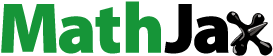
ABSTRACT
Introduction: The most widely used pharmacological treatment for Parkinson’s disease is levodopa, the precursor for dopamine formation in the brain. Over time, the effectiveness of levodopa declines, and patients experience motor fluctuations, or OFF periods. A levodopa formulation administered via a capsule-based oral inhaler provides a new delivery mechanism for levodopa that provides rapid relief of OFF periods.
Areas covered: CVT-301 is a dry powder formulation designed to supply levodopa to the systemic circulation via pulmonary absorption. The technology, pharmacokinetics, efficacy, and safety data of this formulation are presented.
Expert opinion: Oral inhalation is a novel method of administration for levodopa that bypasses the gastrointestinal tract, allowing levodopa to enter the systemic circulation rapidly and more reliably than oral medications. Gastrointestinal dysfunction, a common feature of Parkinson’s disease, can lead to impaired absorption of oral medications. Pulmonary delivery rapidly elevates levodopa plasma concentrations to provide relief of OFF periods for patients receiving oral levodopa.
1. Overview of the market: OFF periods and Parkinson’s disease
Although treatment with levodopa provides the most effective long-term symptomatic therapy for Parkinson’s disease (PD) [Citation1], many patients find that this drug declines in consistency of benefit after a few years of use. Levodopa is a neutral amino acid that serves as a precursor for dopamine synthesis in the brain. After oral administration, its uptake is through a facilitated transport mechanism in the duodenum and proximal jejunum, and it subsequently crosses the blood–brain barrier to reach the neuronal areas where dopamine is synthesized [Citation2]. After a few years of levodopa treatment, high variability of levodopa plasma concentration and compromise of the gastrointestinal (GI) absorption process often become the major impediments to this drug providing reliable control of PD symptoms and managing motor and non-motor fluctuations.
One of the factors impacting levodopa absorption is the presence of gastroparesis and delayed gastric emptying [Citation3,Citation4]. Delayed gastric emptying has been reported in 70–100% of PD patients and the symptoms or gastroparesis, such as bloating, nausea, and early satiety, have been reported in 25–45% [Citation5]. Gastroparesis and delayed gastric emptying affect the passage of levodopa through the stomach and may therefore delay absorption by slowing or preventing its transit to the proximal small intestine, where it is absorbed [Citation6,Citation7]. The longer the time that levodopa spends in the stomach and small intestine, the more it is catabolized by the enzymes DOPA decarboxylase and catechol-O-methyltransferase, which decreases its availability for uptake [Citation8]. Helicobacter pylori infection may also be a factor as it is a risk factor for worse PD outcomes and poor response to oral levodopa treatment [Citation9,Citation10]. In addition, solid food delays gastric emptying, and neutral amino acids compete with levodopa for active transport in the small intestine, so when to time taking an oral dose with respect to eating meals is important [Citation11].
While several levodopa products have been devised to enhance duration of its uptake and hence, a more consistent clinical effect, the need for rapid onset of symptom relief for patients experiencing erratic symptom control has been wanting. With an inhaled formulation of levodopa (CVT-301, Inbrija®, Acorda Therapeutics) that was approved for the treatment of OFF periods in 2018, a treatment is now available to ensure that the therapeutic effect of levodopa can be achieved faster and more reliably than through oral administration alone. In this report, we describe the technology behind CVT-301, which has become a marketed on-demand product for PD patients who experience OFF periods or OFF episodes [Citation12,Citation13].
2. How the technology works: inhaled therapy
The pulmonary route of drug delivery is usually employed to achieve local action of the drug or the needs of systemic drug delivery, primarily by overcoming disadvantages of oral delivery including poor solubility of the drug, oral bioavailability, and the desire to avoid first-pass metabolism [Citation14].
Nebulizers have been available since the beginning of the twentieth century for pulmonary drug delivery. Most inhaled proteins for clinical development have been developed as liquids for use in nebulizers. Modern inhaler therapy has its origins in treating asthma with pressurized metered-dose inhalers (pMDIs) [Citation15,Citation16]. The commercial dominance of pMDIs was first challenged when chlorofluorocarbon (CFC) propellants were subject to international control and ultimately phase-out through the Montreal Protocol in 1987 [Citation17]. The difficulty of reformulation of inhaled drugs for alternative propellants and the concurrent demand for alternative delivery systems for the products of biotechnology prompted development and use of dry powder inhalers (DPIs) [Citation16].
DPI technology has evolved since the first modern devices appeared more than 50 years ago [Citation18]. Historically, the approach to inhaler design was to optimize the formulation with respect to device performance in terms of the energy (pressure drop and airflow) required to overcome the forces of interaction between the particles [Citation19–21]. Particle interaction can be attributed to capillary, electrostatic and van der Waals forces, and mechanical interlocking due to surface asperities [Citation21]. Capillary and electrostatic forces and interlocking can be modulated to some degree in lactose blends by controlling physicochemical properties (density, hygroscopicity, crystal form, particle morphology, and size) and environmental conditions [Citation22]. The majority of drugs intended to treat asthma and chronic obstructive pulmonary disease are potent agents effective in low doses, often ≤100 μg. These are blended as micronized, respirable particles into large quantities (>10 mg/dose) of lactose. The latter acts as a diluent and dispersion aid [Citation19,Citation21]. However, challenges to control of particle shape, forces of interaction, and responsiveness to airflow continue to cause inefficiency of aerosol generation from these devices, and this has limited delivery to ≤1 mg doses of active ingredient. High-dose delivery (>10 mg drug) from a device requires a particle engineering strategy that allows the drug particles to resist interaction and to disperse independently of device function [Citation21].
