ABSTRACT
This study investigates the combined effect of the El Niño–Southern Oscillation (ENSO) and stratospheric quasi-biennial oscillation (QBO) on the Madden Julian Oscillation (MJO). The results show that the western Pacific MJO originating from the Indian Ocean during La Niña/QBO easterly years is stronger than that during El Niño years. This relation, however, disappears during La Niña/QBO westerly years. The reason is that ENSO and the QBO have different effects on each MJO event. For an El Niño year, there is only about one MJO event, and the QBO effect is small. During a La Niña/QBO easterly year, there are 1.7 MJO events, while during a La Niña/QBO westerly year, there are only 0.6 MJO events. El Niño can reinforce the MJO over the western Pacific because of the positive moisture advection of the El Niño mean state by MJO easterly wind anomalies. The QBO mainly affects the MJO over the Maritime Continent region by changing the high-cloud-controlled diurnal cycle; and the Maritime Continent barrier effect is enhanced during the QBO westerly phase because of the strong diurnal cycle. During El Niño years, even the MJO over the Maritime Continent is suppressed by the QBO westerly phase; the MJO can be reinforced over the western Pacific. During La Niña/QBO westerly years, the MJO over the Maritime Continent is suppressed because of the strong Maritime Continent diurnal cycle, and it is further suppressed over the western Pacific because of the lack of a reinforcement process.
Graphical abstract
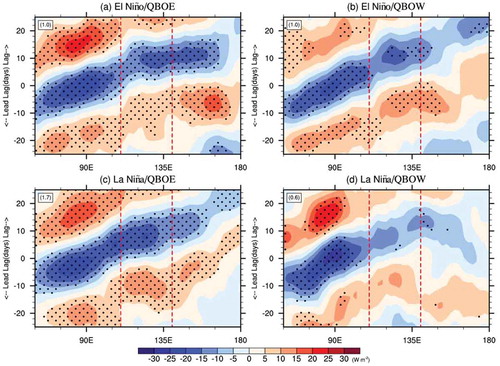
摘要
本文研究了平流层准两年振荡与ENSO对MJO的协同作用。结果显示:在拉尼娜/准两年振荡东风位相,起源于印度洋到达西太平洋的MJO要比在厄尔尼诺年强。但是在拉尼娜/准两年振荡西风位相,这种关系不复存在。原因在于ENSO和准两年振荡对MJO事件的影响不同。厄尔尼诺年西太平洋地区正水汽平流有利于MJO加强,而准两年振荡西风位相下海洋性大陆地区较强的日循环会使得海洋性大陆屏障作用增强。在厄尔尼诺年,尽管MJO在准两年振荡西风位相下会受到抑制,但是在到达西太平洋是都能得到加强。在拉尼娜/准双周振荡的西风位相下,海洋性大陆较强日循环和西太平洋不利的背景条件都会抑制MJO。
1. Introduction
Understanding the interannual variability of the propagation of the Madden–Julian Oscillation (MJO) is important for improving subseasonal predictability. The MJO is a dominant intraseasonal variability with a period of 30–60 days in the tropical atmosphere (Madden and Julian Citation1971). Often starting in the equatorial Indian Ocean, the MJO moves eastward with a slow phase speed of 5 m s−1. The convection of the MJO can cross the Maritime Continent and ends in the central Pacific. The circulation associated with the MJO, however, can propagate eastward globally (Zhang Citation2005).
