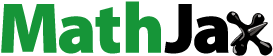
ABSTRACT
This study investigated the impact of openings on the punching shear strength of ‘non-coarse aggregate acrylic polymer concrete’ (APC) slabs. Twelve specimens representing scaled models of a flat, with different parameters such as concrete type, opening size, and distance from the column edge. The specimens were divided into five groups, including a control group of normal strength concrete and APC specimen without openings, as well as APC specimens with various opening sizes and distances, along with APC enhancement specimens. Experimental results were validated using Abaqus finite element software, demonstrating good agreement between the experimental and simulated outcomes. The analysis encompassed several aspects, including load-deflection response, ultimate loading capacity, post-ultimate loading capacity, failure patterns, and strain distribution. The absence of coarse aggregate in APC significantly impacted its shear resistance, leading to a reduction in internal friction. Compared to ordinary concrete slabs, the ultimate capacity of APC slabs without openings was approximately 36% lower. The presence of two openings adjacent to the column further decreased the ultimate capacity by approximately 33%. The size and distance of the openings played a crucial role in determining the ultimate capacity of APC slabs. Notably, the APC specimens exceeded the design ultimate load calculated by Egyptian Code of Practice (ECP-203-2020) and ACI-318-19 codes by approximately 1.4 and 1.6, respectively. However, the study advises against relying solely on APC in shear-sensitive constructions without integrating additional enhancements for shear resistance, as the results indicate a significant reduction in the shear resistance compared to ordinary concrete slabs.
Introduction
Polymer concrete has gained popularity in the construction industry due to its advantageous properties such as lightweight, self-compaction, workability, durable strength, quick curing, and low permeability. It is composed of a powdered material, often derived from recycled construction materials, mixed with a liquid polymer resin. While extensive studies have been conducted on the mechanical and chemical characteristics of different forms of polymer concrete, further investigations are still underway to explore its application in casting structural components. One important aspect of polymer concrete is its punching shear strength, particularly in flat slabs where it represents the most common failure mechanism.
Flat slabs are widely used in structural systems for their unique features [Citation1], as they transfer applied loads directly to columns without the need for beams or girders. However, the design can lead to concentrate shear and bending loads around the column-slab connections, making punching shear failure a significant concern [Citation2]. This failure mode is characterized by the development of a cone-shaped element associated with brittle failure. Consequently, the failure of a flat slab can potentially result in the progressive collapse of the entire structure, especially if the concrete quality is poor or the reinforcement is inadequate.
Incorporating openings near columns in flat slabs is a common practice to accommodate mechanical and electrical installations, lighting, pipelines, or elevators. However, these openings can reduce the amount of concrete available to resist punching shear and disrupt the arrangement of reinforcing bars. As a result, openings often increase punching shear stresses in reinforced concrete (RC) flat slabs and significantly limit their punching shear capacity [Citation3]. Therefore, it is crucial to analyze punching shear stresses in flat slabs with openings of varying sizes and positions.
Previous experiments on concrete have demonstrated that the presence of coarse aggregate creates a rough surface upon failure, while fine aggregate results in a smooth fractured surface. The interlock of aggregates on rough fractured surfaces enhances shear transfer, whereas the absence of coarse aggregate reduces the shear capacity of the element. To improve the overall shear capacity, the addition of another material to polymer concrete has been suggested [Citation4–10].
In order to have a comprehensive understanding of the punching shear behavior of polymer concrete flat slabs under different conditions, including the presence of openings, further studies are needed. These studies should also compare the behavior with that of normal strength concrete and other types of shear reinforcing components.
Therefore, the aim of this experimental program is to investigate the effect of openings on the punching shear behavior of polymer concrete slabs under static loads. Openings of various sizes will be placed near the column at different distances.