Many drugs are delivered from hydrofluoroalkane propellant-based systems that have recently replaced ozone depleting CFC propellants. The efficiency of delivery is rarely above 50%, despite the slower emission velocity of new propellants. Generally, these inhalers are restricted to low-dose delivery due to physicochemical limitations on drug dispersion in nonpolar propellants and the limited number of successful formulation strategies that are available to overcome this.
Generally, scientists have attempted to address the issue of aggregation in DPIs and dose limitation of pMDIs by developing inhalers that could break apart particle aggregates or have other features. One of the authors (RSL), along with David Edwards and other colleagues, took a radically different approach by changing aerosol particle geometry. Although prior work involved using small (~2 µm) diameter nonporous aerosols, we created large (5–20 µm), highly porous aerosols with extremely low densities. By lowering their density, we hypothesized that aerosol aerodynamics would be changed, making it possible for unusually large particles to enter the lungs through an airstream. We further hypothesized that increasing aerosol particle size would lead to decreased particle aggregation, creating greater inhalation efficiency, as well as decreased phagocytosis by lung macrophages. The decreased phagocytosis could potentially result in sustained drug release. We created such aerosols and found that more than 10 times the dose could be delivered this way compared with conventional aerosols and inhalers. The molecules also could be delivered to animals using these particles for more than 4 days from a single dose [Citation23]. This capability enables simple small inhalers that can contain as much as 70 mg of substance to be delivered in a single dose. Before this, the delivery of even 10 mg was generally not possible. Other forms of high-dose inhalers exist, including those for a precision spray-dried form of mannitol for the treatment of cystic fibrosis [Citation24,Citation25], and the polymyxin antibacterial colistin has been administered as dry powder for inhalation via a Turbospin inhaler [Citation26,Citation27].
The majority of inhalers and nebulizers are used for local delivery of drugs for asthma, chronic obstructive pulmonary disease and other lung diseases. Inhaler use for systemic drug delivery is much less common. Locally acting drugs for the treatment of asthma and chronic obstructive pulmonary disease are in the therapeutic categories of β2-adrenergic agonists and anticholinergics, which act on the sympathetic and parasympathetic nerves resulting in bronchodilatation, and glucocorticosteroids that treat the underlying inflammation. These potent low-dose drugs are delivered effectively by both pMDIs and DPIs.
Recently, an engineered dry particle strategy has been employed to achieve high-dose delivery (112 mg in 4 capsules) of tobramycin to treat Pseudomonas aeruginosa infection in cystic fibrosis patients [Citation28]. The most successful systemically active small-molecular-weight drugs have been those active against neurological disorders such as migraine (ergotamine and hydroxyergotamine) and psychosis (loxapine) [Citation29], so there is a clear opportunity for the use of engineered particles to deliver drugs for the systemic treatment of neurological disease.
CVT-301 was developed to address the need for treating episodes when PD symptoms can reemerge (OFF periods), despite a regular daily regimen of levodopa and other orally administered dopaminergic drugs. To accomplish this goal, CVT-301 utilizes the Arcus® dry powder delivery technology to deliver levodopa via particles suitable for inhalation with an aerodynamic diameter of 1–5 µm, to the pulmonary capillary bed via oral inhalation [Citation23,Citation30]. This strategy facilitates rapid uptake of levodopa into the systemic circulation, circumventing the major limitations associated with oral delivery of this drug. CVT-301 oral inhalation delivers a dose of levodopa that adds to the plasma concentration from the patient’s oral dose to bring plasma concentration of levodopa above the threshold necessary for enacting clinical effect. Patients are instructed to self-administer CVT-301 as soon as they start to experience OFF period symptoms. The inhaled dose is intended to elevate levodopa plasma concentration to a supra-threshold concentration so that brain dopamine synthesis increases and patients achieve reversal of an OFF episode until the next orally administered dose is absorbed. The device and the overall therapy concept underwent ‘human means’ testing, as reported to the United States Food and Drug Administration (FDA). Clinical experience has shown that CVT-301 achieves an onset of clinical benefit as soon as 10 minutes after administration, with clinically meaningful benefit seen by 30 minutes and this improvement lasts for at least 60 minutes after oral inhalation [Citation13].
CVT-301 is manufactured using a spray-drying process that produces particles with a relatively large geometric particle size, low mass density and small aerodynamic diameter, rendering them suitable for efficient dispersion and pulmonary deposition from a DPI. The DPI inhaler for CVT-301 consists of 4 major components; the chamber to hold the dose (capsule), the mechanism to pierce the capsule, the mouthpiece and the geometry of the flowpath that has been designed in a way to disperse the powder (). The inhaler is a high resistance device (~0.2) with a 4 kPa pressure drop across the inhaler at approximately 36 LPM. The system was determined to be robust across a wide range of flow rates and volumes (20 LPM/1 L up to 90 LPM/2 L) with system performance maintaining a ± 25% range around nominal. Thus, no specific statement is required for users in the CVT-301 Instructions for Use, regarding the flow rate that must be employed by a patient to use the CVT-301 system. CVT-301 has a nominal delivered dose of 33.4 mg levodopa (80% if calculated relative to the capsule fill weight of 42 mg levodopa), and a nominal fine particle mass of 25 mg levodopa (of particles less than 5.6 µm aerodynamic diameter per capsule), or 60% relative to the capsule fill weight of 42 mg levodopa or 75% relative to the nominal delivered dose of 33.4 mg levodopa.
