1. Introduction
DNA nanotechnology has moved from a structure-centric field into more focus on functionalities and applications. The delivery of drugs is among the most intensely studied applications for DNA nanotechnology and for obvious reasons. Methods such as Rothemund’s DNA origami, single-stranded DNA tiles, and the more recent polygonal DNA origami have enabled formation of structurally defined nanoparticles of various shapes and sizes, which appear to be crucial parameters for the fate of systemic nanoparticles. The inherent structural principles of DNA nanostructures, the programmability of the individual building blocks, allow for attachment of molecules in rationally designed numbers and patterns. This can, for example, affect their interaction with living cells by tuning either the position, or stoichiometry of the functional molecules. In addition, DNA nanostructures have the unique potential to control large dynamic changes induced by strand displacement reactions.
Although clinical applications are still far from realization, several in vitro experiments have been conducted throughout the past decade. This has yielded new insights into the behavior of DNA nanostructure stability toward DNases and serum proteins, uptake into cultured cells, and the delivery of drugs. The results and conclusions from these studies have already been reviewed in great detail [Citation1,Citation2]. The full complexity and multifaceted challenges related to in vivo experiments, however, cannot be truly mimicked in vitro and results can rarely be extrapolated directly to in vivo experiments. Therefore, in vivo experiments have emerged during the past 5 years ( and ) where DNA nanostructures are injected into animals and the pharmacodynamics and pharmacokinetics are studied. Here, we review the methods and results from the 12 papers published so far on this topic.
Table 1. Overview of the literature describing DNA nanostructures in animal experiments.
Figure 1. Graph showing the number of articles published and corresponding number of citations for search terms ‘DNA nanotechnology’ and ‘drug delivery’ in titles, abstracts or keywords (www.scopus.com). Red asterix (*) is placed at 2011, when the first paper containing an animal experiment for a DNA nanostructure was published28. The count for 2016 is from August. Full color available online.
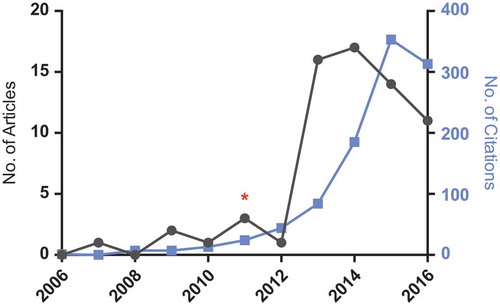
2. Animal experiments
The first in vivo experiment on a DNA nanostructure was reported in 2011 on Caenorhabditis elegans (C. elegans) by Krishnan and colleagues. They showed that an icosahedra, ~17 nm on each side, is taken up into coelomocytes through anionic ligand-binding receptors and can deliver fluorescein isothiocyanate-labeled 10-kDa dextran [Citation3]. To study the stability of the icosahedra inside the nematode, they later developed a Förster resonance energy transfer (FRET)-based method [Citation6], similar to what has been used by Turberfield in cell culture. The lifetime of the icosahedra was found to be more than 24 h, compared to ~14 h for the linear unstructured counterpart.
Bachelet and his team have studied a barrel-shaped DNA nanorobot inside living cockroaches (Blaberus discoidalis) [Citation8]. Intriguingly, the study shows that dynamic DNA structures can perform simple Boolean computations in a living animal. For example, two nanorobots loaded with DNA key oligos can activate a third nanorobot to emulate an OR gate and bind host hemocytes. In this paper, the cue to open the two key-caring nanorobots was human proteins: platelet-derived growth factor and vascular endothelial growth factor. Future experiments should tell whether DNA nanorobots can also be activated by endogenous molecules. In vitro, the nanorobots can induce cell signaling in human leukocytes [Citation15]. It will be interesting to see whether this ability is retained in vivo. In a recent study, Bachelet and his colleagues demonstrate that nanorobots inside cockroaches can be temporally controlled by human brain activity [Citation11].