While previous research has explored various types of reinforcement, such as steel bars, studs [Citation3–6], shear bolts, screws [Citation6–8], and carbon fiber-reinforced polymer (CFRP) rods, grids, and fans [Citation6–9], to resist shear in RC flat slabs, there is still limited understanding of how openings specifically impact the punching shear behavior of two-way RC slabs [Citation11–13]. Furthermore, design codes lack reliable methods to estimate the punching resistance of structures with openings.
Although the size and position of openings significantly influence the punching shear behavior of RC flat slabs, comprehensive research on this topic remains limited. Previous studies have identified the junction of the two middle strips as the optimal zone for placing openings in two-way RC slabs [Citation14,Citation15]. It has also been observed that increasing the opening size and reducing the distance between the opening and the column edge decrease punching shear resistance [Citation16]. Moreover, it has been found that if openings are located at a distance greater than 4 times the slab thickness (4d) from the column, the same shear strength can be achieved as in a slab without openings [Citation17–19].
In light of the aforementioned gaps in knowledge, this study aims to assess the impact of openings on the punching shear strength of polymer concrete flat slabs. The parameters under investigation include the type of concrete, the size of the openings, and the distance of the openings from the column edge. Testing will involve casting and examining specimens made of normal concrete and polymer concrete with various opening sizes and positions relative to the column. The absence of coarse aggregates in polymer concrete, as well as the size and distance of the openings from the column face, are expected to have an effect on shear resistance. By delving into a more detailed discussion on the influence of test parameters and the underlying mechanical mechanisms, this study aims to enhance the overall quality of knowledge in this area.
The study aims to advance the understanding of the behavior of APC concrete slabs with openings subjected to punching shear and the effect of different enhancement procedures on its behavior. The insights gained from this research can have practical implications for the design and construction of flat slab structures, leading to more efficient and reliable structural solutions.
Characteristics of materials used
Acrylic polymer concrete consists of powder and liquid polymer and water. The powder was tested by X-Ray fluorescence spectroscopy (XRF), all components shown in , while the Liquid Polymer is a solution mix with water before adding to powder.
Table 1. Acrylic powder components.
Characteristics mix properties
The Acrylic Polymer Concrete mixed from three components (powder, polymer and water), the percentage of water was 17% of powder, and the polymer percentage was 0.333% of water. This mix design was recommended by the manufactures.
6 standard cubes (15 × 15 * 15) were tested for compression with each specimen.
3 Standard cylinders (300 mm length with 150 mm diameter) were casted and tested to get the stress strain curve as shown in .
Brazilian tensile strength test were used to determine the tension strength for APC by testing 3 cylinders, , show the Brazilian tensile strength test setup and illustrate the tension strength results for the test specimens, The average value of ‘Fctr’ illustrated from test specimens is 1.27 N/mm2 which equal about 4.37% of compression strength.
Table 2. Brazilian tensile strength test results.
Reinforcement steel
Two types of steel were used in this research, high-strength and mild-strength reinforcement. present the mechanical properties of the used steel.
Table 3. Mechanical properties of steel bars.
Test program
A series of tests were conducted to assess the punching shear capacity of APC flat slabs, involving a total of nine slabs with and without openings. The experimental program aimed to investigate the influence of various factors, such as the size and position of the openings, on the shear strength of the slabs.
The specimens had dimensions of (1700 × 1700) mm and a thickness of 120 mm. The lower mesh reinforcement consisted of 8 bars per meter, each with a diameter of 16 mm, while the upper mesh reinforcement comprised 8 bars per meter with a diameter of 10 mm. Additional bars were incorporated in the slabs with openings according to the recommendations outlined in the ECP-203-2020 [Citation20].
All the slabs were constructed with a central column measuring (200 × 200) mm and a height of 250 mm. The column itself was reinforced with four steel bars, 16 mm in diameter, and stirrups were provided using 8 mm bars spaced at 50 mm intervals, as depicted in .
