ABSTRACT
Introduction
On-target, off-tumor toxicity severely limits systemic dosing of cytokines and agonist antibodies for cancer. Intratumoral administration is increasingly being explored to mitigate this problem. Full exploitation of this mode of administration must include a mechanism for sustained retention of the drug; otherwise, rapid diffusion out of the tumor eliminates any advantage.
Areas covered
We focus here on strategies for anchoring immune agonists in accessible formats. Such anchoring may utilize extracellular matrix components, cell surface receptor targets, or exogenously administered particulate materials. Promising alternative strategies not reviewed here include slow release from the interior of a material depot, expression following local transfection, and conditional proteolytic activation of masked molecules.
Expert opinion
An effective mechanism for tissue retention is a critical component of intratumorally anchored cytokine therapy, as leakage leads to decreased tumor drug exposure and increased systemic toxicity. Matching variable drug release kinetics with receptor-mediated cellular uptake is an intrinsic requirement for the alternative strategies mentioned above. Bioavailability of an anchored form of the administered drug is key to obviating this balancing act.
1. Introduction
Despite the expanding success of immunotherapy for cancer, there remains an important gap in the therapeutic space: immune agonist antibodies [Citation1] and cytokines [Citation2,Citation3] have yet to make significant inroads, despite the early approval of IL-2 for melanoma over 30 years ago. This stands in stark contrast with the broad reach and acceptance of antagonistic drugs, such as checkpoint blockade antibodies. The crux of the issue with agonist drugs is on-target (correct receptor type) /off-tumor (wrong tissue localization) activity resulting in a narrow therapeutic window, as represented schematically in (). The high drug doses required to achieve therapeutic levels in tumor tissue inevitably activate immune cells throughout the body, producing severe systemic dose-limiting toxicities. To circumvent this challenge, strategies have been developed to: a) limit systemic activity through masking [Citation4–8]; b) use weakened mutant cytokines either alone or targeted within a bispecific construct (NCT04250155) [Citation9,Citation10]; c) alter cytokine receptor subunit specificities [Citation11,Citation12]; d) express cytokines intratumorally [Citation13–19]; or e) entrap cytokines within eroding biomaterial matrices [Citation20,Citation21]. In addition, anchoring cytokines to a pharmacologically insoluble scaffold that stably persists in a bioavailable state is a fundamentally distinct delivery strategy that we will focus on in this review. A particular benefit of this strategy is that it shifts both of the response curves in () in favorable directions, rather than just one of the two.
Figure 1. Framing the problem: two alternative ways to open the therapeutic window. Potency of cytokines is usually similar within and outside tumor tissue, leading to close tracking of the toxicity and efficacy dose response curves. The hypothetical curves shown here schematically represent this phenomenon. The resulting therapeutic window can then be impractically small – or even nonexistent. Multiple efforts are underway to solve this problem. Anchored cytokine immunotherapy shifts both response curves in favorable directions, greatly expanding the therapeutic window.
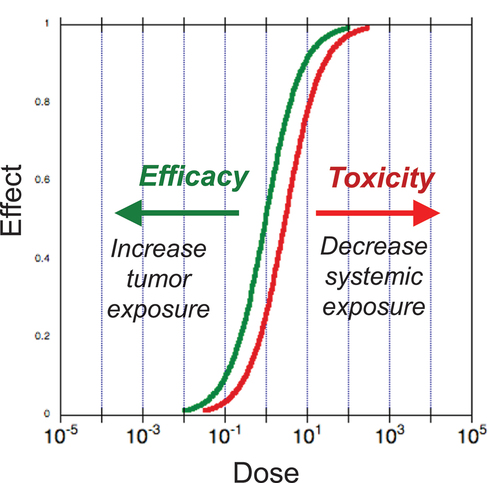
2. Intratumoral therapy
Enthusiasm for intratumoral drug administration is burgeoning, encouraged by the recognition that self-vaccinal stimulation of an adaptive anti-tumor T cell response holds potential for systemic and durable therapeutic effects [Citation22–28]. Direct injection allows generation of in situ tumor-associated antigens without prior antigen identification or synthesis. Approval of talimogene laherparepvec (TVEC) oncolytic viral therapy and ongoing clinical trials for multiple others [Citation14], together with favorable clinical results for intratumorally administered toll-like receptor (TLR) agonists [Citation29] provide encouraging precedents. Advances in the field of interventional radiology [Citation26] and robotic endoscopy [Citation30] have significantly ameliorated previous concerns regarding the accessibility of visceral tumors for injection. Nevertheless, an underappreciated issue to date has been the rapidity with which soluble cytokines or other protein drugs leak out of a tumor following injection, leading to reduced efficacy, a need for frequent repeat injections, and potentially toxic systemic accumulation of drug.
2.1. Intratumorally administered proteins exit rapidly in the absence of a retention strategy
The speed with which cytokine or antibody-size proteins diffuse out of a tumor is faster than often recognized. However, this phenomenon has been thoroughly demonstrated in mouse transplant tumor models [Citation31–34]. Furthermore, this same rapid exit is observed in the clinic. An early intratumoral IL-12 clinical trial resulted in peak plasma IL-12 5–7 hours post injection, and ‘remarkable toxicity … at the 100 ng/kg dose’ [Citation35]. Inducible IL-12 expression from intratumorally administered oncolytic viruses in glioblastoma multiforme (GBM) has resulted in active levels of IL-12 in the blood [Citation13]. These results demonstrate that if the rate of local cytokine provision does not closely approximate the local consumption rate, cytokine can be released at systemically toxic levels.