Both C. elegans and cockroaches differ from vertebrates in that they do not have a closed circulatory blood system, nor do they have a fixed body temperature. To better mimic the environment and challenges of the human body, recent papers have investigated the behavior of DNA nanostructures in mice. The 186 base pair DNA tetrahedron was the first to be administered in mice. Lee and coworkers decorated the tetrahedron with up to six small-interfering RNA molecules labeled with the tumor targeting agent folate [Citation5]. It showed that siRNA-decorated tetrahedrons injected into the tail vein of mice accumulate in KB tumor xenografts and knock down gene expression, although it requires relatively high dosages (initial blood concentrations [IBCs] were higher than 500 nM). The blood circulation time was estimated to be ~24.2 min for the tetrahedrons compared to ~6 min for the parent siRNA. Jiang and coworkers, however, recently found a blood circulation time of ~5.3 min for the DNA tetrahedron [Citation14]. They studied the biodistribution of the tetrahedron using near-infrared fluorescence and single-photon emission computed tomography (SPECT) simultaneously. Unlike Lee and coworkers, who found accumulation in the kidneys 12 h post injection, Jiang and coworkers found that tetrahedrons are quickly excreted to the urine through the kidneys. They found the liver to be the primary organ for accumulation 2 h post injection. This discrepancy could be explained by the difference in administered amounts (Jiang and coworkers used at least five times less tetrahedron than Lee and coworkers). A study by Li and coworkers uses a 99mTc-labeled DNA bipyramid to enable SPECT imaging in mice and found a similar blood circulation time of ~6 min and accumulation in liver and kidneys [Citation13], but with much lower IBC (~12 nM). Huang and coworkers recently reported the delivery of the metal complex ruthenium polypyridyl (RuPOP) through interaction with the DNA tetrahedron [Citation12]. They are the first to report reduced tumor growth for mice administered with DNA tetrahedrons and found them to accumulate mainly in the liver, but also in kidneys and tumor. It should be considered, that IBCs of DNA tetrahedrons were very high (~1800 nM), but without any reported toxicity.
Besides cancer cell uptake, immunostimulation using CpG oligos anchored to DNA nanostructures have been widely studied in vitro. Liu and coworkers have shown that the DNA tetrahedron can also be used to present antigen-adjuvant complexes to boost the activation of immune cells in vivo [Citation4].
DNA origami structures have also been tested in mice. Compared to the DNA tetrahedron they are ~40-fold larger with respect to molecular mass and charge. Perrault and his team found that a DNA octahedron origami is primarily excreted to the urine after 2 h [Citation9]. The blood circulation time was ~49 min, but is increased more than fivefold by encapsulation in a liposome. In agreement with this, the biodistribution analysis shows that the encapsulated DNA origami was primarily in the blood after 2 h and a smaller fraction accumulated in the liver as expected for liposomal delivery. Same year, Zhang and coworkers demonstrated the potential for DNA origami to deliver the chemotherapeutic molecule doxorubicin to tumors in mice [Citation7]. This strategy has been confirmed several times in vitro and plays to the observation that doxorubicin is easily loaded into DNA nanostructures through intercalation. Remarkably, the study reports an extraordinary high-loading capacity of more than 60 doxorubicin molecules per base pair. Three different DNA origami structures were tested at relatively low IBCs (0.15 nM) and have similar biodistribution profiles, with passive accumulation in tumor, liver, and kidneys. Noteworthy, the triangular-shaped DNA origami has significantly better uptake in tumors than a rectangle and a tube and is the first DNA nanovehicle to reduce tumor size. The triangular shape was also chosen for in vivo experiments by Jiang and coworkers to deliver gold nanorods to tumor xenografts in mice [Citation10]. By applying near-infrared laser irradiation, the delivered gold nanorods can be specifically heated, kill tumor cells, and improve survival rates.
3. Expert opinion
The experiments by Krishnan in C. elegans have shown that cargo-loaded DNA nanovehicles can enter cells through receptor-mediated endocytosis. Bachelet has shown that DNA origami structures can interact and undergo large conformational changes as well as bind to cells in vivo in cockroaches. Both C. elegans and cockroaches have the advantages that they have low systemic nuclease activity and low systemic volumes. Mice, however, resemble the human anatomy and the biological barriers related to this much better. They contain the same large organs, such as heart, lungs, kidneys, liver, and spleen, which can be assessed for nanoparticle accumulation in biodistribution profiling. Experiments have shown that the liver and the kidneys are the main responsible for DNA nanovehicle clearance in mice. Other studies involving xenografted tumors have demonstrated passive accumulation of DNA nanovehicles in tumors, likely due to the enhanced permeation and retention effect caused by increased vascularization around tumors.