The stratospheric quasi-biennial oscillation (QBO) and the El Niño–Southern Oscillation (ENSO) are two dominant interannual variabilities in the tropics. The QBO is denoted by the reversal of 10–100-hPa equatorial zonal winds between westerly and easterly every 28 months, approximately (Baldwin et al. Citation2001), while ENSO is associated with anomalously warm (El Niño) and cold (La Niña) conditions in the tropical Pacific with a period of 2–8 years (Deser et al. Citation2010). Recent studies have shown that the strength and predictability of the MJO may be modulated by the QBO (Pang et al. Citation2016; Yoo and Son Citation2016; Son et al. Citation2016). The tropical deep convection during the QBO easterly phase (QBOE) has been found to be much stronger than that during the westerly phase (QBOW), because of different tropopause height, temperature, vertical wind shear, and associated static stability (Collimore et al. Citation2003). Compared to the QBOW, the tropopause of the QBOE is higher and colder, and is associated with ascending motion; plus, its vertical zonal wind shear is much weaker across the tropopause, resulting in lower static stability of the upper troposphere (Baldwin et al. Citation2001). Thus, the activities of the MJO are significantly stronger in the QBOE than the QBOW; and this difference only exists during boreal winter (Son et al. Citation2017; Hood Citation2017; Nishimoto and Yoden Citation2017). The MJO’s propagation appears to be slower and lasts longer during the QBOE than the QBOW (Nishimoto and Yoden Citation2017). The QBO-related change in the MJO can explain up to 40% of the interannual variation of the boreal-winter MJO amplitude (Marshall et al. Citation2016). The fluctuation in MJO strength between the two QBO phases, i.e. a stronger MJO during the QBOE than QBOW, has also been found to be amplified by the minimum and maximum of the 11-year solar cycle (Hood Citation2017). The most recent work shows that the stronger activities of the MJO in the QBOE are a consequence of there being more MJO days, as opposed to a larger amplitude of individual MJO events, as previously thought (Zhang and Zhang Citation2018).
The overall amplitude of MJO intensity has been found to be uncorrelated with sea surface temperature (SST) anomalies (Salby and Hendon Citation1994; Hendon, Zhang, and Glick Citation1999; Lawrence and Webster Citation2010), and the overall level of MJO activity across the Maritime Continent does not change much between El Niño and La Niña (Hendon, Zhang, and Glick Citation1999; Gualdi, Navarra, and Tinarelli Citation1999; Gushchina and Dewitte Citation2012). Model calculations also show very little interannual predictability of MJO intensity (Waliser et al. Citation2010). The MJO’s convection, however, is location dependent. Compared to El Niño years, the seasonal mean tropical convection over the Maritime Continent in La Niña winters is strengthened, while that over the central and eastern Pacific is weakened, because of the enhanced Walker circulation in response to a strengthened east–west SST gradient across the tropical Pacific (Son et al. Citation2017). The convection of the MJO is usually confined to the west of the dateline during La Niña years (Salby and Hendon Citation1994). The MJO’s strength in the western Pacific is correlated with the eastern extension of the Warm Pool’s eastern edge (Kessler Citation2001; Liess, Bengtsson, and Arpe Citation2004); and during warm ENSO phases, the convection of the MJO can penetrate further into the central Pacific (Gualdi, Navarra, and Tinarelli Citation1999; Moon, Wang, and Ha Citation2011; Liu et al. Citation2016a, Citation2016b). The intensity of the MJO from the tropical eastern Indian Ocean to the western Pacific is weakened during eastern-Pacific El Niño winters, but enhanced during central-Pacific El Niño winters (Feng et al. Citation2014; Pang et al. Citation2016).
The combined effect of the QBO and ENSO on the MJO, however, is not clear. Our results will show that the QBO effect on the MJO found by Zhang and Zhang (Citation2018), i.e. more MJO events during the QBOE than QBOW, mainly happens during La Niña phases than El Niño phases.
The paper is organized as follows: In section 2, we present the data and methods. The combined effect of the QBO and ENSO on the MJO and the possible mechanisms are explored in section 3. Conclusions and discussion are given in section 4.
2. Data and methods
In this work, the NOAA’s interpolated outgoing longwave radiation (OLR) data from 1979 to 2016 (Liebmann and Smith Citation1996) are used to select the MJO events during boreal winter (November to April). MJO events originating from the Indian Ocean are defined by the equatorial Indian Ocean (15°S–15°N, 75°–95°E) 20–70-day bandpass-filtered OLR anomaly being smaller than 1 standard deviation. Individual MJO events are also identified by using the tracking method developed by Ling et al. (Citation2014) and updated by Zhang and Zhang (Citation2018). First, the 20–70-day bandpass filter is used on the tropical (15°S–15°N average) OLR to obtain the intraseasonal signal; then, the MJO events are defined by three criteria from the lead–lag regression map of OLR on the equatorial Indian Ocean OLR, i.e. a slow eastward propagation speed of 3–7 m s−1, a long propagation range larger than 50° longitude, and an intraseasonal time scale longer than 20 days (Zhang and Ling Citation2017). Other bandpass filters, such as 30–90 and 20–100 days, were also used to obtain intraseasonal signals, and similar results were obtained (not shown).
