1. What makes an effective transdermal formulation?
The answer is straightforward – it must be thermodynamically stable yet still allow enough drug to partition into the stratum corneum so that the resulting transdermal flux ensures that drug levels in the blood are sufficient for therapeutic concentrations to be achieved in the target compartment. Putting this into practice is more difficult since it involves the interplay between the thermodynamics that govern formulation stability and drug partitioning, the kinetics of drug transport across the skin, the pharmacokinetics that determine how rapidly the drug is eliminated from the body and, of course, the pharmacological potency [Citation1,Citation2].
Creams and ointments have long been used to treat localized diseases but can be less effective for systemic therapy due to poor drug bioavailability. Furthermore, dosing can be a problem due to variation in the formulation application area. The development of transdermal therapeutic systems using different patch technologies, e.g. reservoir (clonidine, Catapres TTS®; Boehringer Ingelheim Pharmaceuticals, Inc., USA), matrix (rivastigmine, Exelon®; Novartis Pharmaceuticals Corporation) and drug-in-adhesive (fentanyl, Duragesic®; Janssen Pharmaceuticals, Inc.) designs, brought about the first ‘golden age’ of transdermal delivery [Citation1,Citation2]. However, there have been no new passive transdermal patch products approved since 2010.
The remarkable barrier function of the stratum corneum imposes strict selection criteria and few systemically acting drugs possess the requisite physicochemical properties and pharmacological potency to enable sufficient quantities to be delivered by passive diffusion through an intact stratum corneum in a therapeutically relevant time frame. In order to have more candidates for transdermal delivery, we can either modify drug physicochemical properties or develop new technologies that (i) increase the flux of ‘poor’ candidates or (ii) create new opportunities by expanding the range of molecules for delivery by this route (obviously, increasing potency would be an advantage as well). The use of new technologies means that it is highly likely that we will need to rethink the formulation strategies that have been used thus far.
2. Recent developments – what’s new?
The last 20–30 years have indeed seen the development of several techniques that have the potential to dramatically expand the horizons for transdermal administration – making the ‘undeliverable’ deliverable (). These methods employ different mechanisms, including (i) a second driving force, e.g. iontophoresis [Citation3], where a potential gradient is applied across an intact skin in addition to the concentration gradient to create an active transport process or, (ii) disruption of the stratum corneum and creation of new transport channels by mechanical (e.g. microneedles) or energy-based (e.g. fractional laser ablation) techniques – thereby, facilitating passive diffusion through these transient shunt pathways or (iii) application of energy to disrupt lipid organization and so render passive diffusion through an ‘intact’ stratum corneum more facile (e.g. electroporation and sonophoresis). Since molecules having physicochemical properties different from those of ‘conventional’ transdermal candidates can now be considered or different interfaces created for the partitioning step between the formulation and epidermis, it is reasonable to assume that new formulation approaches should be developed.
Figure 1. Physical techniques (a) iontophoresis: delivery of drugs containing ionizable groups, (b) electroporation: disruption of multilamellar lipid layer and permeabilization of skin followed by internalization of drug after treatment, (c) laser microporation: formation of micropores followed by passive diffusion from a patch to the deeper skin layers, (d) microneedle: insertion of drug coated microneedles into the skin, (e) sonophoresis, disruption of stratum corneum by microbubble assisted acoustic cavitation (skin layer thicknesses not drawn to scale).
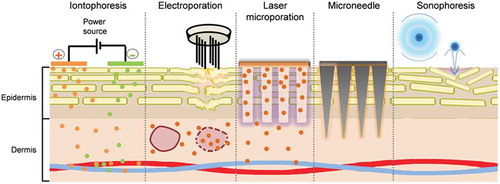
For example, the most important prerequisites for iontophoresis are that the drug should be ionized and water soluble; by definition, it opens the door to the transdermal administration of molecules that are unsuitable for passive delivery across the stratum corneum. The formulation requirements will clearly be different from those of lipophilic molecules delivered from conventional transdermal systems. However, uncharged and poorly water-soluble molecules can also be transformed into candidates for iontophoresis by suitable modulation of their physicochemical properties, e.g. synthesis of water-soluble prodrugs with ionizable moieties [Citation4]. Pre-filled iontophoretic therapeutic systems have been approved for lidocaine (LidoSite®; Vyteris Inc., 2004), fentanyl (Ionsys®; Alza Corp., 2006) and sumatriptan (Zecuity®; Teva Pharmaceuticals Inc., 2013). In addition, a reverse iontophoretic glucose monitoring system was approved in 2002 (GlucoWatch G2; Cygnus Inc.). Unfortunately, none of these products remained on the market for more than 3–4 years although the fentanyl system has recently been re-launched. In the case of Zecuity®, the product was recently withdrawn due to application site reactions, highlighting the fact that formulation/device design must take into account the risk of local current- and drug-induced dermal irritation; the latter might also be addressed, if necessary, by co-formulation strategies [Citation5].