Figure 1. Diagram of the Inbrija Inhaler
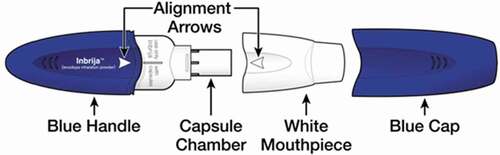
3. Clinical profile and post-marketing findings
Preclinical studies were initially conducted in multiple animal species to demonstrate proof of concept of this approach as well as to identify a formulation suitable for clinical and commercial development. Initial studies conducted in a rat model of PD demonstrated that the pulmonary administration of 2 mg of levodopa resulted in a rapid elevation of plasma concentrations, whereas oral levodopa administration results in a slower rise in plasma concentrations [Citation31]. Similar pharmacokinetic results were seen in beagle dogs, which also demonstrated that inhaled levodopa was absorbed in a more rapid and less variable manner compared with oral administration [Citation32]. Throughout these studies, formulations with increasing loads of levodopa were developed in order to account for expected human dosing levels and to provide for dosing with a maximum of 2 inhalations per dose. The clinical dose of levodopa was adjusted throughout the clinical program via varying the capsule fill weight and/or number of capsules administered per dose. An excipient in the inhaled formulation is dipalmitoyl phosphatidylcholine, the main endogenous constituent of pulmonary surfactant and crucial for lung function, whose function is to lower the surface tension in the alveoli [Citation33,Citation34].
The new drug application clinical development program for CVT-301 consisted of 11 clinical studies, including six phase 1, two phase 2 and three phase 3 studies (). The fine-particle dose (FPD) is the quantity of levodopa present in CVT-301 powder for inhalation that possesses an aerodynamic size considered small enough to reach the lungs and thus provides an estimate of the amount of levodopa deposited in the lungs. In this case, the particle size was defined as <5.6 µm for most studies (using an Andersen cascade impactor), although <5.4 µm was used for some studies, depending on the flow rate through the Andersen cascade impactor.
Table 1. Published studies in the CVT-301 clinical development program
In an initial phase 1 dose escalation study (10–50 mg FPD), 50 mg FPD levodopa resulted in similar mean peak plasma levodopa concentrations (Cmax = 884 ng/mL) compared with oral carbidopa/levodopa (25/100 mg) (Cmax = 637 ng/mL) with significantly less variability (expressed as percent coefficient of variation [CV]) than was seen for oral carbidopa/levodopa dosing in the fed state in healthy volunteers [Citation32]. For all inhaled doses, levodopa plasma concentrations increased more rapidly compared with oral carbidopa/levodopa doses in fasted or fed states [Citation32]. For example, the mean value for maximum levodopa concentration during the first 10 minutes (Cmax,10 min) as well as the area under the curve (AUC0-10 min) were much greater for the 50 mg FPD (Cmax, 10 min = 729 ng/mL, AUC0-10 min = 4824 ng·min/mL) than for fed state oral carbidopa/levodopa (Cmax, 10 min = 18 ng/mL, AUC0-10 min = 124 ng·min/mL) [Citation32]. All inhaled doses including 50 mg FPD were generally well tolerated [Citation32]. Five additional phase 1 studies were conducted in the CVT-301 clinical program for the purposes of confirming dose selection, reducing the number of capsules per dose to 2 capsules, comparing the pharmacokinetics and bioavailability of the commercial drug product to oral carbidopa/levodopa (Sinemet), and examining the pharmacokinetics and safety of CVT-301 in special populations (asthmatics and smokers).
With respect to phase 2 clinical studies in the target patient population, a single dose (25 or 50 mg FPD) in-clinic phase 2a study was initially conducted in PD patients 4 hours after their last meal and in the OFF state, to evaluate the pharmacokinetics, pharmacodynamics, safety, and tolerability of CVT-301 [Citation32]. Upon dosing, similar to the results seen in healthy volunteers, levodopa absorption was rapid, and levodopa exposure was dose proportional. In particular, CVT-301 administration was associated with a more rapid time to reach 50% of Cmax and time to Cmax (Tmax), with a fivefold greater AUC during the first 10 minutes postdosing of 50 mg FPD CVT-301 (mean adjusted levodopa concentration increased ∼500 ng/mL within 10 minutes) than was seen for oral carbidopa/levodopa administration, and the median Tmax was approximately 15 minutes for CVT-301 versus 66 minutes for oral carbidopa/levodopa. Therapeutic concentrations of levodopa were achieved within 10 min in ∼80% of subjects treated with CVT-301 50 mg FPD compared with 20% following oral carbidopa/levodopa. Variability in plasma levodopa concentrations (CV) was lower following CVT‐301 administration than with oral carbidopa/levodopa.
The pharmacodynamic outcomes of CVT-301 were investigated in a phase 2b efficacy study conducted with PD patients. In addition to timed finger tapping as a measure of anti-Parkinsonian motor response, investigator-assessed Unified Parkinson’s Disease Rating Scale Part III (motor examination) (UPDRS-III) [Citation35] was chosen to permit a more global objective measure of response against Parkinsonian signs. Motor responses following CVT‐301 35 and 50 mg FPD were dose‐ordered, with CVT‐301 50 mg FPD resulting in statistically significant improvements in timed tapping for average change during the first 15 and 30 minutes versus placebo. UPDRS-III scores showed significantly more improvement versus placebo for the average and best response for patients on CVT-301 50 mg FPD [Citation32]. A phase 1 pharmacokinetic study of CVT-301 in fed PD patients demonstrated that plasma levodopa concentrations rose more rapidly after a single dose of CVT-301 84 mg taken 4 to 5 hours after their previous routine oral carbidopa/levodopa dose than after they had taken a single dose of oral 25/100 mg carbidopa/levodopa. After CVT-301 dosing, mean levodopa plasma concentrations had increased to ~140 mg/mL after 5 minutes and to ~240 ng/mL by 15 minutes. In contrast, after the oral carbidopa/levodopa dose, no increase in plasma levodopa concentration was observed until 1 h post dose. Between-subject levodopa plasma concentration variability for CVT-301 84 mg was generally less than that for the oral carbidopa/levodopa dose. This was demonstrated by the smaller range of values and interquartile range (IQR) observed for the median Tmax for CVT-301 84 mg (15 min (range 5.0, 90.0 min, 25–75% IQR 10–30 min) compared with oral carbidopa/levodopa 25/100 mg Tmax of 120 min (range 57.0, 240 min 25–75% IQR 90–180 min). The study demonstrated that, by bypassing the GI tract, CVT-301 can raise levodopa plasma concentrations more rapidly than oral levodopa in fed PD patients [Citation36].