The QBO index is defined by the mean zonal wind at 50 hPa averaged over 10°S–10°N. A QBOE (QBOW) phase is defined when this QBO index is smaller (larger) than 0. Monthly mean winds are obtained from the ERA-Interim dataset (Dee et al. Citation2011). During the 38 years from 1979 to 2016, there were 22 QBOE phase years and 15 QBOW phase years. The Niño3.4 index, based on the SST anomaly averaged within (5°S–5°N, 170°–120°W), is used to define ENSO. An El Niño phase is defined by positive boreal-winter Niño3.4 index, and a La Niña one by negative index. The monthly mean SST data are from the Hadley Centre dataset (Rayner et al. Citation2003). For these 38 years, there were 17 El Niño phases and 19 La Niña phases. Other thresholds defining the ENSO event were also used, and similar results were obtained (not shown).
The monthly-mean vertical pressure velocity, high-cloud coverage, and lower-tropospheric humidity are from ERA-Interim (Dee et al. Citation2011). The three-hourly precipitation from the Tropical Rainfall Measuring Mission 3B42v7 Multisatellite Precipitation Analysis (Huffman et al. Citation1997) is used to calculate the strength of the diurnal cycle over the Maritime Continent, which is defined by the maximum minus minimum precipitation on each day.
3. Results
3.1 Evolution of the MJO during different phases
To explore the effect of the QBO and ENSO on the MJO, the relationship between the QBO and ENSO is investigated first. But, no significant relationship is found, and the correlation coefficient between these two indexes for the period 1979–2016 is only −0.014. The past 38 years can be classified into four phases, i.e. El Niño/QBOW, El Niño/QBOE, La Niña/QBOE, and La Niña/QBOW, which cover 7 (1982, 1987, 1990, 1992, 1994, 2006, 2015), 10 (1979, 1986, 1989, 1991, 1993, 1997, 2002, 2003, 2004, 2009), 11 (1981, 1983, 1984, 1988, 1995, 1996, 2000, 2005, 2007, 2011, 2012), and 8 (1980, 1985, 1998, 1999, 2001, 2008, 2010, 2013) years, respectively.
First, we investigate whether the QBO and ENSO can affect the MJO’s propagation. During these four phases, the MJOs that originate from the equatorial Indian Ocean show different evolutions (). During El Niño winters, significant MJO convection can cross the Maritime Continent and reach the western Pacific (,), under either the QBOE or QBOW. During La Niña, however, the QBO has different effects on the MJO’s evolution. Significant MJO signals can reach the western Pacific under the QBOE ()), whereas it remains in the Indian Ocean and cannot pass the Maritime Continent to affect the western Pacific under the QBOW ()). The MJO’s strength over the equatorial Indian Ocean during La Niña/QBOW phases, however, is comparable to that during La Niña/QBOE, which means that the strength of the initial MJO is not the reason controlling whether the MJO can pass the Maritime Continent. In summary, the QBO only affects the MJO’s eastward propagation during La Niña years, and MJO signals originating from the Indian Ocean cannot reach the western Pacific during La Niña/QBOW phases.
Figure 1. Evolution of MJO signals originating from the equatorial Indian Ocean in (a) El Niño/QBOE, (b) El Niño/QBOW, (c) La Niña/QBOE, and (d) La Niña/QBOW phases. The patterns are shown by longitude–time-lagged diagrams of boreal-winter equatorial (15°S–15°N) OLR anomalies (contours; units: W m−2) regressed on the equatorial Indian Ocean (15°S–15°N, 75°-95°E) OLR anomaly during 1979–2016. Dots denote anomalies significant at the 0.05 level. The red dashed lines denote the longitudinal range of the Maritime Continent (110°–140°E).