In contrast to iontophoresis, electroporation involves application of high voltage pulses of 50–500 V for a few milliseconds to perturb lipid organization in the stratum corneum (electrical resistance drops by several orders of magnitude) and so facilitate passive diffusion. Electrochemotherapy, the most successful clinical application of electroporation, is used for the treatment of (sub)cutaneous tumor nodules in malignant melanoma patients [Citation6]. Following either intravenous or intratumoural injection of bleomycin or cisplatin, electric pulses increase the permeability of tumor cells, facilitate drug uptake and thus potentiate antitumor activity.
The stratum corneum is the principal diffusional barrier to molecular transport; therefore, another approach is to simply remove it – preferably, in a minimally invasive manner – thereby (i) facilitating passive diffusion of low-molecular-weight drugs and (ii) enabling the transdermal delivery of biomolecules. It should be noted that since the skin barrier is compromised, formulations may need to be manufactured under sterile conditions. In fractional laser ablation, a micropore array is created through the focused application of energy at a given wavelength and the depth of each micropore is dependent on the applied fluence. It is possible to remove selectively the stratum corneum or the entire viable epidermis and even create micropores that reach the deep dermis. This creates a different interface for drug transfer and the rheological properties of the formulation must enable it to enter the micropores so that partitioning can occur in the transport conduits. Fractional ablation has the advantage over ‘bulk ablation’ that the presence of regions of intact skin between the pores facilitates skin healing and pore closure typically occurs within 48–72 h; therefore, it can be advantageous to create intraepidermal drug depots for sustained drug release from within the skin after micropore closure [Citation7].
Microneedles are micron-scale devices that enable mechanical perforation of the skin barrier; the aim is to penetrate the stratum corneum and epidermis without reaching the dermis and its pain-sensitive nerve endings [Citation8]. They can be divided into four categories: (i) solid microneedles that are used to pretreat the skin in a ‘two-step’ approach prior to formulation application (similar to fractional laser ablation), (ii) drug-coated microneedles that enable rapid drug delivery to the epidermis since the drug is present on the microneedle surface, (iii) dissolving microneedles where the drug is formulated/engineered into the microneedle and (iv) hollow microneedles which are in contact with a drug reservoir and which function in the same way as a conventional needle. Coated microneedles have been developed for the delivery of low molecular weight compounds (e.g. lidocaine; sMTS drug delivery system, 3M Drug Delivery Systems), peptides (e.g. human parathyroid hormone (hPTH(1–34)); ZP Patch Technology, Zosano Pharma Corp.) and vaccines (NanopatchTM; Vaxxas Pty Ltd.). The development of sMTS and Nanopatch systems is ongoing, but the daily hPTH(1–34) ZP patch was discontinued in 2015 [Citation9]; however, the company recently announced that outcomes from a phase III trial into the delivery of zolmitriptan met both primary endpoints. Dissolvable microneedles have been developed by Corium Inc. (MicroCor® PTH(1–34)) and BioSerenTach Inc. (lixisenatide) but are still at an early stage [Citation9]. Commercialization of products might be hindered by manufacturing costs, formulation development and stiff competition from intradermal injectors already in the market (e.g. the first intradermal influenza vaccine (Intanza®/IDflu®) was launched and marketed in Europe by Sanofi Pasteur in 2009 using the intradermal BD Soluvia™ injection system (Becton, Dickinson and Company)) [Citation8,Citation9].