With respect to phase 3 clinical program, 3 phase 3 studies demonstrated the safety and efficacy of CVT-301 [Citation13,Citation37,Citation38]. The pivotal phase 3, randomized, double-blind, placebo-controlled, 12-week multicenter study of inhaled CVT-301 for the treatment of up to 5 OFF periods per day versus placebo (SPAN-PD study) resulted in a statistically significant achievement of the primary efficacy endpoint for the target 50 mg FPD commercial dose (reduction in UPDRS-III score versus placebo at 30 minutes) that also was supported by multiple secondary endpoints. In particular, the least squares mean UPDRS-III score change from predose to 30 minutes post dose was – 5·91 for the placebo group and – 9·83 for the CVT-301 84 mg group (between-group difference –3·92 P = 0.0088) [Citation13]. The percentage of patients who, at the 12-week assessment, had and maintained an ON response through 60 min was also statistically significant compared to placebo (58% and 36%, respectively). For the other secondary endpoints, treatment differences vs placebo for UPDRS-III score at 10 and 20 minutes postdose, Patient Global Impression of Change (PGIC) and OFF time were not statistically significant according to the hierarchical statistical analysis [Citation13]. Concerning safety, in support of the results seen in the phase 3 study described above, a 12-month, multicenter, observational cohort-controlled safety study found CVT-301 84 mg produced no clinically significant differences in pulmonary function (as measured by forced expiratory volume in 1 sec [FEV1], forced vital capacity [FVC], FEV1/FVC ratio, and diffusing capacity of the lungs for carbon monoxide [DLCO]) compared with the observational cohort. Improvements in UPDRS-III scores, OFF time, and patient-reported outcomes also supported the phase 3 clinical efficacy results described above during the entire 52 weeks of the study [Citation37]. With respect to adverse events (AEs), the most commonly reported AEs related to CVT-301 treatment were cough, dyskinesia, throat irritation, and discolored sputum. The most commonly observed AE was cough, which in the majority of cases was mild and did not prevent or impede dose administration [Citation37].
The safety profile of CVT-301 is thus consistent with the known safety profile of the referenced product, oral carbidopa/levodopa, with the addition of cough associated with inhalation of a dry powder. In particular, acute and chronic pulmonary safety evaluations showed no notable differences between treatment with CVT-301 compared with placebo or with an observational control population in spirometry parameters and DLco [Citation37,Citation38]. Evaluations of vital signs, clinical laboratory, and electrocardiogram showed no unusual safety signals. Inbrija is not recommended, however, in patients with asthma, COPD or other chronic underlying lung disease. A further safety and tolerability study showed that Inbrija could also be safely used as an adjunct treatment with oral carbidopa/levodopa for early morning OFF periods (or morning akinesia) [Citation39]. Overall, the clinical experience gained from the CVT-301 development program supports the efficacy of CVT-301 (84 mg) in the treatment of symptoms of OFF periods in PD patients with motor fluctuations.
4. Alternative technologies
Inbrija is the only levodopa product for treating PD OFF symptoms delivered by oral inhalation. Other pharmaceutical therapies for treating PD are administered orally by ingestion, subcutaneous injection, sublingually, or by intestinal pump. As described earlier, there are other drugs that are delivered via the pulmonary route to treat neurological disorders. Ergotamine and hydroxyergotamine are delivered from pMDIs, and loxapine is delivered from an evaporation condensation technology (Staccato®) [Citation29,Citation40].
5. Conclusion
CVT-301 was developed to address the need in PD patients for treating OFF periods that occur despite adjustment of conventional oral levodopa and use of adjunctive medication regimens. CVT-301 uses the Arcus dry powder delivery technology for delivering levodopa via oral inhalation, which facilitates rapid pulmonary capillary uptake of levodopa into the systemic circulation, circumventing the major limitations associated with irregular oral intake, such as delayed gastric emptying and GI dysfunction. CVT-301 is manufactured using a spray-drying process that produces particles with a relatively large geometric particle size, low mass density, and small aerodynamic diameter, allowing efficient delivery and pulmonary deposition from a DPI. CVT-301 oral inhalation delivers a dose of levodopa that supplements the levodopa plasma concentration provided by oral administration, exceeding the threshold necessary for a clinical effect. The inhaled dose elevates circulating levodopa concentration rapidly and with less variability than orally administered levodopa to achieve reversal of an OFF state.
