1. Introduction
Cancer ranks amongst the most deadliest diseases, associated with significant burden, often fatal prognosis and huge clinical challenge [Citation1]. Cancer management has substantially improved mainly on cancer diagnostics and therapeutics, resulting in extended survival rates and decreased mortality worldwide. Chemotherapy, along with radiotherapy and surgery, represents one of the major therapeutic approaches for cancer cells eradication, however associated with several shortcomings, as poor cancer tissue biodistribution, accelerated drug degradation and clearance, insufficient cancer targetability and still high systemic toxicity, overall compromising efficacy and safety [Citation2,Citation3].
Nanotechnologies are being widely explored to increase drug efficacy through site specific targeted drug delivery, promoting drug accumulation within cancer tissues and minimizing systemic adverse effects [Citation4]. In the past, this was thought to occur passively through the enhanced permeability and retention (EPR) effect, however it is now understood that the marked cancer heterogeneity and intratumoral environment greatly compromise the ability of nanomedicines to accumulate through the EPR effect. Although nanosystems can be harnessed to incorporate targeting ligands for active targeted delivery with enhanced selectivity to target cancer cells, ligand coupling processes are often laborious, expensive and complex.
Bioinspired approaches that attempt to mimic biological structures and their interactions have been shown to improve the biocompatibility, efficacy and safety of nanosystems by promoting controlled cell-surface communications. Cell membrane-coated nanosystems consisting of biomimetic attributes can maximize drug targeting by exploiting the inherited biofunctionalities of cell membranes extracted from source cells [Citation2]. Particularly, cancer cell membrane (CCM) coatings preserve the source targeting abilities imparted by a repertoire of cell adhesion molecules, providing CCM-coated nanosystems (CCM-NS) with additional cancer-homing features via a homotypic targeting mechanism [Citation5]. Additionally, the molecularly rich surface of CCM coatings enable surface exposition of cancer-specific antigens, capable of eliciting multiantigen responses with relevant applications in anticancer vaccination and immunotherapy [Citation5,Citation6].
2. Pharmaceutical applications of CCMs
The multifunctional surface of native CCMs can be successfully used to endow nanoparticle cores with surface coatings containing a wide molecular repertoire, which includes: ‘self-markers’ (e.g., CD47) that avoid the recognition by immune cells, self-recognition molecules (surface adhesion galectin-3, E-cadherin and tissue factor-antigen) that facilitate homotypic adhesion and binding to source cancer cells, and cancer-specific antigens [Citation2,Citation3,Citation7].
The CCM-NS preparation involves: (1) membrane extraction and membrane vesicles isolation; (2) preparation of the nanoparticle core, and (3) fusion of the nanoparticle core with the CCM vesicles [Citation3,Citation5]. Several methods for preparing CCM-NS have been reported so far. The most common method involves CCMs extraction from source cells via hypotonic lysis, followed by homogenization, centrifugation and purification steps yielding CCM vesicles. The size of the vesicles can be tailored and optimized using membrane extrusion. The coating of nanoparticle cores can be carried out by either a co-extrusion or sonication process, thus assembling CCM-NS [Citation5].
The versatility of the nanoparticle cores – both in composition and features – enables the encapsulation of a variety of compounds (drugs, nucleic acids, photosensitizers, imaging components), aiming at cancer therapy and cancer theranostics () [Citation2,Citation5]. So far, several chemotherapy drugs (e.g., doxorubicin, paclitaxel, camptothecin, cisplatin) have been successfully guided to malignant lesions by CCM-NS with improved anticancer effects when compared to non-coated counterparts [Citation1,Citation2,Citation5,Citation6]. The augmented performance is ascribed to: (1) improved targeted drug delivery and (2) enhanced cellular uptake, resulting from homotypic binding features of surface cell adhesion components, which favors (3) site-specific accumulation in cancer tissues, also aided by (4) prolonged blood circulation time.
