bader, S. Janicot, and C. R. Mechoso. 2012. “Tropical SST and Sahel Rainfall: A Non-Stationary Relationship.” Geophysical. Research. Letters 39: L12705. doi:10.1029/2012GL052423.
- Martín-Rey, M., B. Rodríguez-Fonseca, I. Polo, and F. Kucharski. 2014. “On the Atlantic–Pacific Niños Connection: A Multidecadal Modulated Mode.” Climate Dynamics 43 (11): 3163–3178. doi:10.1007/s00382-014-2305-3.
- Mcgregor, S., A. Timmermann, M. F. Stuecker, M. H. England, M. Merrifield, F. F. Jin, and Y. Chikamoto. 2014. “Recent Walker Circulation Strengthening and Pacific Cooling Amplified by Atlantic Warming.” Nature Climate Change 4 (10): 888–892. doi:10.1038/nclimate2330.
- Mitchell, T. D., and P. D. Jones. 2005. “An Improved Method of Constructing a Database of Monthly Climate Observations and Associated High-Resolution Grids.” International Journal of Climatology 25 (6): 693–712. doi:10.1002/(ISSN)1097-0088.
- New, M., M. Hulme, and P. Jones. 2000. “Representing Twentieth-Century Space-Time Climate Variability. Part II: Development of 1901–96 Monthly Grids of Terrestrial Surface Climate.” Journal of Climate 12 (12): 829–856. doi:10.1175/1520-0442(1999)012<0829:RTCSTC>2.0.CO;2.
- Nicholson, S. E. 2005. “On the Question of the “Recovery” of the Rains in the West African Sahel.” Journal of Arid Environments 63 (3): 615–641. doi:10.1016/j.jaridenv.2005.03.004.
- Nicholson, S. E., A. H. Fink, and C. Funk. 2018. “Assessing Recovery and Change in West Africa’s Rainfall Regime from a 161‐Year Record..” International Journal of Climatology 38: 3770–3786. doi:10.1002/joc.2018.38.issue-10.
- Nnamchi, H. C., and J. P. Li. 2011. “Influence of the South Atlantic Ocean Dipole on West African Summer Precipitation.” Journal of Climate 24: 1184–1197. doi:10.1175/2010JCLI3668.1.
- Paeth, H., A. H. Fink, S. Pohle, F. Keis, H. Mächel, and C. Samimi. 2011. “Meteorological Characteristics and Potential Causes of the 2007 Flood in sub-Saharan Africa.” International Journal of Climatology 31 (13): 1908–1926. doi:10.1002/joc.2199.
- Panthou, G., T. Vischel, and T. Lebel. 2014. “Recent Trends in the Regime of Extreme Rainfall in the Central Sahel.” International Journal of Climatology 34 (15): 3998–4006. doi:10.1002/joc.2014.34.issue-15.
- Pu, B., and K. H. Cook. 2010. “Dynamics of the West African Westerly Jet.” Journal of Climate 23 (23): 6263–6276. doi:10.1175/2010JCLI3648.1.
- Pu, B., and K. H. Cook. 2012. “Role of the West African Westerly Jet in Sahel Rainfall Variations.” Journal of Climate 25 (8): 2880–2896. doi:10.1175/JCLI-D-11-00394.1.
- Rayner, N. A., D. E. Parker, E. B. Horton, C. K. Folland, L. V. Alexander, D. P. Rowell, E. C. Kent, and A. Kaplan. 2003. “Global Analyses of Sea Surface Temperature, Sea Ice, and Night Marine Air Temperature since the Late Nineteenth Century.” Journal Geophysical Research 108 (D14): 4407.
- Sanogo, S., A. H. Fink, A. O. Jerome, A. Ba, R. Redl, and V. Ermert. 2015. “Spatio-Temporal Characteristics of the Recent Rainfall Recovery in West Africa.” International Journal of Climatology 35 (15): 4589–4605. doi:10.1002/joc.4309.
- Sharon, N. E. 2009. “On the Factors Modulating the Intensity of the Tropical Rain Belt over West Africa.” International Journal of Climatology 29: 673–689. doi:10.1002/joc.1702.