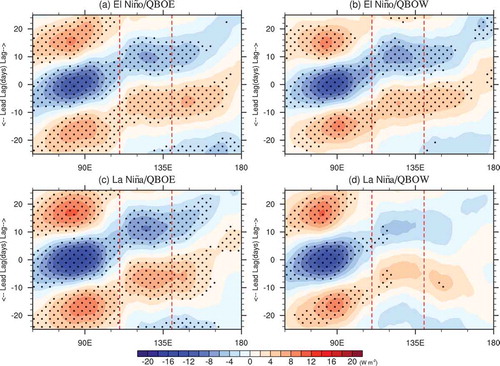
3.2 Change in MJO events caused by ENSO and the QBO
,) show how ENSO can affect the MJO by tracking each MJO event formed west of the Maritime Continent. For each El Niño year, there is one MJO that can pass the Maritime Continent. Along with its eastward propagation, the MJO is suppressed over the Maritime Continent and becomes reinforced over the western Pacific (Liu and Wang Citation2016). During a La Niña year, there are 1.3 MJO events. The MJO’s strength over the Indo-Maritime Continent region during a La Niña year is stronger than that during an El Niño year, whereas there is a stronger MJO over the western to central Pacific during an El Niño year than during a La Niña year.
Figure 2. MJO propagation patterns along the equator during (a) El Niño, (b) La Niña, and (c) QBOW and (d) QBOE phases. The patterns are shown by composites of OLR anomalies (contours; units: W m−2) for tracked boreal-winter MJO events formed west of the Maritime Continent (40°–100°E) during 1979–2016. Dots denote anomalies significant at the 0.05 level. The number in each panel denotes the number of MJO events per year during each phase. The red dashed lines denote the longitudinal range of the Maritime Continent (110°–140°E).
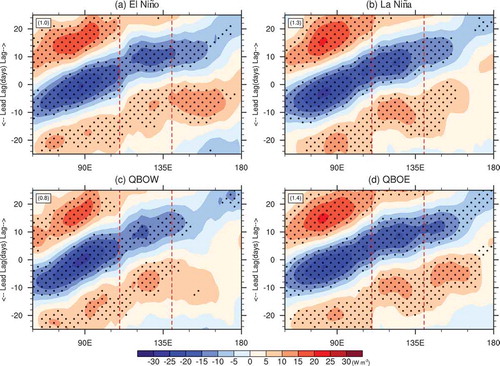
,) exhibit the effect of the QBO on the MJO. For each QBOE year, there are 1.4 MJO events. This occurrence frequency of the MJO is much higher than the 0.8 per QBOW year. The MJO’s intensity from the Maritime Continent to the western Pacific during a QBOE year is also stronger than that during a QBOW year. These QBO effects, i.e. more frequent MJO events and stronger MJO intensity from the Maritime Continent to the western Pacific, were first observed by Zhang and Zhang (Citation2018).
In summary, La Niña can slightly increase the number of MJOs able to pass the Maritime Continent, whereas El Niño can reinforce the MJO over the western Pacific. The QBOE tends to increase the number of MJOs passing the Maritime Continent and enhance the MJO intensity from the Maritime Continent to the western Pacific.
3.3 Change in MJO events caused by ENSO and the QBO combined
Since El Niño can reinforce the MJO after passing over the Maritime Continent and the QBOE can increase the MJO intensity from the Maritime Continent to the western Pacific, the combination of ENSO and the QBO should change the MJO’s propagation differently compared to a single mode. For each El Niño/QBOE year ()), there is one MJO. An MJO originating from the Indian Ocean is suppressed over the Maritime Continent, but it can be reinforced when arriving in the western Pacific and can reach the central Pacific. For each El Niño/QBOW year ()), there is also one MJO, and the MJO can also be reinforced after passing over the Maritime Continent. The MJO declines quickly around 140°E. It seems that the QBO does not change the MJO’s propagation during a El Niño year.
Figure 3. MJO propagation patterns along the equator during (a) El Niño/QBOE, (b) El Niño/QBOW, (c) La Niña/QBOE, and (d) La Niña/QBOW phases. The patterns are shown by composites of OLR anomalies (contours; units: W m−2) for tracked boreal-winter MJO events formed west of the Maritime Continent (40°–100°E) during 1979–2016. Dots denote anomalies significant at the 0.05 level. The number in each panel denotes the number of MJO events per year during each phase. The red dashed lines denote the longitudinal range of the Maritime Continent (110°–140°E).