Figure 1. Schematic illustration of the main modalities of cancer cell membrane-coated nanosystems (CCM-NS) applications in cancer management, namely cancer therapy, cancer imaging and cancer theranostics. The different modalities include cancer-targeted (A) drug delivery (e.g., chemotherapy); (B) multiantigenic cancer vaccine (antigen-exposing surface and combination with immunoadjuvants); (C) immunotherapy (loading or surface coupling of monoclonal antibodies); (D) phototherapy, either photothermal therapy (loading of heat-generating components) or photodynamic therapy (loading of ROS e-generating photosensitizers); (E) gene delivery (loading of nucleic acids); and (F) imaging, for enhanced cancer diagnostics and bioimaging. Different modality combinations expand the multifunctionality and impart additional therapeutic performance to CCM-coated nanosystems
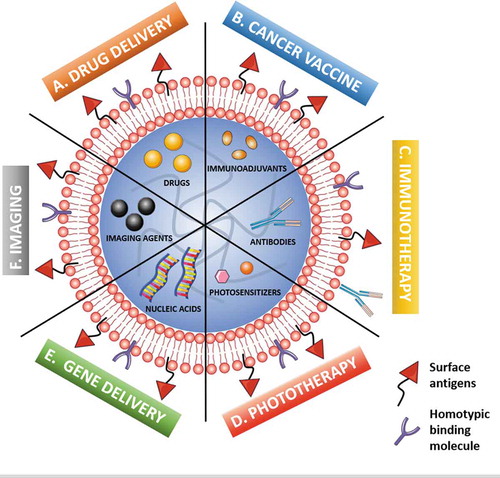
The tendency is to include more than one therapeutic agent to accomplish synergistic anticancer therapies, or to combine drugs with imaging agents for cancer theranostics. The combination of different payloads to create multimodal cancer-targeted CCM-NS has been shown to significantly improve anticancer effects when compared to the correspondent monotherapies and non-coated counterparts. For instance, the combination of photothermal therapy (PTT) and chemotherapy in a single CCM-NS enabled excellent ablation of cancer cells as well as photoacoustic imaging properties [Citation8]. The co-loading of doxorubicin and indocyanine green dye in hollow copper sulfide melanoma cell membrane (B16F10 cell)-coated nanoparticles improved in vitro homologous targeting ability to the source cancer cells, and revealed notorious in vivo self-recognition to the melanoma cells with minimal toxicity, exhibiting superior anticancer activity when compared to the respective non-coated nanoparticles [Citation8]. In fact, the PTT component often comprises a dual function, serving as both heat-generating and imaging component. Also, the amplification of chemotherapy via cancer-targeted starvation therapy by reducing glucose availability to cancer cells has recently been reported. Doxorubicin-loaded metal organic frameworks coated with glucose oxidase (GOx)-inserted lung CCM (4T1 cell) displayed glycolytic activity and functioned as a cascade nanoreactor, showing enhanced intracellular doxorubicin release and GOx-mediated glucose depletion [Citation9]. The cancer-homing features conveyed by CCMs can also benefit the intracellular uptake of photosensitizers, and therefore expand their ROS-mediated cytotoxicity to cancer cells upon light irradiation. As an example, gastric CCM (SGC7901 cell) has been demonstrated to improve the cancer photodynamic therapy of silica nanoparticles loaded with chlorin e6 [Citation10]. These encouraging findings open the field to many different drug/photosensitizer combinations, building on decades of monotherapy-based investigations, giving huge scope for improvement in future therapies.
The precision and efficiency of CCM coating technology hold increasing potential toward enhanced cancer targeting and maximized nucleic acid delivery, either isolated or in combined therapies. Recently, programmed death‐ligand 1 (PD-L1) siRNA (siPD-L1) and doxorubicin co-loaded homotypic (HeLa) CCMs-coated PLGA nanoparticles improved in vitro cellular internalization and PD-L1 knockdown when compared to the non-coated and heterotypic (MDA-MB-231) CCMs-coated PLGA nanoparticles, owing to the homotypic cancer targeting features conferred by the surface-exposed proteins of HeLa CCM-NS [Citation11].