Enclosure of agonists within material depots such as hydrogels [Citation20] or chitosan [Citation21] prevents their rapid release, but also obscures the payload from intratumoral target immune cells until the payload is released from the depot. The release rate of soluble drug from the depot material must be matched to the client cell uptake rate lest toxic leakage occur. Similarly, approaches that rely on protein expression or proteolytic unmasking of soluble drug at the tumor site must generate sufficient drug to prime an effective response without exceeding local cell uptake and causing systemic leakage. Achieving optimal dosing is complicated by high heterogeneity in transfection efficiency or protease levels across primary tumors. As an alternative, we review here strategies for delivering bioavailable but physically anchored cytokines ().
Figure 2. Schematic of alternative approaches to directly inject cytokines into tumors. Administration of soluble or depot-contained cytokine produces a kinetic competition between target cell uptake (T cells in this representation), and leakage out of the tumor. Skewing that competition toward intratumoral target-mediated consumption and away from leakage is a moving target, as both the number of target cells and depot release rate may vary patient-to-patient, by tumor microenvironment composition, and over time. Administering cytokine in a sterically accessible but anchored format obviates this issue.
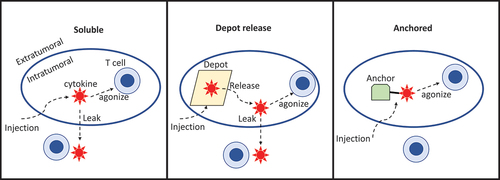
2.2. Alternative anchorage points
Three categories of anchored cytokine attachment points can be envisioned: extracellular matrix, cell surfaces, or exogenously administered materials. Each option offers differing spatial distribution, cytokine loading capacity, and retention kinetics, leading to numerous potential degrees of freedom for future optimization. Several examples of each type have been reported to date, and further optimization is accelerating. The most advantageous anchor type for any given payload remains to be discovered.
2.2.1. Extracellular matrix (ECM) anchoring
An early application of ECM anchoring exploited the specificity of expression of the fibronectin extra domain B (EDB) in the perivascular compartment of tumors and healing wounds [Citation36]. This specificity enables imaging from systemically administered anti-EDB antibodies [Citation37,Citation38]. EDB antibodies fused with cytokines (a type of ‘immunocytokine’) can enhance tumor localization and cytokine activity after systemic administration, but such agents also stimulate their cognate cytokine receptors on immune cells in the bloodstream [Citation39] thereby placing a limit on dose levels that can be achieved and the corresponding therapeutic window. Instead, administering these EDB-targeted immunocytokines intratumorally may provide optimal specificity and activity with extended tumor retention through ECM binding at the injection site [Citation40–42]. The alternatively spliced EDA domain of fibronectin has been used analogously to anchor cytokines such as IL-12 following intratumoral injection [Citation43].
Collagen is one of the most abundant proteins in the body, almost omnipresent in solid tumors [Citation44]. It therefore makes an appealing target for cytokine fusion proteins, which have been administered systemically [Citation45] or intratumorally [Citation46]. In favor of intratumoral administration, it is perhaps noteworthy that accessible collagen attachment sites in the liver and kidney can together capture a significant fraction of collagen-binding proteins if systemically administered, and hepatotoxicity is often dose-limiting for immune agonists – although this paper did not report significant immune related events in the treated mice [Citation45]. Intratumorally administered collagen-binding IL-2 and IL-12 fusion proteins cure established syngeneic tumors, and prime a sufficient CD8 T cell response to cure contralateral noninjected tumors [Citation46]. In a model with resection of mammary fat pad 4T1 tumors, neoadjuvant intratumoral administration of collagen-binding IL-12 essentially eliminates formation of pulmonary metastases, an indication of protective systemic adaptive immunity. Finally, a BRAF-PTEN genetically engineered mouse model of melanoma is cured by intratumoral collagen-retained IL-2 and IL-12 plus systemic checkpoint blockade [Citation46].
The extensive history of tumor targeting via systemic administration of antibodies and other binders motivated development of a predictive theory to provide design guidance with respect to tumor-targeting drug size and binding affinity as they determine the efficiency of tumor drug uptake [Citation47]. This ‘outside-in’ model describes principally the tradeoff between decreased renal clearance with increasing size, vs. decreased extravasation with increasing size. An analogous model was recently reported for ‘inside-out’ intratumoral drug delivery to an ECM anchorage point such as collagen [Citation48], and was validated with quantitative PET biodistribution imaging. Key predictions of this model are that for ECM-anchored agents, molecular weights above approximately 60 kDa are preferable to decrease the rate of diffusion out of the tumor, while improved matrix-binding affinity monotonically increases retention. Consistent with these model predictions, tighter collagen-binding IL-2 fusion proteins gave progressively more beneficial therapeutic effects in this system [Citation48].
2.2.2. Cell surfaces
Cell surface receptors are another point of attachment for intratumorally administered cytokines. The immunocytokine drug class was designed to accomplish tumor localization following systemic administration [Citation49]. However, uptake by circulating cytokine receptor-positive immune cells creates a significant sink for such drugs [Citation39,Citation50] – a sink which is then expanded in a positive feedback loop by amplification of the number of cytokine-receptor-expressing cells [Citation50,Citation51]. This amplification and activation of circulating client cells also generates dose-limiting toxicity, in addition to consuming a significant proportion of the drug dose. One way to circumvent this issue is to intratumorally administer immunocytokines originally intended for intravenous administration. The high local concentration of drug after intratumoral administration rapidly binds to target antigen in the tumor leading to prolonged retention and a potent immune response while avoiding systemic clearance and toxicity [Citation41,Citation52,Citation53]. Key design parameters include target cell and receptor numbers, as well as the endocytosis kinetics driving target-mediated drug disposition (TMDD). One might expect that endocytic consumption could limit target exposure duration, but nevertheless this approach has been efficacious in tumor models.