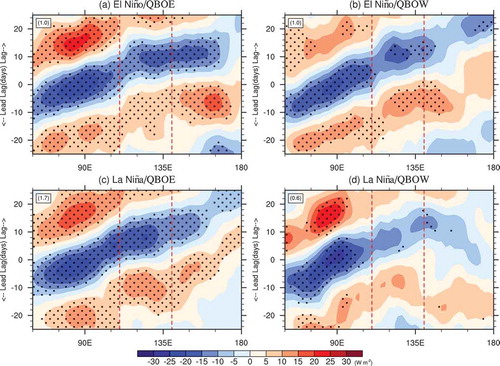
During a La Niña year (,), the effect of the QBO dominates. For each La Niña/QBOE year ()), there are 1.7 MJO events, which is much larger than that in an El Niño year and La Niña/QBOW year. The last phase has only 0.6 MJO events ()). During a La Niña/QBOE year, the MJO can propagate to the western Pacific without being suppressed over the Maritime Continent. During a La Niña/QBOW year, the existing MJO is observed to be enhanced over the Indian Ocean, while it is barely able to pass the Maritime Continent ()).
In summary, the QBO’s effect is weak in an El Niño year, and the reinforcement of the MJO by El Niño is likely the reason. The QBO’s effect whereby the MJO’s occurrence frequency increases and the Maritime Continent barrier decreases, mainly works during a La Niña year, and the MJO is rarely observed during a La Niña/QBOW year.
3.4 Possible mechanisms for these combined effects
To understand these different MJO responses to these combined effects of the QBO and ENSO, different mean states controlling the MJO’s evolution are shown in . Owing to the positive SST anomaly from the central Pacific to the eastern Pacific during El Niño years ()), a weakened Walker circulation causes a negative moisture anomaly ()) and downward motion ()) over the Maritime Continent from 110°E to 140°E, which tends to suppress the MJO. The positive specific humidity anomaly over the central Pacific from 160°E to 200°E ()), however, will reinforce the MJO when it reaches the western Pacific, because the easterly wind anomaly to the east of the MJO’s center will advect the moisture by this eastward gradient of the mean moisture anomaly.
Figure 4. Boreal-winter mean of equatorial (15°S–15°N) (a) SST (units: °C), (b) 1000–500-hPa averaged specific humidity (units: g kg−1), (c) 500-hPa vertical velocity (units: Pa s−1), and (d) high-cloud coverage (units: %) anomalies for El Niño/QBOE (red dashed), El Niño/QBOW (red solid), La Niña/QBOE (blue dashed), and La Niña/QBOW (blue solid) phases. Panel (e) shows the equatorial (10°S–10°N) diurnal range of precipitation intensity (mm h−1) for QBOE (red line) and QBOW (blue line) phases during boreal winter 1998–2016. Dots denote anomalies significant at the 0.05 level. The green dashed lines denote the longitudinal range of the Maritime Continent (110°–140°E).
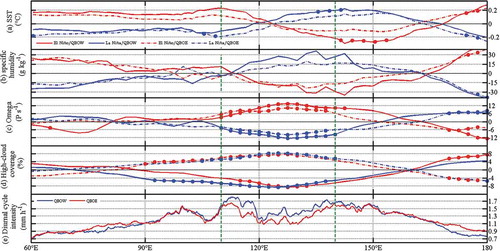
The QBO mainly affects the MJO’s propagation through changing high-cloud coverage ()), and the high-cloud coverage is much enhanced during a QBOE phase — a finding consistent with previous work (Zhang and Zhang Citation2018). Owing to the reduced insolation caused by greater high-cloud coverage, the diurnal cycle of precipitation intensity over the Maritime Continent is much suppressed during the QBOE ()), which will decrease the barrier effect of the Maritime Continent on the MJO (Hagos et al. Citation2016).