Furthermore, three specimens underwent enhancements to improve their shear resistance. The first specimen was reinforced with inverted beams around the openings dropped by additional depth equal to t/4, with width equal to d reinforced by shear stirrups Y8@50, this beam is just around the openings edge as such used in many practice situations. The second specimen had shear stirrups Y8@50 added around the openings, and the third specimen had steel fiber incorporated into the polymer mix used for casting width equal (d) around the openings, as shown in . These enhanced specimens were tested to assess the performance of APC in combination with the enhancement measures.
The experiment involved casting multiple slabs with varying sizes and positions of openings in relation to the column. The openings were located adjacent to the column, at a distance of ‘d’, and at a distance of 3d from the column face. The openings were aligned with the column’s length. Ten slabs were cast using APC mix, while two slabs were cast using ordinary concrete for comparison. , along with , provide detailed dimensions and reinforcement information for the specimens.
Table 4. Details of tested specimens.
Testing of specimens
For the current study, six cubes measuring 150 × 150 × 150 mm and three cylinders were cast for each casting group to assess the compressive strength of both APC and concrete specimens. The casting and curing procedures followed standard protocols to determine the mechanical properties of APC, including its stress-strain curve and Young’s modulus.
The specimens were tested horizontally, with a vertical load applied through the column stub. A load control system was used to apply and regulate the load. A square steel frame, consisting of four standard IB no 26 with stiffeners at 20 cm intervals, provided support for the slab. This steel frame rested on four RC blocks measuring 50 × 50 * 70 cm, which were placed on the ground. A hinged support was created at the slab edge using a 4 cm diameter round bar and a 0.8 cm thick, 6 cm wide steel plate over the square steel frame, as depicted in .
Displacement control sensors were employed to measure the deformations in the slab. These sensors were mounted on a handling unit positioned on the ground beneath the center of the slab. LVDTs and strain gauges were strategically placed as shown in . Among them, LVDT1, located at the middle of the slab beneath the column, provided the most reliable readings. This LVDT reading was particularly important as it was placed in the critical area where the punching shear effect would occur.
Testing procedure
During the testing process, the hydraulic jack was utilized to generate pressure by pumping oil into the system. This pressure was then transmitted to the specimens being tested. A load cell, which is a device designed to measure force or load, was placed below the jack. It was connected to an electronic system that allowed for the accurate measurement of the load applied to the specimen.
To ensure a comprehensive understanding of the specimen’s behavior, the load was applied gradually and slowly. This approach allowed the researchers to closely observe the initiation of cracks and monitor the specimen’s response to the applied load. By recording their observations at various stages of loading, the researchers could gather valuable data on the specimen’s structural performance, crack propagation, and overall behavior under stress.
Analyzing the crack initiation and subsequent crack propagation provided insights into the specimen’s strength, durability, and deformation characteristics. This information was crucial for evaluating the performance and structural integrity of the material being tested, as well as for informing future design considerations and improvements in similar applications.
Test results
The specimens were subjected to an incremental increase in applied load until the ultimate load was reached. This incremental loading process allowed researchers to observe the behavior of the specimen under gradual loads. During the initial stages of loading. Typically, cracks begin to appear on the lower face of the slab once the load reaches the crack load.
As the loading continued beyond the crack load, the cracks in the specimen gradually propagated and spread. Meanwhile, the load-deflection curve exhibited a linear relationship up to approximately 0.8 of the ultimate loads. Beyond this point, the load-deflection curve deviated from linearity, indicating nonlinear behavior. Strain gauges were used to measure the strain in the reinforcement, providing valuable data for understanding the load transfer mechanisms and failure patterns. By analyzing this data, it was found that all specimens failed in punching shear mode failure except specimen (p-S) has failed in Flexural Punching failure.
presents the recorded maximum load and corresponding vertical deflection obtained from the tests. In the control group, two specimens (CO-S & CO-0-C) failed in a punching shear mode, while the specimen (p-S) failed due to flexural punching. The failure load of the (APC) specimen decreased by approximately 34% compared to the ordinary concrete specimen (CO-S). These results indicate that the absence of coarse aggregate in the APC mix has a significant impact compared to using ordinary concrete, as depicted in .