2.2.3. Exogenous depot
Rather than rely on endogenously expressed attachment points on the ECM or cell surfaces, one can attach cytokines to an insoluble or particulate material, in such a fashion as to not sterically occlude the cytokine. An early example of this approach was the intratumoral administration of liposomes with lipid-tethered cytokines on the surface [Citation32,Citation33] – however, here the longevity of stimulation is limited by rapid biodegradation/endocytic clearance of lipid vesicles. Interestingly, even systemically administered nanoparticles of this type can deliver cytokines to the tumor volume via the enhanced permeability and retention (EPR) effect, with residual circulating particles being rapidly cleared by the reticuloendothelial system, thereby diminishing systemic toxicity [Citation54]. Exosomes have also been engineered to surface-display IL-12 for intratumoral administration in a similar manner [Citation55] and have entered early stage clinical trials (NCT05156229).
It has been shown recently that the common vaccine adjuvant alum can serve as an attachment point for presentation of cytokines in the tumor space. Although physisorption to the material can be rapidly reversed in vivo, one can instead exploit a strong ligand exchange reaction between phosphorylated amino acids and the aluminum hydroxide matrix [Citation56]. When IL-12 is attached to alum in this fashion, it persists in active form at the injection site for at least one to two weeks, extensively activating the myeloid compartment, and stimulating priming of anti-tumor CD8 + T cells [Citation57]. Single intratumoral administrations of alum-anchored IL-12, alone or in combination with systemic PD-1 antibody blockade, cure established syngeneic tumors in mice with negligible toxicity.
2.3. Priming systemic adaptive immunity
A common concern raised for intratumoral therapy is how to treat disseminated, metastatic disease. This is because the classic paradigm in oncologic pharmacology has been to design drugs intended to kill every cancer cell in the patient, hoping to eliminate resistance and recurrence. With the advent of immunotherapy, drugs are instead targeted to immune cells to foster potentially curative adaptive immune memory in the form of CD8 + T cells, whether exogenously administered or primed endogenously by therapy. The adaptive immune response can proceed to track tumor antigenic adaptations in a fashion that static therapies do not.
T cell responses that protect against tumor re-challenge are more the norm than the exception for curative immunotherapies in mice [Citation15,Citation33,Citation39,Citation41,Citation46,Citation48,Citation57–63]. Of greater interest is whether such responses happen in treated humans. The frequency of occurrence of spontaneous protective immunity (an ‘abscopal’ effect) from external beam radiation therapy alone has been vanishingly small [Citation64], perhaps unsurprisingly so given collateral damage to infiltrating immune cells and the tumor draining lymph nodes essential for an immune response. Promisingly, there are a growing number of clinical reports of responses in uninjected lesions following intratumoral immunotherapy. The extensive (but not exhaustive) list of examples of clinical abscopal effects from intratumoral therapies summarized below strongly supports the contention that this form of therapy can prime tumor-specific and clinically meaningful systemic T cell responses. Intratumoral administration of ECM-anchored IL-2 and TNF-alpha led to complete responses in over half of the noninjected lesions in 20 melanoma patients [Citation40]. A clinical trial of intratumoral Flt3L and TLR agonist, plus external beam radiation therapy, recruited cross-presenting DCs and induced an anti-tumor CD8 + T cell response leading to systemic cancer remission in patients with advanced indolent Non-Hodgkin’s Lymphoma [Citation27]. Local radiotherapy and anti-CTLA-4 antibody treatment together induced systemic anti-tumor T cells in metastatic NSCLC patients, achieving an 18% response rate [Citation65]. In a trial of intratumoral oncolytic virus combined with systemic anti-CTLA-4, 52% of the patients showed decreases in non-virally-injected visceral lesions (by comparison to 23% for those treated with anti-CTLA4 as monotherapy) [Citation66]. Phase I studies of intratumoral CpG combined with local radiation therapy elicited partial responses in uninjected lesions of both lymphoma [Citation67] and mycosis fungoides [Citation68] patients. Intratumoral electroporation of an IL-12 expression plasmid led to regression of at least one uninjected lesion in 46% of treated metastatic melanoma patients [Citation69]. In a trial of neoadjuvant checkpoint blockade plus primary NSCLC lesion stereotactic irradiation, 66% of patients downgraded previously tumor-positive tumor-draining lymph nodes to ‘N0’ stage following therapy [Citation70].
This growing list of successful clinical abscopal effects from intratumoral immunotherapy provides solid evidence that it is possible in some patients to foster an efficacious adaptive immune response. Such ‘existence proofs’ are perhaps analogous to the dawn of immunotherapy, when the first robust responses obtained with anti-PD-1 therapy were observed in only a minority of patients, yet were indicative that, with further engineering optimization, such successes could be expanded to a broader patient population.
2.4. Technical advancements in intralesional therapy
A common question is: how readily can intralesional therapy be applied to nonsuperficial lesions? In fact, there have been numerous advancements in the fields of image-guided interventional radiology [Citation25,Citation26] and robotic endoscopy [Citation30] that make most lesions injectable. Further demonstration of this feasibility is the growing prevalence of needle biopsies of tumors. In a survey of Medicare patients, over 700,000 needle biopsies were performed in 2010 [Citation71]. Many such biopsies are performed annually in breast [Citation72], lung [Citation73], prostate [Citation74], and liver [Citation75] cancer. Even so, such procedures are sufficiently invasive that it is desirable to limit their repeat frequency. To quote Hong & Levy [Citation76]: ‘due to increased availability of interventional radiologic, endoscopic and laparoscopic procedures, most if not all lesions can now be accessed with or without the assistance of imaging modalities such as ultrasound, CT, etc … Therefore, the challenge in intratumoral therapy now lies not in initial accessibility, but in optimization of drug delivery technologies to enhance intratumoral delivery and reduce repeat injections.’ It is realistic to expect that safe and effective intratumoral immunotherapies that do not require frequent administration will find a straightforward path to acceptance in clinical practice.
