ABSTRACT
Introduction
3D printing (3DP) applications in medicine are intensively investigated, creating an opportunity to provide patient-tailored therapy by delivering a drug with an accurate dose and release profile. Moving away from the ‘one size fits all’ paradigm, it could be beneficial for treating mental and neurological disorders, improving the efficiency and safety of the therapy. The aim of this critical review is to assess recent advances and identify gaps regarding 3DP in this important and challenging field, by focusing on recent research examples.
Areas covered
Applications of the 3DP techniques for solid dosage forms in mental and neurological disorders have been covered and discussed, together with recent advantages, limitations, and future directions.
Expert opinion
The personalize treatment, which is considered as the most significant advantage of the 3DP technique, can be beneficial in mental and neurological disorders therapy, where the dose should be adjusted to the patient. Printing of medicines enables creating the structure modifications and thus controlling the drug release or combining multiple drugs into one tablet, simplifying the dose regimen. Medications printed on-demand, in health-care facilities, could address the special needs of pediatric patients and help avoid interruptions in the supply chain. Despite promising advances, the described methods have limitations and need further investigation before being scaled-up to an industrial manufacturing environment. There is also a need to establish protocols for the preparation and registration of 3DP dosage forms.
1. Current treatment in psychiatric and neurological disorders
According to the World Health Organization (WHO) Mental Health Report 2022, 970 million people in 2019 were suffering from mental problems [Citation1], with the most common being anxiety and depression. In the same report, schizophrenia and bipolar disorders were considered the most substantial concern of mental health services [Citation1]. By affecting the working-age population, these diseases also substantially impact the economy.
The pharmacotherapy of mental disorders is based mainly on oral drug delivery administration, especially when patients stay at home and there is no need for hospitalization. Taking tablets or capsules is easy and noninvasive, thus, still remains most popular. However, there is a group of patients struggling or afraid of swallowing tablets (especially larger in size), including children, elderly patients, and those affected by Alzheimer’s disease or other neurological malfunctions. That limitation affects adherence and creates a risk that the patient could modify the dosage form (e.g. by cutting or crushing) in a way that may alter the drug’s pharmacokinetics and cause harm [Citation2]. Also, due to the complexity, mental disorders require special attention and individual treatment. Although active pharmaceutical ingredients (APIs) are available on the market in various doses, it is still challenging to select the appropriate one for individualized patient treatment. An additional issue is dosing in pediatric patients, who may need lower doses, that are not available on the market. That reveals the problem with dividing the pills to obtain the desired dose, and many parents, who chose to split the tablet, may unintentionally disrupt modified release profiles. Moreover, treating mental illnesses often requires polytherapy, and the dosing regimen may be confusing for patients, especially seniors suffering from cognitive problems and living alone. Those problems lead to poor adherence and worsen the condition of the patients. The omission of the dose or wrong dosing may lead to unsuccessful therapy and frustration, while overdosing may cause serious health problems and hospitalization. The limitations of conventional solid oral dosage forms are summarized in .
Currently, various dosage forms (e.g. tablets, injections, patches, nasal sprays) are available for mental or neurological disorders [Citation3–5]. Different routes of administration and modified release profiles intend to overcome limitations and improve patient’s adherence and comfort, but responding to individual needs, is still challenging. Currently, biochemical and genetic tests are increasingly used to determine how individual patients will respond to therapy [Citation6]. Such information enable treatment to be tailored precisely to the patient, but at the same time induce major manufacturing technology challenges.
Since three-dimensional printing (3DP) got involved in production of various dosage forms, it became clear that this emerging technology (ET) may be a real breakthrough for personalized medicines (PM). 3DP enables manufacturing of dosage forms with different shapes, complex structures, or layers with variety release profiles or combining multiple APIs at the same formulation [Citation7,Citation8]. It is also easier to obtain doses adjusted to patient needs, to meet the differences in metabolism and drug tolerance, especially for actives with a narrow therapeutic window. 3DP can also provide taste masking and thus increase patient’s adherence, which is quite problematic in the case of pediatric therapies [Citation9]. To improve children’s acceptability of the drug sweet-like formulations can be formed (e.g. chocolate [Citation10] or Spritam® sweets [Citation11]).
Recently, the matter of 3DP for neurological disorders was discussed by Gander et al. [Citation12] Their review presents applications of the 3DP in neurosurgery, like obtaining the models of tumors or anatomical structures for surgery planning or training, and using 3DP models to help educate patients about their health condition. Moreover, the authors emphasize that the 3DP can be used to manufacture customized instruments used in medical procedures or personalized implants, that facilitate repairs of bons in the skull. While the review by Gander et al. [Citation12] discusses the multidisciplinary use of 3D printing in neurodegenerative diseases and also touches on 3DP drugs, we focus on 3DP dosage forms and analyze in detail developments in the field of neurological and mental diseases.