Table 5. Summary of test results.
By analyzing , it was observed that the slopes of the slabs in the control group varied. Specimen (CO-S) had the steepest slope, followed by specimen (p-S), and then specimen (CO-0-C). Although specimen (CO-0-C) was made of normal concrete with openings adjacent to the sides of the column, it affected the behavior of the slab, resulting in reduced carrying capacity and deflection at failure. On the other hand, while APC decreased the carrying capacity of the slab, it also reduced the deflection value by approximately 9% compared to specimen (CO-S). This decrease in deflection is attributed to the absence of coarse aggregates in APC.
Furthermore, it was observed that the cracks in the ordinary concrete specimens ((CO-S) &; (CO-0-C)) exhibited greater length and a larger distribution area compared to the cracks in the corresponding APC specimens ((p-S) &; (p-0-C)). The absence of coarse aggregates in APC reducing crack length and distribution. Coarse aggregates in ordinary concrete can act as stress concentrators, promoting crack initiation and propagation. In APC, the absence of these coarse aggregates reduces stress concentrations and limits crack development
All other specimens in the following groups (APC specimens) failed due to pure punching shear. The values of failure loads in their corresponding deflections of the slabs are shown in .
The general behavior of the different specimens of tested slabs were relatively constant in all the tests. All the specimens exhibited punching shear. The load-deflection curves are shown in ). By observing figures thoroughly, it is noticed that at the beginning of the testing procedure, the slabs followed a linear behavior corresponding to the uncracked behavior, then a zone where the slab’s behavior deflected, indicating a reduction in their stiffness. No curve showed a sign of plastic deformation.
Shows that the ultimate load was increased by increasing the opening distance from the column face. The ultimate load increased by about 31% with the opening movement from just at the column face to a distance equal to ‘d’ from the column face. The ultimate load increased by about 11% with the opening movement from distance ‘d’ from the column face to distance ‘3d’. At the same time, increasing the ultimate load values lead to increases the deflection values, but not in the same manner.
The deflection values were the same for both specimens (p-S and p-0-C), the lower value in the group. On the other hand, the deflection value for the specimen (P-d-C) was more significant than that for the specimen (p-3d-C). We noticed that the opening location of APC slabs did not have a specific behavior regarding the deflection value; the presence of extra reinforcing bars around the opening did not control the slabs’ deflection.
shows the behavior of specimens with opening size equal to half the column dimension. The ultimate load increases by increasing the distance between the opening and the column face. The ultimate load increased by about 15% with the opening movement from just at the column face to a distance equal to ‘d’ from the column face. The ultimate load increased by about 6% with the opening movement from distance ‘d’ from the column face to distance ‘3d’.
In all cases, the deflection values were almost the same except for specimen (P-d-0.5C); it was noticed that an increase in its deflection value accompanied the increase in the slab’s ultimate load.
presents the results of investigating different enhancement methods on the behavior of APC specimens.
The first method examined is the use of a beam around the opening. This approach involves placing a reinforced beam around the perimeter of the opening to distribute the shear forces. The results show that this method is highly effective, as it leads to a significant increase in both the ultimate load and the deflection capacity of the APC specimens. The ultimate load increased by approximately 307% compared with specimen (p-0-C), while it increased by about 206% comparing with APC specimen without openings (p-S), and about 131% more than the solid ordinary concrete specimen (CO-S), indicating a substantial improvement in structural performance. Moreover, the deflection increased by about 180%. This suggests that the beam reinforcement contributes to the overall flexibility and deformability of the structure.
The second method investigated is the use of shear reinforcement stirrups placed vertically along the perimeter of the opening to enhance the specimen’s resistance to punching shear. The results indicate that shear reinforcement stirrups also have a positive effect on the APC specimens. The ultimate load increased by approximately 148% comparing with specimen (p-0-C), while it reach about 99% from the ultimate load of APC specimen without openings (p-S). Additionally, the deflection at the ultimate load was reduced by approximately 12%. The reduction in deflection suggests that the presence of stirrups helps to control the deformation and improve the stiffness of the specimens.