3. Conclusions
To date, the areas of greatest growth in cancer immunotherapy have been antagonistic antibodies that target T cells, and autologous engineered T cell therapies. There is a glaring absence of agonistic therapies in the immune oncology pharmacopoeia, despite the clear potential advantages that could be achieved by activating anti-tumor T cells or the ancillary immune cells that instruct and support adaptive cell-mediated immunity. Most clinical trials of immune agonists fail due to dose-limiting toxicity prior to attainment of immune activation in the tumor compartment. A wide variety of tactics are being deployed to circumvent this limitation, but in this review we have focused on the rather straightforward notion of simply putting the drug in the tumor and making it stay there. This strategy has demonstrated compelling efficacy and tolerability in preclinical tumor models.
4. Expert opinion
Anchored cytokines are a key tool for developing biomimetic therapeutic strategies. Biomimicry is a powerful design principle for molecular bioengineers because natural selection hones solutions that are, by definition, robust and effective. Aligning one’s technical objectives with nature’s survival imperative has historically led to major advances (taking as just one example the success of directed evolution in crafting new enzymes and antibodies.) Along these lines, pharmacology might benefit by borrowing from the playbook of an immune response to an invading pathogen. A series of actions limited in location, duration, and sequence reject the initial infection, and construct memory of the attacker’s molecular features that leads to rapid future protection against the same pathogen. One could say that the natural immune response is therefore ‘spatiotemporally programmed.’ Why should we not do the same for cancer immunotherapy?
Taking IL-2 as an example, it is transiently expressed primarily (though not exclusively) by CD4 + T cells following T cell receptor (TCR) stimulation [Citation77]. IL-2 expression is negatively regulated by autocrine/paracrine feedback control loops [Citation78] that strictly limit the duration and location of its expression. By contrast with this endogenous local and time-limited deployment of IL-2, high-dose IL-2 therapy utilizes extended bolus infusions that more closely resembles the exposure patterns of an endocrine factor. This distinction might well be expected to contribute to immune suppressive effects detrimental to therapy, particularly from regulatory T cells stimulated via IL-2 exposure [Citation79].
There is a growing recognition that temporal sequencing (i.e. dose scheduling) within treatment cocktails can have definitive effects on the success or failure of therapy [Citation80]. For example, type I interferon treatment prior to significant antigen uptake completely short-circuits a tumor immune response, while delaying the very same dosage relative to delivery of an anti-tumor antibody produces a curative therapy [Citation61].
Meanwhile, efforts to spatially restrict immunotherapy drugs have been impeded by the widespread preference to ‘soak’ the patient systemically with drug at its maximum tolerated dose for as long as tolerable. The misguidedness of this approach can perhaps best be appreciated by recognizing that a vaccinal effect is essentially an absolute requirement for curative immunotherapy. Would one optimize a vaccine by maximizing antigen and adjuvant exposure throughout the body for extended periods of time? Clearly not. Rather, intense localized inflammation together with concentrated depots of antigen are found to contribute to the most successful vaccination strategies. The analogy to a ‘hot’ tumor microenvironment with high tumor antigen density is direct – the objective should be for the tumor itself to become a localized vaccination site. Such an approach could circumvent the on-going immunoediting process that limits many immunotherapies [Citation81].
The optimal combination of intratumoral location, timing, and duration of exposure is likely to vary considerably amongst different cytokine drug candidates. Although first-generation anchored cytokine immunotherapies have demonstrated powerful preclinical efficacy with minimal toxicity [Citation32,Citation33,Citation46,Citation48,Citation57], it is to be expected that still further improvements will be attainable through the development of better tools for controlling the spatiotemporal cytokine exposure profile, and identification of optimal combinations with mainstay systemic therapies such as checkpoint blockade. Providing oncologists with such tools to precisely orchestrate immune attacks on solid tumors could significantly advance the clinical management of cancer.
Article highlights
Intratumoral administration is increasingly being explored to overcome poor therapeutic indices with immune agonist drugs for cancer.
In the absence of a retention mechanism, localization of protein drugs is rapidly lost.
Anchored, bioavailable cytokines solve the problem of matching local drug supply with cellular demand.
Agonists have been bioavailability anchored to extracellular matrix, biomaterials, or cell surfaces.
Systemic adaptive immune responses to intratumoral agonists have been observed preclinically and in humans.
Tumor anchored cytokines are a critical tool for rational spatiotemporally programmed immunotherapy.
Declaration of interest
KD Wittrup & DJ Irvine are inventors on patent applications filed by the Massachusetts Institute of Technology in the areas described in this review. HL Kaufman and MM Schmidt are employees of Ankyra Therapeutics, which has licensed MIT IP on alum-anchored cytokines, and is clinically translating this technology. KD Wittrup, DJ Irvine, HL Kaufman, and MM Schmidt hold equity in Ankyra Therapeutics. DJ Irvine is an Investigator of the Howard Hughes Medical Institutes. The authors have no other relevant affiliations or financial involvement with any organization or entity with a financial interest in or financial conflict with the subject matter or materials discussed in the manuscript apart from those disclosed.
Reviewer disclosures
Peer reviewers on this manuscript have no relevant financial or other relationships to disclose.
Additional information
Funding
References
- Garber K. Immune agonist antibodies face critical test. Nat Rev Drug Discov. 2020;19(1):3–5. PubMed PMID: 31907434.