The physical methods described above rely on improving diffusion kinetics in the skin and formulation design must address the needs of the different molecules that can be used and/or the new interface between the formulation and the skin. Other formulation approaches can be used to influence the thermodynamic aspects of drug delivery – i.e. partitioning from the vehicle to the skin. In this context, nanocomposites have been studied for epidermal, dermal and systemic delivery; the treatment of dermatological conditions is most promising since it allows for targeted delivery to skin structures and the avoidance of off-target effects. Current efforts in topical drug delivery are focused on the development of nano-sized and micro-structured drug delivery systems [Citation10]. Nanoemulsions, liposomes, solid lipid nanoparticles, nanostructured lipid carriers, polymeric nanoparticles and micelles have proven their ability to deliver molecules to cutaneous tissue and notably target hair follicles [Citation10]. However, the underlying mechanisms are still unknown: although it is highly unlikely that intact nanocarriers are able to cross intact stratum corneum this is still a matter of debate. There is strong evidence that nanocomposites may break up upon skin contact or be preferentially deposited in inter-cluster regions (skin furrows) and appendages – increased local drug concentrations (i.e. elevated thermodynamic activity) and improved skin coverage may facilitate drug partitioning to the skin. The various hypotheses are summarized in .
Figure 2. Putative drug delivery mechanisms of nano-sized systems. Adapted with permission from [Citation10].
![Figure 2. Putative drug delivery mechanisms of nano-sized systems. Adapted with permission from [Citation10].](/cms/asset/85b82c7c-693f-4570-8920-50f0cddb6e57/iedd_a_1311320_f0002_c.jpg)
We have already witnessed the arrival on the market of ‘nano-formulations’ of therapeutic agents that exert both local and systemic effects: (i) estradiol in a micellar emulsion (Estrasorb™, Novavax Inc.), (ii) econazole nitrate in liposomes (Pevaryl® Lipogel, Janssen Pharmaceuticals Inc.) and (iii) tretinoin in polymeric microspheres (Retin A® Micro, Valeant Pharmaceuticals Inc.) – and other products are in clinical development. A liposomal lotion containing T4N5 (a bacterial DNA repair enzyme) seemed to lower the occurrence rate of new actinic keratosis in xeroderma pigmentosum patients when applied for 1 year [Citation11] and received an FDA fast-track designation for the treatment of photosensitivity in xeroderma pigmentosum patients in 2007. A nitroglycerin microemulsion developed by MediQuest Therapeutics Inc. (Seattle, USA) presented a 13% improvement over placebo in patients presenting with Raynaud’s disease [Citation12]. Recent phase III trials have indicated the efficacy of nanoemulsion formulations of (i) 5-aminolevulinic acid for the treatment of actinic keratosis [Citation13] and (ii) NB-001 for the treatment of herpes labialis [Citation14] (NanoBio Corp., Ann Arbor, USA) in comparison to placebo. An additional phase II trial is yet to start recruiting to test the topical administration of amphotericin B in a liposomal gel for the treatment of cutaneous leishmaniasis [Citation15].
3. Expert opinion
Recent years have witnessed the development of innovative transdermal drug delivery systems – including technologies employed to breach the skin barrier – that are still evolving as we seek to improve efficacy, practicality and safety. The feasibility of using these techniques has been demonstrated in preclinical studies, clinical trials and, in some cases, by the approval and marketing of new products. However, successful proof-of-principle studies and the demonstration of feasibility even in human subjects do not guarantee the successful development and commercialization of a new product. This can sometimes depend more on business-related factors than scientific/technical excellence and innovation. Furthermore, development can also stall because there is a reluctance to take ‘perceived’ risks with new drug delivery strategies – therefore, the arguments need to be compelling. This inertia might be overcome by new products that clearly demonstrate superiority over existing alternatives – not just in terms of patient compliance and ease of use but with respect to efficacy and the creation of new therapeutic options for the clinician. One approach to convince the skeptics would be to develop more ‘cost-effective/delivery-efficient’ formulations; ‘off-the-shelf’ solutions, originally developed for more ‘conventional’ molecules, are being used far too often. Little work has been done on the rational design of formulations optimized for use with the technologies and with the rather different types of therapeutic agent that can now be envisaged for transdermal delivery. Excipients and formulations used currently have been selected for the delivery of moderately lipophilic drugs with poor aqueous solubility into intact skin. Although usable, they may not be ideal for formulating the types of therapeutic agents that can be considered for delivery, for example, with the new minimally-invasive methods – is it realistic to expect that excipients designed to deliver estradiol into intact skin should be effective for the delivery of a protein into microporated skin following fractional laser ablation or the application of solid microneedles? Formulation development will be key to the successful exploitation of these new technologies since the formulation (i) ensures the stability and activity of the therapeutic agent – e.g. for biotechnology-derived drugs, (ii) determines the interface with the skin and so must be adapted to facilitate drug partitioning – e.g. into microporated skin and (iii) optimizes drug delivery efficiency so as to maximize the fraction of drug content delivered to the body and to minimize the amount retained within the formulations. Therefore, more effort must be focused on formulation design and development so that the potential of the new delivery technologies can be fully realized.