During El Niño years, the weakening of the MJO over the Maritime Continent is controlled by the El Niño-induced downward motion, even when the QBO is in its easterly phase ()). Over the western Pacific, the moisture advection of the El Niño-induced positive moisture anomaly from the central Pacific will reinforce the MJO, even when the QBO is in its westerly phase ()). As a result, the MJO is suppressed over the Maritime Continent and reinforced over the western Pacific.
During La Niña years, the weakening of the MJO over the Maritime Continent should reduce, and the QBOE phase also weakens the barrier effect of the Maritime Continent by decreasing the diurnal cycle; thus, the MJO can pass over the Maritime Continent without being weakened ()). The QBOW phase, however, will suppress the MJO intensity over the Maritime Continent through enhancing the diurnal cycle; plus, there is no reinforcement of the MJO over the western Pacific because of the cold central Pacific of La Niña. Thus, the MJO rarely reaches the western Pacific during the La Niña/QBOW phase ()).
4. Conclusions and discussion
In recent years, the effect of the QBO on the MJO has been investigated. It has been reported that the occurrence frequency of the MJO can be enhanced under the QBOE (Zhang and Zhang Citation2018). Here, we further explore how ENSO modulates this QBO effect. Our results show that MJO signals originating from the equatorial Indian Ocean rarely affect the western Pacific during a La Niña/QBOW year, whereas such signals can reach the western Pacific in both QBO easterly and westerly phases during an El Niño year ().
Further investigation by tracking each MJO event shows that the number of MJO events is small during La Niña/QBOW years (). Among the eight La Niña/QBOW years, four do not feature an MJO. During each La Niña/QBOE year, the occurrence frequency of MJO events is greatly enhanced. During El Niño years, the QBO effect is very weak. The reason is that, although El Niño can suppress the MJO over the Maritime Continent, it can also reinforce it over the western Pacific through moisture advection of the mean state via the MJO’s easterly wind anomaly. During La Niña years, however, this reinforcement process disappears because of the cold central Pacific (). Under the QBOW phase, the MJO is heavily suppressed over the Maritime Continent because of the enhanced diurnal cycle; thus, the MJO cannot reach the western Pacific because of the lack of reinforcement.
The findings of this work should improve our understanding of MJO dynamics, as well as subseasonal prediction when considering interannual variability. Although there is no significant relationship between the QBO and ENSO, the QBOE tends to weaken the amplitude of SST, moisture, and vertical velocity anomalies, regardless of the phase of ENSO. This impact of the QBO should be investigated in future studies.
Disclosure statement
No potential conflict of interest was reported by the authors.
Additional information
Funding
References
- Baldwin, M. P., L. J. Gray, T. J. Dunkerton, K. Hamilton, P. H. Haynes, W. J. Randel, J. R. Holton, M. J. Alexander, I. Hirota, and T. Horinouchi. 2001. “The Quasi‐Biennial Oscillation.” Reviews of Geophysics 39 (2): 179–229. doi:10.1029/1999RG000073.
- Collimore, C. C., D. W. Martin, M. H. Hitchman, A. Huesmann, and D. E. Waliser. 2003. “On the Relationship between the QBO and Tropical Deep Convection.” Journal of Climate 16 (15): 2552–2568. doi:10.1175/1520-0442(2003)016<2552:otrbtq>2.0.co;2.
- Dee, D. P., S. M. Uppala, A. J. Simmons, P. Berrisford, P. Poli, S. Kobayashi, U. Andrae, M. A. Balmaseda, G. Balsamo, and P. Bauer. 2011. “The ERA‐Interim Reanalysis: Configuration and Performance of the Data Assimilation System.” Quarterly Journal of the royal meteorological society 137 (656): 553–597. doi:10.1002/qj.828.
- Deser, C., M. A. Alexander, S. P. Xie, and A. S. Phillips. 2010. “Sea Surface Temperature Variability: Patterns and Mechanisms.” Annual Review of Marine Science 2 (1): 115. doi:10.1146/annurev-marine-120408-151453.
- Feng, J., P. Liu, W. Chen, and X. C. Wang. 2014. “Contrasting Madden-Julian Oscillation Activity during Various Stages of EP and CP El Niños.” Atmospheric Science Letters. doi:10.1002/asl2.516.