- Holder PG, Lim SA, Huang CS, et al. Engineering interferons and interleukins for cancer immunotherapy. Adv Drug Deliv Rev. 2022;182:114112. Epub 20220124. PubMed PMID: 35085624.
- Mansurov A, Lauterbach A, Budina E, et al.Immunoengineering approaches for cytokine therapy.Am J Physiol Cell Physiol.2021;321(2):C369–C83; Epub 20210707. PubMed PMID: 34232748;
- Nirschl CJ, Brodkin HR, Hicklin DJ, et al. Discovery of a conditionally activated interleukin-2 that promotes anti-tumor immunity and induces tumor regression. Cancer Immunol Res. 2022;10:581–596. Epub 20220314. PubMed PMID: 35286392.
- Hsu EJ, Cao X, Moon B, et al. A cytokine receptor-masked IL2 prodrug selectively activates tumor-infiltrating lymphocytes for potent antitumor therapy. Nat Commun. 2021;12(1):2768. Epub 20210513. PubMed PMID: 33986267; PubMed Central PMCID: PMC8119481
- Erster O, Thomas JM, Hamzah J, et al. Site-specific targeting of antibody activity in vivo mediated by disease-associated proteases. J Control Release. 2012;161(3):804–812. : Epub 20120523. PubMed PMID: 22634092; PubMed Central PMCID: PMC3412904
- Adams G, Vessillier S, Dreja H, et al.Targeting cytokines to inflammation sites.Nat Biotechnol.2003;21(11):1314–1320; Epub 20031005. PubMed PMID: 14528315;
- Mansurov A, Hosseinchi P, Chang K, et al. Masking the immunotoxicity of interleukin-12 by fusing it with a domain of its receptor via a tumour-protease-cleavable linker. Nat Biomed Eng. 2022. Epub 20220509. https://doi.org/10.1038/s41551-022-00888-0. PubMed PMID: 35534574.
- Xu Y, Carrascosa LC, Yeung YA, et al. An engineered IL15 cytokine mutein fused to an Anti-PD1 improves intratumoral T-cell function and antitumor immunity. Cancer Immunol Res. 2021;9(10):16–25. Epub 20210810. PubMed PMID: 34376502
- Pogue SL, Taura T, Bi M, et al. Targeting attenuated interferon-α to myeloma cells with a CD38 antibody induces potent tumor regression with reduced off-target activity. PLoS One. 2016;11(9):e0162472. Epub 20160909. PubMed PMID: 27611189; PubMed Central PMCID: PMC5017640
- Spangler JB, Moraga I, Mendoza JL, et al. Insights into cytokine-receptor interactions from cytokine engineering. Annu Rev Immunol. 2015;33:139–167. Epub 20141210. PubMed PMID: 25493332; PubMed Central PMCID: PMC4445396.
- Glassman CR, Mathiharan YK, Jude KM, et al. Structural basis for IL-12 and IL-23 receptor sharing reveals a gateway for shaping actions on T versus NK cells. Cell. 2021;184(4):983–99.e24. PubMed PMID: 33606986; PubMed Central PMCID: PMC7899134.
- Chiocca EA, Yu JS, Lukas RV, et al. Regulatable interleukin-12 gene therapy in patients with recurrent high-grade glioma: results of a phase 1 trial. Sci Transl Med. 2019;11(505). PubMed PMID: 31413142; PubMed Central PMCID: PMC7286430. https://doi.org/10.1126/scitranslmed.aaw5680.
- Kaufman HL, Shalhout SZ, Iodice G. Talimogene Laherparepvec: moving From First-In-Class to Best-In-Class. Front Mol Biosci. 2022;9:834841. Epub 20220222. PubMed PMID: 35274007; PubMed Central PMCID: PMC8901478.
- Li Y, Su Z, Zhao W, et al. Multifunctional oncolytic nanoparticles deliver self-replicating IL-12 RNA to eliminate established tumors and prime systemic immunity. Nat Cancer. 2020;1(9):882–893. Epub 20200810. PubMed PMID: 34447945; PubMed Central PMCID: PMC8386348
- Hewitt SL, Bailey D, Zielinski J, et al. Intratumoral IL12 mRNA therapy promotes TH1 transformation of the tumor microenvironment. Clin Cancer Res. 2020;26(23):6284–6298. Epub 20200817. PubMed PMID: 32817076
- Hotz C, Wagenaar TR, Gieseke F, et al. Local delivery of mRNA-encoded cytokines promotes antitumor immunity and tumor eradication across multiple preclinical tumor models. Sci Transl Med. 2021;13(610):eabc7804. Epub 20210908. PubMed PMID: 34516826
- Nash AM, Jarvis MI, Aghlara-Fotovat S, et al. Clinically translatable cytokine delivery platform for eradication of intraperitoneal tumors. Sci Adv. 2022;8(9):eabm1032. Epub 20220302. PubMed PMID: 35235346; PubMed Central PMCID: PMC8890714
- Daud AI, DeConti RC, Andrews S, et al. Phase I trial of interleukin-12 plasmid electroporation in patients with metastatic melanoma. J Clin Oncol. 2008;26(36):5896–5903. Epub 20081124. PubMed PMID: 19029422; PubMed Central PMCID: PMC2645111
- Park CG, Hartl CA, Schmid D, et al. Extended release of perioperative immunotherapy prevents tumor recurrence and eliminates metastases. Sci Transl Med. 2018;10(433): PubMed PMID: 29563317. https://doi.org/10.1126/scitranslmed.aar1916.