6. Expert opinion
As PD progresses, oral levodopa absorption often becomes highly variable and/or delayed, and the duration of effectiveness for each dose can be brief [Citation1,Citation2,Citation41,Citation42]. This results in many patients experiencing daily dose-by-dose fluctuations in symptom control, despite standard medication regimens that previously provided more constancy of effect. OFF periods (motor fluctuations, when PD symptoms return due to delayed drug uptake or short duration of response) can also be joined by nonmotor symptoms [Citation41,Citation43,Citation44]. Both motor and nonmotor OFF periods often impose a major negative impact on patients’ quality of life, as they can make independent mobility and comfort unpredictable from day to day and even from hour to hour [Citation45–47]. Treatment of these symptoms remains an important clinical need for patients. The decline in levodopa efficacy is due in part to changes in GI uptake of the drug and to the continuing degeneration of dopaminergic neurons. Although PD results in diminished dopaminergic neurotransmission, the basis for motor fluctuations remains incompletely understood [Citation2,Citation41,Citation43]. However, irregular delivery of levodopa into the systemic circulation from the GI tract is a major component for many patients. When levodopa plasma concentrations are reduced after an oral dose, Parkinsonian features (i.e. OFF period symptoms) tend to return, often at intervals as short as 2 to 3 hours with immediate-release levodopa. For this problem, management strategies include use of sustained-release levodopa formulations, more frequent dosing, use of inhibitors of catechol-O-methyltransferase or monoamine oxidase-B, use of an adenosine A2a antagonist, or use of a levodopa/carbidopa intestinal gel, which is administered into the jejunum and duodenum at constant rates by a pump [Citation41,Citation48,Citation49]. In some patients, deep brain stimulation, a surgical procedure involving high frequency electrical pulsing into specific brain regions, can help at treating motor fluctuations [Citation50,Citation51].
Another factor to be considered is that orally ingested levodopa is affected by GI dysfunction that is common as PD progresses [Citation3,Citation52]. Levodopa is absorbed solely in the proximal small intestine. Delayed gastric emptying frequently occurs in PD patients, manifested as slowed or incomplete transport of levodopa distal to the pyloric sphincter and into the small intestine [Citation5,Citation52]. Meals also slow down gastric emptying, and dietary neutral amino acids compete with levodopa for the facilitated transport system in the gut [Citation4,Citation53]. These mechanisms may delay the absorption of oral levodopa and may contribute to delayed symptom control after ingesting an oral levodopa dose.
CVT-301 is effective for the intermittent treatment of OFF episodes in patients with PD who are currently treated with carbidopa/levodopa. Based on study data and the FDA indication, it can be taken up to 5 times a day. CVT-301 bypasses the GI tract and is absorbed faster than oral medication. This has been demonstrated in the clinical development program, which yielded findings showing Tmax of orally inhaled levodopa obtained in approximately 15 minutes as compared with oral carbidopa/levodopa Tmax (45 minutes to 2 hours, depending on fed or fasted state [Citation32,Citation36]). This rapidity of action is an advantage to patients seeking to bridge the gap between oral levodopa medication without experiencing OFF periods. Also, because the variability of levodopa plasma concentrations is much lower with CVT-301 due to its mode of uptake compared with oral levodopa, it offers a more consistent effect. Handling the inhaler device, placing the capsules in it and inhaling the product have generally not been problems in use of Inbrija. PD patients use the product not when completely immobile (i.e. fully OFF) but when they are just starting to experience a decline in PD control. As a result, the inhaled levodopa benefits arise in advance of when they are most needed. Levodopa has a well-understood safety profile. Currently, the only other FDA-approved drugs in the U.S. intended to serve as on-demand medications for OFF periods involve apomorphine. One of these products is subcutaneously injected (Apokyn®, US WorldMeds). Sublingual apomorphine strips (Kynmobi™, Sunovion Pharmaceuticals), absorbed through the oral mucosa, also bypasses the GI tract for uptake. Subcutaneous apomorphine can be used to help relieve early morning OFF (or morning akinesia) [Citation54,Citation55].
Reviewer disclosures
A reviewer on this manuscript has disclosed that they are employed by PureIMS, a company that develops an inhaled levodopa product that may eventually become a competitor of Inbrija. Peer reviewers on this manuscript have no other relevant financial relationships or otherwise to disclose.
Article highlights
CVT-301 is a drug and device combination product comprising capsules containing levodopa inhalation powder delivered using a breath-actuated inhaler device.
A spray-drying process allows for the manufacture of particles with a relatively large geometric particle size, low density, and small aerodynamic diameter that are suitable for efficient delivery and pulmonary deposition of relatively high doses of levodopa.
A clinical development program established that pulmonary administration of levodopa using a specially designed inhaler was safe and effective in treating OFF periods in patients with Parkinson’s disease when used adjunctively with a standard optimized oral carbidopa/levodopa treatment regimen.
CVT-301 increases plasma concentrations of levodopa rapidly and with lower variation compared to oral carbidopa/levodopa.
CVT-301 has no notable impact on pulmonary function in clinical trials.
The most frequent adverse event is cough that is usually transient and of mild-to-moderate severity.
Declaration of interest
MML was an employee and stockholder of Acorda Therapeutics at the time of the studies.