More animal experiments will emerge and should aim to address fundamental challenges for DNA nanovehicles, such as their structural integrity, passive homing to tumors, and interactions with the immune system. Wireframe DNA origami structures, which have been reported recently and have shown to be more stable in biological liquids should be tested in animals. To assess the structural integrity of DNA nanovehicles, Krishnan suggests a method in C. elegans based on FRET, however, this method is not suitable for experiments in mice. Therefore, new methods to probe the structural integrity of DNA nanovehicles in larger animals are needed. The ability of DNA nanovehicles to target tumors appears to depend on their shape [Citation7] and interactions of nanoparticles with complement factors are also shape dependent. Accordingly, future experiments should aim to elucidate this, as it may not only provide valuable insight for DNA nanovehicles, but for drug delivery vehicles in general.
A crucial barrier to overcome is the immune system [Citation2]. Zhang and colleagues found no effect on blood cell counts or TNF-a levels in mice after administration of doxorubicin-loaded DNA origami structures, however, interferon and cell apoptosis levels, which are often elevated in response to foreign DNA, have not been investigated in literature so far. It will benefit the further progression of DNA nanovehicles if assays to test immunogenicity become the standard for future animal experiments.
Declaration of interest
The authors have no other relevant affiliations or financial involvement with any organization or entity with a financial interest in or financial conflict with the subject matter or materials discussed in the manuscript apart from those disclosed.
Additional information
Funding
References
- Chen Y, Groves B, Muscat RA, et al. DNA nanotechnology from the test tube to the cell. Nat Nanotechnol. 2015 Sep 3;10(9):748–760.
- Surana S, Shenoy AR, Krishnan Y. Designing DNA nanodevices for compatibility with the immune system of higher organisms. Nat Nanotechnol. 2015 Sep 3;10(9):741–747.
- Bhatia D, Surana S, Chakraborty S, et al. A synthetic icosahedral DNA-based host-cargo complex for functional in vivo imaging. Nat Commun. 2011 Jan;2(May):339.
- Liu X, Xu Y, Yu T, et al. A DNA nanostructure platform for directed assembly of synthetic vaccines. Nano Lett. 2012;12(8):4254–4259.
- Lee H, Lytton-Jean AKR, Chen Y, et al. Molecularly self-assembled nucleic acid nanoparticles for targeted in vivo siRNA delivery. Nat Nanotechnol. 2012 Jun;7(6):389–393.
- Surana S, Bhatia D, Krishnan Y. A method to study in vivo stability of DNA nanostructures. Methods. 2013;64(1):94–100.
- Zhang Q, Jiang Q, Li N, et al. DNA origami as an in vivo drug delivery vehicle for cancer therapy. ACS Nano. 2014;8(7):6633–6643.
- Amir Y, Ben-Ishay E, Levner D, et al. Universal computing by DNA origami robots in a living animal. Nat Nanotechnol. 2014 Apr 6;9(5):353–357.
- Perrault SD, Shih WM. Virus-inspired membrane encapsulation of DNA nanostructures to achieve in vivo stability. ACS Nano. 2014 May 27; 8(5):5132–5140.
- Jiang Q, Shi Y, Zhang Q, et al. A self-assembled DNA origami-gold nanorod complex for cancer theranostics. Small. 2015;11(38):5134–5141.
- Arnon S, Dahan N, Koren A, et al. Thought-controlled nanoscale robots in a living host. PLoS One. 2016;11(8):e0161227.
- Huang Y, Huang W, Chan L, et al. A multifunctional DNA origami as carrier of metal complexes to achieve enhanced tumoral delivery and nullified systemic toxicity. Biomaterials. 2016;103:183–196.
- Li J, Jiang D, Bao B, et al. Radiolabeling of DNA bipyramid and preliminary biological evaluation in mice. Bioconjug Chem. 2016;27:905–910. acs.bioconjchem.5b00680.
- Jiang D, Sun Y, Li J, et al. Multiple-armed tetrahedral DNA nanostructures for tumor-targeting, dual-modality in vivo imaging. ACS Appl Mater Interf. 2016;8(7):4378-4384.
- Douglas SM, Bachelet I, Church GM. A logic-gated nanorobot for targeted transport of molecular payloads. Science (80-). 2012 Feb 17;335(6070):831–834.