- Zaharoff DA, Hance KW, Rogers CJ, et al. Intratumoral immunotherapy of established solid tumors with chitosan/IL-12. J Immunother. 2010;33(7):697–705. PubMed PMID: 20664357; PubMed Central PMCID: PMC3468951.
- Marabelle A, Kohrt H, Caux C, et al. Intratumoral immunization: a new paradigm for cancer therapy. Clin Cancer Res. 2014;20(7):1747–1756. PubMed PMID: 24691639; PubMed Central PMCID: PMC3979477.
- Huang A, Pressnall MM, Lu R, et al. Human intratumoral therapy: linking drug properties and tumor transport of drugs in clinical trials. J Control Release. 2020;326:203–221. Epub 20200714. PubMed PMID: 32673633.
- Marabelle A, Andtbacka R, Harrington K, et al. Starting the fight in the tumor: expert recommendations for the development of human intratumoral immunotherapy (HIT-IT). Ann Oncol. 2018;29(11):2163–2174. PubMed PMID: 30295695; PubMed Central PMCID: PMC6290929.
- Tselikas L, Champiat S, Sheth RA, et al. Interventional radiology for local immunotherapy in oncology. Clin Cancer Res. 2021;27(10):2698–2705. Epub 20210108. PubMed PMID: 33419781
- Muñoz NM, Williams M, Dixon K, et al. Influence of injection technique, drug formulation and tumor microenvironment on intratumoral immunotherapy delivery and efficacy. J Immunother Cancer. 2021;9(2):e001800. PubMed PMID: 33589526; PubMed Central PMCID: PMC7887346.
- Hammerich L, Marron TU, Upadhyay R, et al. Systemic clinical tumor regressions and potentiation of PD1 blockade with in situ vaccination. Nat Med. 2019;25(5):814–824. Epub 20190408. PubMed PMID: 30962585
- Weide B, Neri D, Elia G. Intralesional treatment of metastatic melanoma: a review of therapeutic options. Cancer Immunol Immunother.2017;66(5):647–656; Epub 20170111. PubMed PMID: 28078357; PubMed Central PMCID: PMC6126622;
- Milhem M, Zakharia Y, Davar D, et al. Durable responses in anti-PD-1 refractory melanoma following intratumoral injection of Toll-like receptor 9 (TLR9) agonist CMP-001, in combination with pembrolizumab. J Immunother Cancer. 2020. https://doi.org/10.1136/LBA2019.4.
- Kent AJ, Byrnes KA, Chang SH. State of the art: robotic bronchoscopy. Semin Thorac Cardiovasc Surg.2020;32(4):1030–1035; Epub 20200823. PubMed PMID: 32846232;
- Rohner NA, Thomas SN. Melanoma growth effects on molecular clearance from tumors and biodistribution into systemic tissues versus draining lymph nodes. J Control Release. 2016;223:99–108. Epub 20151223. PubMed PMID: 26721446; PubMed Central PMCID: PMC4724472.
- Kwong B, Liu H, Irvine DJ. Induction of potent anti-tumor responses while eliminating systemic side effects via liposome-anchored combinatorial immunotherapy. Biomaterials.2011;32(22):5134–5147; Epub 20110422. PubMed PMID: 21514665; PubMed Central PMCID: PMC3140866;
- Kwong B, Gai SA, Elkhader J, et al.Localized immunotherapy via liposome-anchored Anti-CD137 + IL-2 prevents lethal toxicity and elicits local and systemic antitumor immunity.Cancer Res.2013;73(5):1547–1558; Epub 20130222. PubMed PMID: 23436794; PubMed Central PMCID: PMC3594475;
- Hanes J, Sills A, Zhao Z, et al. Controlled local delivery of interleukin-2 by biodegradable polymers protects animals from experimental brain tumors and liver tumors. Pharm Res. 2001;18(7):899–906. PubMed PMID: 11496947.
- van Herpen CM, Looman M, Zonneveld M, et al. Intratumoral administration of recombinant human interleukin 12 in head and neck squamous cell carcinoma patients elicits a T-helper 1 profile in the locoregional lymph nodes. Clin Cancer Res. 2004;10(8):2626–2635. PubMed PMID: 15102664.
- Neri D, Carnemolla B, Nissim A, et al. Targeting by affinity-matured recombinant antibody fragments of an angiogenesis associated fibronectin isoform. Nat Biotechnol. 1997;15(12):1271–1275. PubMed PMID: 9359110.
- Sauer S, Erba PA, Petrini M, et al. Expression of the oncofetal ED-B-containing fibronectin isoform in hematologic tumors enables ED-B-targeted 131I-L19SIP radioimmunotherapy in Hodgkin lymphoma patients. Blood. 2009;113(10):2265–2274. Epub 20090108. PubMed PMID: 19131554
- Jailkhani N, Ingram JR, Rashidian M, et al. Noninvasive imaging of tumor progression, metastasis, and fibrosis using a nanobody targeting the extracellular matrix. Proc Natl Acad Sci U S A. 2019;116(28):14181–14190. Epub 20190508. PubMed PMID: 31068469; PubMed Central PMCID: PMC6628802
- Tzeng A, Kwan BH, Opel CF, et al.Antigen specificity can be irrelevant to immunocytokine efficacy and biodistribution.Proc Natl Acad Sci U S A.2015;112(11):3320–3325; Epub 2015/03/02. PubMed PMID: 25733854; PubMed Central PMCID: PMC4371941;
- Danielli R, Patuzzo R, Di Giacomo AM, et al. Intralesional administration of L19-IL2/L19-TNF in stage III or stage IVM1a melanoma patients: results of a phase II study. Cancer Immunol Immunother. 2015;64(8):999–1009. Epub 20150514. PubMed PMID: 25971540
- Lutz E, Jailkhani N, Momin N, et al. Nanobody-IL-2 fusions targeting the tumor microenvironment suppress solid tumor growth in mice. Submitted. 2022.