Interestingly, the specimens with shear stirrups exhibit a gradual reduction in capacity rather than a sudden failure. This behavior is attributed to the increased ductility provided by the shear stirrups. The presence of shear stirrups enhances the ductility of the APC specimens, allowing them to exhibit more gradual and controlled failure mechanisms.
The third method investigated in the study is the addition of steel fibers to the APC mix. The results show that the addition of steel fibers has the least effect on punching shear enhancement compared to the other methods. The ultimate load increased by approximately 130% when steel fibers were added, indicating a moderate improvement. On the other hand, the deflection at the ultimate load was reduced by approximately 24%, suggesting a slight improvement in stiffness compared to specimens without steel fibers.
Overall, the study highlights the effectiveness of different enhancement methods in improving the behavior of APC specimens subjected to punching shear. The use of a beam around the opening demonstrates the highest efficiency, followed by shear reinforcement stirrups. The addition of steel fibers has a relatively smaller impact on punching shear enhancement. These findings provide valuable insights for designing and reinforcing structures to enhance their punching shear resistance and overall structural performance.
Theoretical verification
The finite element software ABAQUS (2017) [Citation21] was utilized to examine the behavior of polymer concrete slabs with openings. The given model was utilized to verify the experimental investigation of tested specimens.
Material modeling of tested specimens
Concrete
The concrete material was modeled using damage plasticity in ABAQUS software. The failure types in concrete are tensile cracking and compressive crushing. The progress of the failure surface is determined by tension and compression equivalent plastic strains, as shown in .
Reinforcement
The steel can be modeled using an elastic completely plastic, as shown in . Full bond between embedded reinforcement and concrete is assumed.
Abaqus finite element package was used to verify the results of the tested specimens to ease future work in investigating APC slab behavior. The results obtained from Abaqus were compared to those of the experimental program, as shown in .
Table 6. Comparison between experimental and theoretical results.
Specimens capacity according to codes equations
Egyptian code of practice (ECP-203-2020)
The specimens punching shear capacity were calculated according to ECP section [2–2,3] as follow.
qcup (concrete punching shear strength) according to ECP is the minimum of the followings.
qup (concrete punching shear capacity) ɣc (concrete reduction factor = 1.5)
b0 (punching shear perimeter) a&b (column dimension)
α (factor equal 4 for interior column, 3 for edge column, and 2 for corner column)
ACI-318-19 [Citation22]
The specimens punching shear capacity were calculated according to ACI as follow
Least of (a), (b), and (c).0.33
Notes:
is the size effect factor calculated from formula. →
β is the ratio of long to short sides of the column, concentrated load, or reaction area.
αs is 40 for interior columns, 30 for edge columns, and 20 for corner columns.
The specimens punching shear capacity (considering above codes equations for normal concrete) were calculated for both codes and listed in to compare the results with the experimental and theoretical ultimate load results.
shows that the ultimate loads obtained from both the theoretical model and the experimental program varied between −3% to 6%, indicating the reliability of those models for further investigation of the APC slabs’ behavior. Moreover, by comparing the experimental and theoretical load-deflection curves, it is noticed that the curves almost coincided in most cases, indicating that the slabs experienced the same behavior in both investigations.
On the other hand, also shows the design values of the ultimate loads of the specimens according to the design equations of the regular concrete for both the Egyptian and ACI codes, which showed that ordinary concrete specimens reached a failure load exceeding twice the design ultimate load, while APC specimens failure load exceed the design ultimate load by approximately 140% by the equations of the Egyptian code and about 160% by the equations of the ACI code
presents the experimental and theoretical curves and failure mode shape for all specimens in different cases and parameters, which illustrate the compatibility of the ultimate load, elastic behavior, and the failure mode shape in all specimens between the experimental results and the finite element model results.