- Gualdi, S., A. Navarra, and G. Tinarelli. 1999. “The Interannual Variability of the Madden–Julian Oscillation in an Ensemble of GCM Simulations.” Climate Dynamics 15 (9): 643–658. doi:10.1007/s003820050307.
- Gushchina, D., and B. Dewitte. 2012. “Intraseasonal Tropical Atmospheric Variability Associated with the Two Flavors of El Niño.” Monthly Weather Review 140 (11): 3669–3681. doi:10.1175/mwr-d-11-00267.1.
- Hagos, S. M., C. Zhang, Z. Feng, C. D. Burleyson, C. D. Mott, B. Kerns, J. J. Benedict, and M. N. Martini. 2016. “The Impact of the Diurnal Cycle on the Propagation of Madden-Julian Oscillation Convection across the Maritime Continent.” Journal of Advances in Modeling Earth Systems 8 (4): 1552–1564. doi:10.1002/2016MS000725.
- Hendon, H. H., C. Zhang, and J. D. Glick. 1999. “Interannual Variation of the Madden-Julian Oscillation during Austral Summer.” Journal of Climate 12 (8): 2538–2550. doi:10.1175/1520-0442(1999)012<2538:ivotmj>2.0.co;2.
- Hood, L. 2017. “QBO/Solar Modulation of the Boreal Winter Madden-Julian Oscillation: A Prediction for the Coming Solar Minimum.” Paper presented at the EGU General Assembly Conference, Vienna. doi:10.1002/2017gl072832.
- Huffman, G. J., R. F. Adler, P. Arkin, A. Chang, R. Ferraro, A. Gruber, J. Janowiak, A. Mcnab, B. Rudolf, and U. Schneider. 1997. “The Global Precipitation Climatology Project (GPCP) Combined Precipitation Dataset.” Bulletin of the American Meteorological Society 78 (1): 5–20. doi:10.1175/1520-0477(1997)078<0005:tgpcpg>2.0.co;2.
- Kessler, W. S. 2001. “EOF Representations of the Madden-Julian Oscillation and Its Connection with ENSO.” Journal of Climate 14 (14): 3055–3061. doi:10.1175/1520-0442(2001)014<3055:erotmj>2.0.co;2.
- Lawrence, D. M., and P. J. Webster. 2010. “Interannual Variations of the Intraseasonal Oscillation in the South Asian Summer Monsoon Region.” Journal of Climate 14 (13): 2910–2922. doi:10.1175/1520-0442(2001)014<2910:ivotio>2.0.co;2.
- Liebmann, B., and C. A. Smith. 1996. “Description of a Complete (Interpolated) Outgoing Longwave Radiation Dataset.” Bulletin of the American Meteorological Society 77 (6): 1275–1277.
- Liess, S., L. Bengtsson, and K. Arpe. 2004. “The Intraseasonal Oscillation in ECHAM4 Part I: Coupled to a Comprehensive Ocean Model.” Climate Dynamics 22 (6–7): 653–669. doi:10.1007/s00382-004-0406-0.
- Ling, J., P. Bauer, P. Bechtold, A. Beljaars, R. Forbes, F. Vitart, M. Ulate, and C. Zhang. 2014. “Global versus Local MJO Forecast Skill of the ECMWF Model during DYNAMO.” Monthly Weather Review 142 (6): 2228–2247. doi:10.1175/mwr-d-13-00292.1.
- Liu, F., T. Li, H. Wang, L. Deng, and Y. W. Zhang. 2016a. “Modulation of Boreal Summer Intraseasonal Oscillations over the Western North Pacific by ENSO.” Journal of Climate 29 (20): 7189–7201. doi:10.1175/jcli-d-15-0831.1.
- Liu, F., and B. Wang. 2016. “Role of Horizontal Advection of Seasonal-Mean Moisture in the Madden–Julian Oscillation: A Theoretical Model Analysis.” Journal of Climate 29 (17): 6277–6293. doi:10.1175/jcli-d-16-0078.1.