- Pretto F, Elia G, Castioni N, et al.Preclinical evaluation of IL2-based immunocytokines supports their use in combination with dacarbazine, paclitaxel and TNF-based immunotherapy.Cancer Immunol Immunother.2014;63(9):901–910; Epub 20140604. PubMed PMID: 24893857;
- Pasche N, Wulhfard S, Pretto F, et al.The antibody-based delivery of interleukin-12 to the tumor neovasculature eradicates murine models of cancer in combination with paclitaxel.Clin Cancer Res.2012;18(15):4092–4103; Epub 20120612. PubMed PMID: 22693354;
- Riegler J, Labyed Y, Rosenzweig S, et al. Tumor elastography and its association with collagen and the tumor microenvironment. Clin Cancer Res. 2018;24(18):4455–4467. Epub 20180524. PubMed PMID: 29798909
- Ishihara J, Ishihara A, Sasaki K, et al. Targeted antibody and cytokine cancer immunotherapies through collagen affinity. Sci Transl Med. 2019;11(487). PubMed PMID: 30971453; PubMed Central PMCID: PMC6541444. https://doi.org/10.1126/scitranslmed.aau3259.
- Momin N, Mehta NK, Bennett NR, et al. Anchoring of intratumorally administered cytokines to collagen safely potentiates systemic cancer immunotherapy. Sci Transl Med. 2019;11(498). https://doi.org/10.1126/scitranslmed.aaw2614. PubMed PMID: 31243150; PubMed Central PMCID: PMC7811803
- Schmidt MM, Wittrup KD. A modeling analysis of the effects of molecular size and binding affinity on tumor targeting. Mol Cancer Ther. 2009;8(10):2861–2871. PubMed PMID: 19825804; PubMed Central PMCID: PMC4078872.
- Momin N, Palmeri JR, Lutz EA, et al. Maximizing response to intratumoral immunotherapy in mice by tuning local retention. Nat Commun. 2022;13(1):109. Epub 20220110. PubMed PMID: 35013154; PubMed Central PMCID: PMC8748612
- Hutmacher C, Neri D. Antibody-cytokine fusion proteins: biopharmaceuticals with immunomodulatory properties for cancer therapy. Adv Drug Deliv Rev. 2019;141:67–91. Epub 20180907. PubMed PMID: 30201522.
- Ribba B, Boetsch C, Nayak T, et al. Prediction of the optimal dosing regimen using a mathematical model of tumor uptake for immunocytokine-based cancer immunotherapy. Clin Cancer Res. 2018;24(14):3325–3333. Epub 20180220. PubMed PMID: 29463551
- van Brummelen EMJ, Huisman MC, de Wit-van der Veen LJ, et al. Zr-labeled CEA-targeted IL-2 variant immunocytokine in patients with solid tumors: CEA-mediated tumor accumulation and role of IL-2 receptor-binding. Oncotarget. 2018;9(37):24737–24749. Epub 20180515. PubMed PMID: 29872502; PubMed Central PMCID: PMC5973872
- Baniel CC, Sumiec EG, Hank JA, et al.Intratumoral injection reduces toxicity and antibody-mediated neutralization of immunocytokine in a mouse melanoma model. J Immunother Cancer. 2020;8(2):e001262. PubMed PMID: 33115944; PubMed Central PMCID: PMC7594540
- Hong Y, Robbins Y, Yang X, et al. Cure of syngeneic carcinomas with targeted IL-12 through obligate reprogramming of lymphoid and myeloid immunity. JCI Insight. Epub 20220308. https://doi.org/10.1172/jci.insight.157448. Epub 20220308. 2022; 7: 5
- Zhang Y, Li N, Suh H, et al.Nanoparticle anchoring targets immune agonists to tumors enabling anti-cancer immunity without systemic toxicity.Nat Commun.2018;9(1):6; Epub 20180102. PubMed PMID: 29295974; PubMed Central PMCID: PMC5750237;
- Lewis ND, Sia CL, Kirwin K, et al. Exosome surface display of il12 results in tumor-retained pharmacology with superior potency and limited systemic exposure compared with recombinant IL12. Mol Cancer Ther. 2021;20(3):523–534. Epub 20201221. PubMed PMID: 33443094
- Moyer TJ, Kato Y, Abraham W, et al. Engineered immunogen binding to alum adjuvant enhances humoral immunity. Nat Med. 2020;26(3):430–440. Epub 20200217. PubMed PMID: 32066977; PubMed Central PMCID: PMC7069805
- Agarwal Y, Milling LE, Chang JYH, et al. Intratumourally injected alum-tethered cytokines elicit potent and safer local and systemic anticancer immunity. Nat Biomed Eng. Epub 20220110. Epub 20220110. 2022;6:129–143.
- Kwan BH, Zhu EF, Tzeng A, et al. Integrin-targeted cancer immunotherapy elicits protective adaptive immune responses. J Exp Med. 2017;214(6):1679–1690. Epub 20170504. PubMed PMID: 28473400; PubMed Central PMCID: PMC5460993
- Moynihan KD, Opel CF, Szeto GL, et al. Eradication of large established tumors in mice by combination immunotherapy that engages innate and adaptive immune responses. Nat Med. 2016;22(12):1402–1410. Epub 20161024. PubMed PMID: 27775706; PubMed Central PMCID: PMC5209798
- Rothschilds A, Tzeng A, Mehta NK, et al.Order of administration of combination cytokine therapies can decouple toxicity from efficacy in syngeneic mouse tumor models.Oncoimmunology.2019;8(5):e1558678; Epub 2019/02/19. PubMed PMID: 31069130; PubMed Central PMCID: PMC6492973;
- Tzeng A, Kauke MJ, Zhu EF, et al. Temporally programmed CD8α. Cell Rep. 2016;17(10):2503–2511. PubMed PMID: 27926855; PubMed Central PMCID: PMC5204262.