RL receives licensing fees (to patents in which he was an inventor on) from, invested in, consults (or was on Scientific Advisory Boards or Boards of Directors) for, lectured (and received a fee), or conducts sponsored research at MIT for which he was not paid for the following entities: 7th Sense, Abpro Labs, Abpro-Korea, Acorda (Formerly Civitas Therapeutics), Aleph Farms, Alivio Therapeutics, Alkermes, Allevi, Alnylam Pharmaceuticals, Inc, Apotex, Arcadia Biosciences, Inc, Arsenal Medical, Artificial Cell Technology, Inc, Avalon-Globocare, BASF Corporation, Bayer, Bilayer Therapeutics, Biogen, BioInnovation Institute (Novo Nordisk Founden), Blackstone (Formerly Clarus), Boston Children’s Hospital, Celero, Cellink, Cellomics Technology, Llc, Cellular Biomedical, Charles River Laboratories, Inc., Clontech Laboratories, Combined Therapeutics (‘CTx’), Conference Forum, Cornell University, Crispr Therapeutics Ag, Crown Bioscience Inc., Cygnal Therapeutics, Inc., Daré Biosciences (Formerly Microchips Biotech, Juniper Pharmaceuticals, and Columbia Laboratories), Daros, Inc., DeepBiome, Dewpoint Therapeutics, Dispendix Domain, Eagle Pharmaceuticals, Earli, Edigene Biotechnology, Inc., Editas Medicine, Inc., Eli Lilly, Eisai Inc., Entrega, EpiBone Establishment Labs, SA. Everlywell, Evox Therapeutics, Ltd., Flagship Pioneering, Frequency Therapeutics, Inc., Genemedicine Co Ltd, GenScript USA Inc, GENUV, GlaxoSmithKline LLC, Glycobia, Glympse Bio, Goldman Sachs, Greenlight Biosciences, HCR (HealthCare Royalty Partners), Hopewell Therapeutics, Horizon Discovery Group Plc, Humacyte, Inc., IBEX Pharmaceuticals, Inc., ImmuneXcite Inc., Indivor, Institute of Immunology Co. Ltd, Integrated DNA Technologies, Inc., InVivo Therapeutics, IxBio, J.R. Simplot Company, Jnana Therapeutics, Kala Pharmaceuticals, Kallyope, Inc., Kensa, Kodikaz Therapeutics, Ksq Therapeutics, Inc., Landsdowne Labs, LikeMinds, Lonza, Luminopia, Inc., Luye (Shandong luye), Lyndra Therapeutics, Lyra Therapeutics (Formerly ‘ Biomedical’), McGovern Institute, Medikinetics Co., Ltd., Merck, MGH Ragon Institute, Micelle, Moderna Therapeutics, Momenta, Muse Biotechnologies Inc., Mylan, NTech, Nanobiosym, Nanobiotix, Newbridge Ventures LLC, Noveome Biotherapeutics, Inc., Novo Nordisk, Particles for Humanity, Pfizer, Inc., Pioneer Hi-Bred International, Inc., Placon Therapeutics, Polaris Partners, Portal Instruments, Preceres, Llc (Acquired by Monsanto), PrognomIQ Inc., Pulmatrix, PureTech, Quris, ReLive, Reprocell USA, Inc. (Formerly Stemgent), Rubius Therapeutics, Secant Medical, Inc., Seer, Inc., Selecta Biosciences, Senses LLC, Setsuro Tech Inc., Seventh Sense Biosystems, Inc., Shenzhen Rice Life Technology, Ltd, Shire Ag, Sigilon, Sigma Aldrich Co. Llc, Sio, Ske S.R.L., Soil Culture Solutions LLC (Dba Soilcea), SQZ Biotechnologies, StemBioSys, Inc., SuonoBio, T Biosystems, Taconic Biosciences, Inc. (formerly Taconic Farms), TARA, Tarveda Therapeutics, Teal Bio, Tesio Pharmaceuticals, Third Rock Ventures, Tiba Biotech LLC, TissiuM (formerly ”Gecko”), Transgenic Inc., Translate Bio (Formerly Rana Therapeutics, Inc.), Trilink Biotechnologies, Inc., Unilever (Living Proof), VasoRX, Verseau Therapeutics, Inc., Vitakey, Vivtex Corporation, Whitehead Institute, Wiki Foods, Yz Biosciences (Guangzhou) Inc., Zenomics.
PAL He has also served as a consultant or advisor for Acorda Therapeutics, Amneal, Appello, Aptynix, Axovant Biosciences, Biogen, Cavion, Denali Therapeutics, F. Hoffmann-La Roche, Impel Neuropharma, Intec, Ipsen, Kyowa Kirin Hakko, Kashiv, Lundbeck A/S, Merck, Merz, Mitsubishi Tanabe Neuropharma, Neurocrine Biosciences, NeuroDerm Ltd, Noven, Parkinson Study Group, Prexton Therapeutics, Revance Therapeutics, Saccadous, Supernus, and US WorldMeds, and has received speaker honoraria from Acorda Therapeutics, Amneal, Britannia, the American Academy of Neurology, The International Parkinson’s Disease and Movement Disorders Society, Kyowa Kirin Hakko, Neurocrine Biosciences, Palidan Labs, Supernus, US WorldMeds, and the World Parkinson Congress. He is compensated for services as editor in chief of Clinical Neuropharmacology and serves without compensation on the editorial boards of Journal of Neural Transmission, Translational Neurodegeneration, and Journal of Parkinson’s Disease. His employers have received clinical research grant support from Acorda Therapeutics, Amneal, Aptinyx, Biotie, Bukwang, Cereval, Denali Therapeutics, Impax Labs, The Michael J. Fox Foundation for Parkinson’s Research, Pharma 2B, F. Hoffmann-La Roche, Revance, Scion NeuroStim LLC, Sunovion, Sun Pharma, and Supernus.
The authors have no other relevant affiliations or financial involvement with any organization or entity with a financial interest in or financial conflict with the subject matter or materials discussed in the manuscript apart from those disclosed.
Acknowledgments
Editorial assistance was provided by Robin Smith, PhD, of The Curry Rockefeller Group, LLC, and this assistance was funded by Acorda Therapeutics, Inc.