Table 7. Experimental and theoretical curves and failure mode shape.
Conclusions
This study includes an experimental and numerical examination of the behavior of non-coarse aggregate polymer concrete slabs with an opening under punching loads. And also explain the shear strength issue of polymer concrete, specifically in punching resistance. From both experimental and numerical results, the following conclusions can be written:
It was observed that the ultimate capacity of non-coarse aggregate polymer concrete slabs, both with and without openings, was significantly lower compared to ordinary concrete slabs. Specifically, the ultimate capacity of non-coarse aggregate polymer concrete slabs without openings decreased by approximately 36% compared to ordinary concrete slabs. When two openings were introduced adjacent to the column, the ultimate capacity decreased by about 22% compared to ordinary concrete slabs.
The presence of openings adjacent to the column had a further detrimental effect on the ultimate capacity of both ordinary concrete slabs and non-coarse aggregate polymer concrete slabs. In fact, the introduction of two openings led to a reduction in the ultimate capacity of ordinary concrete slabs by approximately 45%, while the reduction was around 33% for non-coarse aggregate polymer concrete slabs.
Additionally, the study highlighted the influence of the opening size and its proximity to the column face on the ultimate capacity of non-coarse aggregate polymer concrete slabs. Increasing the size of the opening or decreasing its distance from the column face resulted in a decrease in the ultimate capacity of the slabs. This suggests that careful consideration should be given to the design and placement of openings in polymer concrete slabs to maintain their structural integrity.
Using a beam around the opening exhibited the highest efficiency in enhancing the behavior of APC specimens subjected to punching shear. This method resulted in an approximate 307% increase in ultimate load and an approximately 180% increase in deflection capacity. The presence of the reinforced beam contributed to the overall flexibility and deformability of the structure.
Shear reinforcement stirrups placed vertically along the perimeter of the opening also had a positive effect on the behavior of APC specimens. The ultimate load increased by approximately 148% compared to specimens without shear reinforcement stirrups, while the deflection at the ultimate load was reduced by approximately 12%. The use of shear stirrups helped control deformation and improve the stiffness of the specimens.
The addition of steel fibers to the APC mix had the least effect on punching shear enhancement compared to the other methods. The ultimate load increased by approximately 130%, indicating a moderate improvement, while the deflection at the ultimate load was reduced by approximately 24%, suggesting a slight improvement in stiffness.
The ordinary concrete specimens achieved failure loads that exceeded twice the design ultimate load calculated by both ECP and ACI Codes. In contrast, the APC specimens exceeded by about 1.4&1.6 the design ultimate load calculated by ECP and ACI codes respectively.
This study cautions against relying solely on APC (non-coarse aggregate polymer concrete) in shear-sensitive constructions without incorporating additional measures to enhance shear resistance. The findings reveal a significant decrease in shear resistance compared to regular concrete slabs. However, the study demonstrates the effectiveness of various enhancement techniques. The most efficient method involves employing a beam around the opening, followed by the use of shear reinforcement stirrups. The inclusion of steel fibers has a relatively smaller impact on enhancing punching shear. These insights are valuable for the design and reinforcement of structures, aiming to improve their punching shear resistance and overall structural performance. Moreover, the study establishes a close correlation between experimental and numerical results, affirming the effectiveness of finite element modeling in evaluating the behavior of APC slabs with openings under punching loads.
Disclosure statement
No potential conflict of interest was reported by the author(s).