- Wittrup KD. Antitumor antibodies can drive therapeutic T cell responses. Trends Cancer.2017;3(9):615–620; Epub 20170729. PubMed PMID: 28867165; PubMed Central PMCID: PMC5657573;
- Zhu EF, Gai SA, Opel CF, et al. Synergistic innate and adaptive immune response to combination immunotherapy with anti-tumor antigen antibodies and extended serum half-life IL-2. Cancer Cell. 2015;27(4):489–501. PubMed PMID: 25873172; PubMed Central PMCID: PMC4398916.
- Abuodeh Y, Venkat P, Kim S. Systematic review of case reports on the abscopal effect. Curr Probl Cancer.2016;40(1):25–37; Epub 20151009. PubMed PMID: 26582738;
- Formenti SC, Rudqvist NP, Golden E, et al. Radiotherapy induces responses of lung cancer to CTLA-4 blockade. Nat Med. 2018;24(12):1845–1851. Epub 20181105. PubMed PMID: 30397353; PubMed Central PMCID: PMC6286242
- Chesney J, Puzanov I, Collichio F, et al. Randomized, open-label phase ii study evaluating the efficacy and safety of talimogene laherparepvec in combination with ipilimumab versus ipilimumab alone in patients with advanced, unresectable melanoma. J Clin Oncol. 2018;36(17):1658–1667. Epub 20171005. PubMed PMID: 28981385; PubMed Central PMCID: PMC6075852
- Brody JD, Ai WZ, Czerwinski DK, et al. In situ vaccination with a TLR9 agonist induces systemic lymphoma regression: a phase I/II study. J Clin Oncol. 2010;28(28):4324–4332. Epub 20100809. PubMed PMID: 20697067; PubMed Central PMCID: PMC2954133
- Kim YH, Gratzinger D, Harrison C, et al. In situ vaccination against mycosis fungoides by intratumoral injection of a TLR9 agonist combined with radiation: a phase 1/2 study. Blood. 2012;119(2):355–363. Epub 20111101. PubMed PMID: 22045986; PubMed Central PMCID: PMC3257006
- Algazi A, Bhatia S, Agarwala S, et al. Intratumoral delivery of tavokinogene telseplasmid yields systemic immune responses in metastatic melanoma patients. Ann Oncol. 2020;31(4):532–540. Epub 20200201. PubMed PMID: 32147213
- Altorki NK, McGraw TE, Borczuk AC, et al. Neoadjuvant durvalumab with or without stereotactic body radiotherapy in patients with early-stage non-small-cell lung cancer: a single-centre, randomised phase 2 trial. Lancet Oncol. 2021;22(6):824–835. Epub 20210518. PubMed PMID: 34015311
- Kwan SW, Bhargavan M, Kerlan RK, et al.Effect of advanced imaging technology on how biopsies are done and who does them.Radiology.2010;256(3):751–758; Epub 20100629. PubMed PMID: 20587643; PubMed Central PMCID: PMC2923730;
- O’Flynn EA, Wilson AR, Michell MJ. Image-guided breast biopsy: state-of-the-art. Clin Radiol. 2010;65(4):259–270. PubMed PMID: 20338392.
- Tam AL, Kim ES, Lee JJ, et al. Feasibility of image-guided transthoracic core-needle biopsy in the BATTLE lung trial. J Thorac Oncol. 2013;8(4):436–442. PubMed PMID: 23442309; PubMed Central PMCID: PMC3879952.
- Moore CM, Robertson NL, Arsanious N, et al. Image-guided prostate biopsy using magnetic resonance imaging-derived targets: a systematic review. Eur Urol. 2013;63(1):125–140. Epub 20120613. PubMed PMID: 22743165
- Piccinino F, Sagnelli E, Pasquale G, et al. Complications following percutaneous liver biopsy. A multicentre retrospective study on 68,276 biopsies. J Hepatol. 1986;2(2):165–173. PubMed PMID: 3958472.
- Hong WX, Haebe S, Lee AS, et al. Intratumoral immunotherapy for early-stage solid tumors. Clin Cancer Res. 2020;26(13):3091–3099. Epub 2020/02/18. PubMed PMID: 32071116; PubMed Central PMCID: PMC7439755
- Malek TR. The biology of interleukin-2. Annu Rev Immunol. 2008;26:453–479. PubMed PMID: 18062768.
- Villarino AV, Tato CM, Stumhofer JS, et al. Helper T cell IL-2 production is limited by negative feedback and STAT-dependent cytokine signals. J Exp Med. 2007;204(1):65–71. Epub 20070116. PubMed PMID: 17227909; PubMed Central PMCID: PMC2118423
- Nelson BH. IL-2, regulatory T cells, and tolerance. J Immunol. 2004;172(7):125–140. PubMed PMID: 15034008.
- Rothschilds AM, Wittrup KD. What,Why, Where, and When: bringing timing to Immuno-Oncology. Trends Immunol.2019;40(1):12–21; Epub 20181210. PubMed PMID: 30545676;
- Mittal D, Gubin MM, Schreiber RD, et al. New insights into cancer immunoediting and its three component phases–elimination, equilibrium and escape. Curr Opin Immunol. 2014;27:16–25. Epub 20140214. PubMed PMID: 24531241; PubMed Central PMCID: PMC4388310.