Additional information
Funding
References
- LeWitt PA. Levodopa for the treatment of Parkinson’s disease. N Engl J Med. 2008 Dec 4;359(23):2468–2476.
- LeWitt PA. Levodopa therapy for Parkinson’s disease: pharmacokinetics and pharmacodynamics. Mov Disord. 2015 Jan;30(1):64–72.
- Fasano A, Visanji NP, Liu LW, et al. Gastrointestinal dysfunction in Parkinson’s disease. Lancet Neurol. 2015 Jun;14(6):625–639.
- Pfeiffer RF, Isaacson SH, Pahwa R. Clinical implications of gastric complications on levodopa treatment in Parkinson’s disease. Parkinsonism Relat Disord. 2020 Jul;11(76):63–71.
- Heetun ZS, Quigley EM. Gastroparesis and Parkinson’s disease: a systematic review. Parkinsonism Relat Disord. 2012 Jun;18(5):433–440.
- Levein NG, Thorn SE, Wattwil M. Dopamine delays gastric emptying and prolongs orocaecal transit time in volunteers. Eur J Anaesthesiol. 1999 Apr;16(4):246–250.
- Robertson DR, Renwick AG, Wood ND, et al. The influence of levodopa on gastric emptying in man. Br J Clin Pharmacol. 1990 Jan;29(1):47–53.
- Nyholm D, Lennernas H. Irregular gastrointestinal drug absorption in Parkinson’s disease. Expert Opin Drug Metab Toxicol. 2008 Feb;4(2):193–203.
- McGee DJ, Lu XH, Disbrow EA. Stomaching the possibility of a pathogenic role for Helicobacter pylori in Parkinson’s disease. J Parkinsons Dis. 2018;8(3):367–374.
- Narozanska E, Bialecka M, Adamiak-Giera U, et al. Pharmacokinetics of levodopa in patients with Parkinson disease and motor fluctuations depending on the presence of Helicobacter pylori infection. Clin Neuropharmacol. 2014 Jul-Aug;37(4):96–99.
- Virmani T, Tazan S, Mazzoni P, et al. Motor fluctuations due to interaction between dietary protein and levodopa in Parkinson’s disease. J Clin Mov Disord. 2016;3:8.
- LeWitt PA, Hauser RA, Grosset DG, et al. A randomized trial of inhaled levodopa (CVT-301) for motor fluctuations in Parkinson’s disease. Mov Disord. 2016 Sep;31(9):1356–1365.
- LeWitt PA, Hauser RA, Pahwa R, et al. Safety and efficacy of CVT-301 (levodopa inhalation powder) on motor function during off periods in patients with Parkinson’s disease: a randomised, double-blind, placebo-controlled phase 3 trial. Lancet Neurol. 2019 Feb;18(2):145–154.
- Newman SP. Drug delivery to the lungs: challenges and opportunities. Ther Deliv. 2017 Jul;8(8):647–661.
- Roche N, Dekhuijzen PN. The evolution of pressurized metered-dose inhalers from early to modern devices. J Aerosol Med Pulm Drug Deliv. 2016 Aug;29(4):311–327.
- Stein SW, Thiel CG. The history of therapeutic aerosols: a chronological review. J Aerosol Med Pulm Drug Deliv. 2017 Feb;30(1):20–41.
- The Montreal protocol on substances that deplete the ozone layer, Final Act (Nairobi: UNEP 1987). Federal Register. 1994 (59 FR 56276–56298).
- De Boer AH, Hagedoorn P, Hoppentocht M, et al. Dry powder inhalation: past, present and future. Expert Opin Drug Deliv. 2017 Apr;14(4):499–512.
- Lavorini F, Pistolesi M, Usmani OS. Recent advances in capsule-based dry powder inhaler technology. Multidiscip Respir Med. 2017;12:11.
- Lin YW, Wong J, Qu L, et al. Powder production and particle engineering for dry powder inhaler formulations. Curr Pharm Des. 2015;21(27):3902–3916.
- Sibum I, Hagedoorn P, De Boer AH, et al. Challenges for pulmonary delivery of high powder doses. Int J Pharm. 2018 Sep 5;548(1):325–336.
- Telko MJ, Hickey AJ. Dry powder inhaler formulation. Respir Care. 2005 Sep;50(9):1209–1227.
- Edwards DA, Hanes J, Caponetti G, et al. Large porous particles for pulmonary drug delivery. Science. 1997 Jun 20;276(5320):1868–1871.
- Aitken ML, Bellon G, De Boeck K, et al. Long-term inhaled dry powder mannitol in cystic fibrosis: an international randomized study. Am J Respir Crit Care Med. 2012 Mar 15;185(6):645–652.
- Bilton D, Robinson P, Cooper P, et al. Inhaled dry powder mannitol in cystic fibrosis: an efficacy and safety study. Eur Respir J. 2011 Nov;38(5):1071–1080.
- Tappenden P, Harnan S, Uttley L, et al. Colistimethate sodium powder and tobramycin powder for inhalation for the treatment of chronic Pseudomonas aeruginosa lung infection in cystic fibrosis: systematic review and economic model. Health Technol Assess. 2013 Dec;17(56):v–xvii, 1–181.
- Uttley L, Harnan S, Cantrell A, et al. Systematic review of the dry powder inhalers colistimethate sodium and tobramycin in cystic fibrosis. Eur Respir Rev. 2013 Dec;22(130):476–486.
- Geller DE, Weers J, Heuerding S. Development of an inhaled dry-powder formulation of tobramycin using PulmoSphere technology. J Aerosol Med Pulm Drug Deliv. 2011 Aug;24(4):175–182.