References
- Torabian A, Isufi B, Mostofinejad D, et al. Behavior of thin lightly reinforced flat slabs under concentric loading. Eng Struct. 2019;196:109327. doi: 10.1016/j.engstruct.2019.109327
- Torabian A, Isufi B, Mostofinejad D, et al. Flexural strengthening of flat slabs with FRP composites using EBR and ebrog methods. Eng Struct. 2020;211:110483. doi: 10.1016/j.engstruct.2020.110483
- Mostofinejad D, Jafarian N, Naderi A, et al. Effects of openings on the punching shear strength of reinforced concrete slabs. Structs. 2020;25:760–773. doi: 10.1016/j.istruc.2020.03.061
- Choulli Y, Marí AR, Cladera A. Shear behavior of full-scale prestressed I-beams made with self compacting concrete. Mater Struct. 2007;41(1):131–141. 10.1617/s11527-007-9225-1.
- Hussein YM, Metwally IM, El-Betar SA. Shear behavior of reinforced beams cast using non-coarse aggregate polymer concrete (icon). Civil Engg Res Magazine, Faculty Of Engg, AlAzhar University. 2011;2011(1): 1–10 10.5402/2011/374807
- Elzeiny SM Shear behavior of steel fibers reinforced beams using non coarse aggregate acrylic polymer concrete. Civil Engg Res Magazine, Faculty Of Engg, Al-Azhar Univer. 2012;34(2):237–250.
- Knaack A, Kurama Y. Design of concrete mixtures with recycled concrete aggregates. ACI Mater J. 2013;110(5). doi: 10.14359/51685899
- Prince MJ, Singh B. Investigation of bond behaviour between recycled aggregate concrete and deformed steel bars. Struct Concr. 2014;15(2):154–168. doi: 10.1002/suco.201300042
- Fan X, Zhang M. Experimental study on flexural behaviour of inorganic polymer concrete beams reinforced with basalt rebar. Composites. 2016;93:174–183. doi: 10.1016/j.compositesb.2016.03.021
- Salem M, Mourad A, Shehata A (2019). Behavior of non-coarse aggregate polymer concrete simply supported deep beams with and without web openings. Int J Scient Engg Res (IJSER), 10(2), 483–490.
- El-Salakawy EF, Polak MA, Soudki KA. New shear strengthening technique for concrete slab-column connections. ACI Struct J. 2003;100(3). doi: 10.14359/12604
- Lowe D, Roy K, Das R, et al. Full scale experiments on splitting behaviour of concrete slabs in steel concrete composite beams with shear stud connection. Struct. 2020;23:126–138. doi: 10.1016/j.istruc.2019.10.008
- Issa AM, Salem MM, Mostafa MT, et al. Performance of shear reinforcement against punching shear loads. Int J Eng Adv Technol. 2019;9(2):841–850. doi: 10.35940/ijeat.b3975.129219
- Borges LLJ, Melo GS, Gomes RB. Punching shear of reinforced concrete flat plates with openings. ACI Struct J. 2013;110(4). doi: 10.14359/51685740
- Mota MC, Kamara M Floor openings in two-way slabs. Concr Int 2006;28(7):33–36.
- Anil Ö, Kina T, Salmani V. Effect of opening size and location on punching shear behaviour of two-way RC slabs. Mag Concr Res. 2014;66(18):955–966. doi: 10.1680/macr.14.00042
- Teng S, Cheong HK, Kuang KL, et al. Punching shear strength of slabs with openings and supported on rectangular columns. ACI Struct J. 2004;101(5). doi: 10.14359/13390
- Anil Ö, Kaya N, Arslan O. Strengthening of one way RC slab with opening using CFRP STRIPS. Constr Build Mater. 2013;48:883–893. doi: 10.1016/j.conbuildmat.2013.07.093
- Afefy HM, Fawzy TM. Strengthening of RC one-way slabs including cut-out using different techniques. Eng Struct. 2013;57:23–36. doi: 10.1016/j.engstruct.2013.09.013
- ECP203-2020: Egyptian Code of Practice – For design and construction Reinforced Concrete. Cairo. Egypt.
- ABAQUS. SIMULIA user assistance 2017, dassault systems similia corp. 2017.
- ACI 318-19: Building code requirements for structural concrete: CCOMMENTARY on building code requirements for structural concrete (ACI 318R-19). American Concrete Institute. 2019.