Cancer imaging is a key modality of cancer detection, being widely employed to monitor tumor progression and spread. Nevertheless, difficulties with site specificity or biocompatibility are common. The homotypic targeting and immune-evading properties of CCM-NS can be exploited to improve the in vivo performance of imaging agents. For instance, the surface functionalization of Ag2Te quantum dots-loaded PLGA nanoparticles with 4T1 CCMs significantly improved both the in vivo targetability to source cancer cells and NIR-II fluorescence intensity in cancer tissues [Citation12]. As more knowledge is gained with the complexities of cancer physiology and tumor microenvironments, increasingly image guided technologies are being developed. CMM coating can allow further protection to the incorporated imaging agent which will ultimately lead to more advanced nanosystems in the near future.
The applicability of CCM-NS as highly-specific biomimetic anticancer nanovaccines is underpinned by the natural immunogenicity imparted by the surface exposition of cancer-specific antigens [Citation6]. The coated nanoparticle core can function as a drug or immunoadjuvant reservoir to extend the cancer vaccine potential by boosting immune responses [Citation5,Citation6]. Particularly, imiquimod -loaded PLGA nanoparticles were coated with mannose-decorated murine melanoma cell membrane (B16-OVA cell) for improved antigen presenting cells (APCs) targeting. The CCM-NS showed relevance toward clinical translation attested by the ability to serve as either prophylactic or therapeutic cancer vaccine solely, or by synergistic combination with immune checkpoint inhibitors (e.g., anti-programmed cell death protein 1 (anti-PD-1)) [Citation13].
3. Expert opinion
CCM-NS exhibit exciting features for improved cancer-targeted therapies, namely by promoting: (1) the development of personalized treatment (once patient-specific CCM can be obtained from source cancer cells), which may be of utmost interest for addressing cancer heterogeneity resulting from the coexistence of multiple cell types with diverse phenotypes, and improve the treatment’s patient-by-patient specificity; (2) biocompatibility and safety, owing to the endogenous source of CCMs; (3) improved immune system evasion and prolonged blood circulation; (4) ideal cancer-homing features and enhanced interaction with source cells and targeting abilities obtained by a top-down technological approach; and (5) ease of preparation.
The cancer targeting scope of CCM-NS includes not only primary cancer targeting, but also the metastatic scenario [Citation1]. Tailoring the surface of CCM-NS toward active targeting strategies by attaching selected ligands is receiving increasing relevance to maximize cancer tissue accumulation and uptake [Citation1–3,Citation5]. Cell engineering may allow tuning CCMs composition for improved outcomes, such as expressing the co-stimulatory signal CD80, as recently reported [Citation14].
Personalized CCM-NS for anticancer vaccination containing patient-derived CCMs may open a new avenue toward improved specificity and efficacy of immune responses for cancer cell eradication [Citation6]. However, several challenges may persist associated with cancer heterogeneity – including the low immunogenicity of certain cancer types and the typical immunosuppressive cancer microenvironment – and the ultimate endogenous provenance of CCMs which may hinder immune-mediated anticancer responses (more pronounced in older patients owing to weaker immune responses).
Also, the combination of CCMs with other distinct cell membranes, yielding multifunctional hybrid cell membranes, may synergistically associate the self-recognition features of CCMs with additional biofunctionalities [Citation15]. This has been applied to develop cancer-erythrocyte hybrid cell membrane-coated nanosystems to convey two-in-one cancer-targeted and immune-escaping nanosystems [Citation2,Citation15].