- Liu, F., L. Zhou, J. Ling, X. Fu, and G. Huang. 2016b. “Relationship between SST Anomalies and the Intensity of Intraseasonal Variability.” Theoretical & Applied Climatology 124 (3–4): 1–8. doi:10.1007/s00704-015-1458-2.
- Madden, R. A., and P. R. Julian. 1971. “Detection of a 40–50 Day Oscillation in the Zonal Wind in the Tropical Pacific.” Journal of Atmospheric Sciences 28 (5): 702–708. doi:10.1175/1520-0469(1971)028<0702:doadoi>2.0.co;2.
- Marshall, A. G., H. H. Hendon, S. W. Son, and Y. Lim. 2016. “Impact of the Quasi-Biennial Oscillation on Predictability of the Madden–Julian Oscillation.” Climate Dynamics 49: 1–13. doi:10.1007/s00382-016-3392-0.
- Moon, J. Y., B. Wang, and K. J. Ha. 2011. “ENSO Regulation of MJO Teleconnection.” Climate Dynamics 37 (5–6): 1133–1149. doi:10.1007/s00382-010-0902-3.
- Nishimoto, E., and S. Yoden. 2017. “Influence of the Stratospheric Quasi-Biennial Oscillation on the Madden-Julian Oscillation during Austral Summer.” Journal of Atmospheric Sciences 74: 4. doi:10.1175/jas-d-16-0205.1.
- Pang, B., Z. Chen, Z. Wen, and L. U. Riyu. 2016. “Impacts of Two Types of El Ni(N)O on the MJO during Boreal Winter.” Advances in Atmospheric Sciences 33 (8): 979–986. doi:10.1007/s00376-016-5272-2.
- Rayner, N. A., D. E. Parker, E. B. Horton, C. K. Folland, L. V. Alexander, D. P. Rowell, E. C. Kent, and A. Kaplan. 2003. “Global Analyses of Sea Surface Temperature, Sea Ice, and Night Marine Air Temperature since the Late Nineteenth Century.” Journal of Geophysical Research: Atmospheres 108: D14. doi:10.1029/2002JD002670.
- Salby, M. L., and H. H. Hendon. 1994. “Intraseasonal Behavior of Clouds, Temperature, and Motion in the Tropics.” Journal of the Atmospheric Sciences 51 (15): 2207–2224. doi:10.1175/1520-0469(1994)051<2207:ibocta>2.0.co;2.
- Son, S., Y. Lim, C. Yoo, H. Hendon, and J. Kim. 2016. “Stratospheric Control of Madden-Julian Oscillation and Its Teleconnection.” EGU General Assembly Conference Abstracts, Vienna. Vol. 19. 2017.
- Son, S.-W., Y. Lim, C. Yoo, H. Hendon, and J. Kim. 2017. “Stratospheric Control of the Madden–Julian Oscillation.” Journal of Climate 30 (6): 1909–1922. doi:10.1175/jcli-d-16-0620.1.
- Waliser, D. E., Z. Zhang, K. M. Lau, and J. H. Kim. 2010. “Interannual Sea Surface Temperature Variability and the Predictability of Tropical Intraseasonal Variability.” Journal of the Atmospheric Sciences 58 (58): 2596–2615. doi:10.1175/1520-0469(2001)058<2596:isstva>2.0.co;2.
- Yoo, C., and S. W. Son. 2016. “Modulation of the Boreal Wintertime Madden‐Julian Oscillation by the Stratospheric Quasi‐Biennial Oscillation.” Geophysical Research Letters 43 (3): 1392–1398. doi:10.1002/2016gl067762.
- Zhang, C. 2005. “Madden‐Julian Oscillation.” Reviews of Geophysics 43 (2). doi:10.1029/2004RG000158.
- Zhang, C., and J. Ling. 2017. “Barrier Effect of the Indo-Pacific Maritime Continent on the MJO: Perspectives from Tracking MJO Precipitation.” Journal of Climate 30 (9): 3439–3459. doi:10.1175/jcli-d-16-0614.1.
- Zhang, C., and B. Zhang. 2018. “QBO‐MJO Connection.” Journal of Geophysical Research Atmospheres. doi:10.1002/2017jd028171.