- Hickey AJ. Back to the future: inhaled drug products. J Pharm Sci. 2013 Apr;102(4):1165–1172.
- Healy AM, Amaro MI, Paluch KJ, et al. Dry powders for oral inhalation free of lactose carrier particles. Adv Drug Deliv Rev. 2014 Aug;75:32–52.
- Bartus RT, Emerich D, Snodgrass-Belt P, et al. A pulmonary formulation of L-dopa enhances its effectiveness in a rat model of Parkinson’s disease. J Pharmacol Exp Ther. 2004 Aug;310(2):828–835.
- Lipp MM, Batycky R, Moore J, et al. Preclinical and clinical assessment of inhaled levodopa for OFF episodes in Parkinson’s disease. Sci Transl Med. 2016 Oct 12;8(360):360ra136.
- Notter RH, Finkelstein JN. Pulmonary surfactant: an interdisciplinary approach. J Appl Physiol Respir Environ Exerc Physiol. 1984 Dec;57(6):1613–1624.
- Willson DF, Notter RH. The future of exogenous surfactant therapy. Respir Care. 2011 Sep;56(9):1369–1386. discussion1386–1388.
- Goetz CG, Tilley BC, Shaftman SR, et al. Movement Disorder Society-sponsored revision of the Unified Parkinson’s Disease Rating Scale (MDS-UPDRS): scale presentation and clinimetric testing results. Mov Disord. 2008 Nov 15;23(15):2129–2170.
- Safirstein BE, Ellenbogen A, Zhao P, et al. Pharmacokinetics of inhaled levodopa administered with oral carbidopa in the fed state in patients with Parkinson’s disease. Clin Ther. 2020 May 29;42(6):1034–1046.
- Grosset DG, Dhall R, Gurevich T, et al. Inhaled levodopa in Parkinson’s disease patients with OFF periods: a randomized 12-month pulmonary safety study. Parkinsonism Relat Disord. 2019 Dec 23;71:4–10.
- Farbman ES, Waters CH, LeWitt PA, et al. A 12-month, dose-level blinded safety and efficacy study of levodopa inhalation powder (CVT-301, Inbrija) in patients with Parkinson’s disease. Parkinsonism Relat Disord. 2020 Oct 17;81:144–150.
- Hauser RA, Isaacson SH, Ellenbogen A, et al. Orally inhaled levodopa (CVT-301) for early morning OFF periods in Parkinson’s disease. Parkinsonism Relat Disord. 2019 Jul;64:175–180.
- Ibrahim M, Verma R, Garcia-Contreras L. Inhalation drug delivery devices: technology update. Med Devices (Auckl). 2015;8:131–139.
- Martinez-Fernandez R, Schmitt E, Martinez-Martin P, et al. The hidden sister of motor fluctuations in Parkinson’s disease: a review on nonmotor fluctuations. Mov Disord. 2016 Aug;31(8):1080–1094.
- Olanow CW, Stocchi F. Levodopa: SF. A new look at an old friend. Mov Disord. 2018 Jul;33(6):859–866.
- Chou KL, Stacy M, Simuni T, et al. The spectrum of “off” in Parkinson’s disease: what have we learned over 40 years? Parkinsonism Relat Disord. 2018 Jun;51:9–16.
- LeWitt PA, Chaudhuri KR. Unmet needs in Parkinson disease: motor and non-motor. Parkinsonism Relat Disord. 2020 Nov;80(Suppl 1):S7–S12.
- Matthews H, Stamford J, Saha R, et al. Exploring issues around wearing-off and quality of life: the OFF-PARK survey of people with Parkinson’s disease and their care partners. J Parkinsons Dis. 2015;5(3):533–539.
- Chapuis S, Ouchchane L, Metz O, et al. Impact of the motor complications of Parkinson’s disease on the quality of life. Mov Disord. 2005 Feb;20(2):224–230.
- Stacy M, Bowron A, Guttman M, et al. Identification of motor and nonmotor wearing-off in Parkinson’s disease: comparison of a patient questionnaire versus a clinician assessment. Mov Disord. 2005 Jun;20(6):726–733.
- Connolly BS, Lang AE. Pharmacological treatment of Parkinson disease: a review. JAMA. 2014 Apr 23-30;311(16):1670–1683.
- Moore H, Shpiner DS, Luca CC. Management of motor features in advanced Parkinson disease. Clin Geriatr Med. 2020 Feb;36(1):43–52.
- Moldovan AS, Groiss SJ, Elben S, et al. The treatment of Parkinson’s disease with deep brain stimulation: current issues. Neural Regen Res. 2015 Jul;10(7):1018–1022.
- Bronstein JM, Tagliati M, Alterman RL, et al. Deep brain stimulation for Parkinson disease: an expert consensus and review of key issues. Arch Neurol. 2011 Feb;68(2):165.
- Pfeiffer RF. Gastrointestinal dysfunction in Parkinson’s disease. Curr Treat Options Neurol. 2018 Oct 25;20(12):54.
- Nutt JG, Woodward WR, Hammerstad JP, et al. The “on-off” phenomenon in Parkinson’s disease. Relation to levodopa absorption and transport. N Engl J Med. 1984 Feb 23;310(8):483–488.
- Isaacson S, Lew M, Ondo W, et al. Apomorphine subcutaneous injection for the management of morning akinesia in Parkinson’s disease. Mov Disord Clin Pract. 2017 Jan-Feb;4(1):78–83.
- Hauser RA, Olanow CW, Dzyngel B, et al. Sublingual apomorphine (APL-130277) for the acute conversion of OFF to ON in Parkinson’s disease. Mov Disord. 2016 Sep;31(9):1366–1372.