The manufacture costs and scale-up logistics are critical points to deeply consider, and those should certainly overcome additional barriers concerning the quality control and regulation. The scale-up process must assure the reproducibility of CCM-NS, which constitutes a challenge owing to: (1) the complexity of the CCMs; (2) the often multicomponent nature of CCM-NS; (3) the necessity of high amounts of CCMs; (4) the several production steps; (5) the self-life of the CCM-NS; and (6) potential batch-to-batch variation [Citation5]. A thorough patient stratification accounting on the genetic and histological landscape may help to find specific patients segments which may benefit more from the treatment, and to fully explore CCM-NS as next-generation cancer therapeutics.
Declaration of interest
The authors have no relevant affiliations or financial involvement with any organization or entity with a financial interest in or financial conflict with the subject matter or materials discussed in the manuscript. This includes employment, consultancies, honoraria, stock ownership or options, expert testimony, grants or patents received or pending, or royalties.
Reviewer disclosures
Peer reviewers on this manuscript have no relevant financial or other relationships to disclose.
Additional information
Funding
References
- Gong X, Li J, Tan T, et al. Emerging approaches of cell-based nanosystems to target cancer metastasis. Adv Funct Mater. 2019;29(48):1903441.
- Fang RH, Kroll AV, Gao W, et al. Cell membrane coating nanotechnology. Adv Mater. 30(23): e1706759. 2018.
- He Z, Zhang Y, Feng N. Cell membrane-coated nanosized active targeted drug delivery systems homing to tumor cells: A review. Mater Sci Eng C Mater Biol Appl. 2020;106:110298.
- Conde J. Above and beyond cancer therapy: translating biomaterials into the clinic. Trends Cancer. 2020. DOI:10.1016/j.trecan.2020.04.008
- Harris JC, Scully MA, Day ES. Cancer cell membrane-coated nanoparticles for cancer management. Cancers (Basel). 2019;11(12):1836.
- Zhou J, Kroll AV, Holay M, et al. Biomimetic nanotechnology toward personalized vaccines. Adv Mater. 2020;32(13): e1901255.
- Janiszewska M, Primi MC, Izard T. Cell adhesion in cancer: beyond the migration of single cells. J Biol Chem. 2020;295(8):2495–2505.
- Wu M, Mei T, Lin C, et al. Melanoma cell membrane biomimetic versatile CuS nanoprobes for homologous targeting photoacoustic imaging and photothermal chemotherapy. ACS Appl Mater Interfaces. 2020;12(14):16031–16039.
- Cheng H, Jiang X-Y, Zheng -R-R, et al. A biomimetic cascade nanoreactor for tumor targeted starvation therapy-amplified chemotherapy. Biomaterials. 2019;195:75–85.
- Yang J, Teng Y, Fu Y, et al. <p>Chlorins e6 loaded silica nanoparticles coated with gastric cancer cell membrane for tumor specific photodynamic therapy of gastric cancer. Int J Nanomedicine. 2019;14:5061–5071.
- Chen M, Chen M, He J. Cancer cell membrane cloaking nanoparticles for targeted co-delivery of doxorubicin and PD-L1 siRNA. Artif Cells Nanomed Biotechnol. 2019;47(1):1635–1641.
- Zhang JJ, Lin Y, Zhou H, et al. Cell membrane-camouflaged NIR II fluorescent Ag2Te quantum dots-based nanobioprobes for enhanced in vivo homotypic tumor imaging. Adv Healthc Mater. 2019;8(14):e1900341.
- Yang R, Xu J, Xu L, et al. Cancer cell membrane-coated adjuvant nanoparticles with mannose modification for effective anticancer vaccination. ACS Nano. 2018;12(6):5121–5129.
- Jiang Y, Krishnan N, Zhou J, et al. Engineered cell-membrane-coated nanoparticles directly present tumor antigens to promote anticancer immunity. Adv Mater. 2020;32(30):e2001808.
- Chen H-Y, Deng J, Wang Y, et al. Hybrid cell membrane-coated nanoparticles: A multifunctional biomimetic platform for cancer diagnosis and therapy. Acta Biomater. 2020;112:1–13.