Declaration of interest
The authors have no relevant affiliations or financial involvement with any organization or entity with a financial interest in or financial conflict with the subject matter or materials discussed in the manuscript. This includes employment, consultancies, honoraria, stock ownership or options, expert testimony, grants or patents received or pending, or royalties.
Additional information
Funding
References
- Perumal O, Murthy SN, Kalia YN. Turning theory into practice: the development of modern transdermal drug delivery systems and future trends. Skin Pharmacol Physiol. 2013;26:331–342.
- Pastore MN, Kalia YN, Horstmann M, et al. Transdermal patches: history, development and pharmacology. Br J Pharmacol. 2015;172:2179–2209.
- Kalia YN, Naik A, Garrison J, et al. Iontophoretic drug delivery. Adv Drug Deliv Rev. 2004;56:619–658.
- Chen Y, Alberti I, Kalia YN. Topical iontophoretic delivery of ionizable, biolabile aciclovir prodrugs: a rational approach to improve cutaneous bioavailability. Eur J Pharm Biopharm. 2016;99:103–113.
- Cormier M, Chao ST, Gupta SK, et al. Effect of transdermal iontophoresis codelivery of hydrocortisone on metoclopramide pharmacokinetics and skin-induced reactions in human subjects. J Pharm Sci. 1999;88:1030–1035.
- Marty M, Sersa G, Garbay JR, et al. Electrochemotherapy – an easy, highly effective and safe treatment of cutaneous and subcutaneous metastases: results of ESOPE (European Standard Operating Procedures of Electrochemotherapy) study. EJC Suppl. 2006;4:3–13.
- Singhal M, Del Río-Sancho S, Sonaje K, et al. Fractional laser ablation for the cutaneous delivery of triamcinolone acetonide from cryomilled polymeric microparticles: creating intraepidermal drug depots. Mol Pharm. 2016;13:500–511.
- Gratieri T, Alberti I, Lapteva M, et al. Next generation intra- and transdermal therapeutic systems: using non- and minimally-invasive technologies to increase drug delivery into and across the skin. Eur J Pharm Sci. 2013;50:609–622.
- Watkinson AC, Kearney M-C, Quinn HL, et al. Future of the transdermal drug delivery market–have we barely touched the surface? Expert Opin Drug Deliv. 2016;13:523–532.
- Roberts MS, Mohammed Y, Pastore MN, et al. Topical and cutaneous delivery using nanosystems. J Control Release. 2017;247:86–105.
- Yarosh D, Klein J, O’Connor A, et al. Effect of topically applied T4 endonuclease V in liposomes on skin cancer in xeroderma pigmentosum: a randomised study. Xeroderma Pigmentosum Study Group. Lancet. 2001;357:926–929.
- Chung L, Shapiro L, Fiorentino D, et al. MQX-503, a novel formulation of nitroglycerin, improves the severity of Raynaud’s phenomenon: a randomized, controlled trial. Arthritis Rheum. 2009;60:870–877.
- Reinhold U, Dirschka T, Ostendorf R, et al. A randomized, double-blind, phase III, multicentre study to evaluate the safety and efficacy of BF-200 ALA (Ameluz((R))) vs. placebo in the field-directed treatment of mild-to-moderate actinic keratosis with photodynamic therapy (PDT) when using the BF-RhodoLED((R)) lamp. Br J Dermatol. 2016;175:696–705.
- Kircik L, Jones TM, Jarratt M, et al. Treatment with a novel topical nanoemulsion (NB-001) speeds time to healing of recurrent cold sores. J Drugs Dermatol. 2012;11:970–977.
- ClinicalTrial.gov. Topical liposomal amphotericin B gel treatment for cutaneous leishmaniasis-NCT02656797. 2016 [cited 2017]. Available from: www.clinicaltrials.gov/ct2/show/NCT02656797.