Mental and neurological disorders are undoubtedly serious diseases, and need to be treated with a great cautiousness. Many mentally ill patients show poor adherence because it is hard for them to remember or understand the complex drug regimen. Also, comorbidity (psychosis, depression, migraine, etc.) is a common problem among epileptic patients, or in Parkinson’s disease which affects mostly elderly people with many other ailments. Moreover, the frequent dosing regimen in depression or schizophrenia may result in discouragement and therapy discontinuation. Attempts to overcome these challenges and develop a 3DP dosage form facilitating the therapy in these fields have been made.
Most of the advantages of 3DP techniques are universal, and applicable to other types of disorders. However, it is worth to emphasize these advantages in the therapy of mental and neurological disorders. For instance, it is essential that the drug dose for patient with epilepsy is accurate, and personalized dosage forms could improve the safety and efficiency of the treatment. Patients with Parkinson’s disease often have problems with swallowing, so 3DP can help create a dosage form suitable in size and shape for an individual patient. Also, patients who have poor adherence due to the mental disorders can benefit from polytablets designed specifically for them, in accurate dose and API combination. Moreover, the 3DP polytablet can be adjusted if the doctor changes the dose of a single drug for a patient.
The aim of this expert opinion is to provide a critical evaluation of recent literature examples of 3D-printed oral dosage forms in the field of psychiatric and neurological disorders. Including advantages, disadvantages, and regulatory considerations.
2. 3D printing in the field of psychiatric and neurological disorders
Additive manufacturing (AM) technology, commonly called 3DP, has been used in various industries for decades. Since the first commercially available AM method appeared in 1986, dozens of 3DP methods have been developed [Citation13]. The AM methods could be classified into inkjet-based, extrusion-based, and laser-based. ().
Figure 2. 3D printing methods, divided by inkjet, extrusion, and laser based: drop on demand (DoD); semi-solid extrusion (SSE); fused deposition modelling (FDM); stereolithography (SLA); digital light processing (DLP); liquid-crystal display (LCD); selective laser sintering (SLS). Created with BioRender.com.
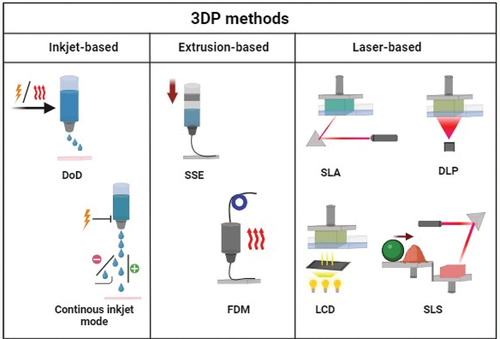
In the first class mentioned, AM process uses lasers to form the desired product. The ‘oldest’ laser-based technique is stereolithography (SLA) [Citation14]. It utilizes photopolymerization for solidification of a liquid resin. Digital Light Processing (DLP) and ultraviolet (UV) liquid-crystal display (LCD) are similar, newer techniques. The difference between these methods is that SLA uses the laser beam for the polymerization, while in DLP, a digital light projector is employed to cure the whole bottom layer of the resin, whereas LCD employs the liquid crystal display [Citation15]. Postprocessing includes cleaning of the product by washing it in a suitable solvent, such as Isopropyl alcohol (IPA) and exposing to light [Citation16]. The main advantage of AM is high accuracy of the shape and dimensions of the product [Citation17]. Moreover, the processed material is not subjected to significant temperature changes during manufacturing. However, possible drug sedimentation must be taken into account in terms of homogeneity in the final product [Citation18].
Another laser-based method is Selective Laser Sintering (SLS). In that process, a fine polymer powder is applied layer-by-layer (LbL) to the printing chamber, and a laser is used to fuse the powder particles. The most promising materials for pharmaceutical applications of SLS method include thermoplastic polymers such as polylactic acid (PLA), poly-L-lactide (PLLA) or polycaprolactone (PCL) [Citation19–21]. SLS has a higher printing resolution than other AM processes and does not need solvents. However, high processing temperatures might affect the drug stability [Citation16].
The second class of 3DP techniques is represented by inkjet printing, also known as binder jetting. According to the properties of the printhead, it can be divided into two types. The ‘older’ one is continuous inkjet mode, where the liquid flows out of the nozzle continuously and breaks into droplets. Another method, the drop on demand (DoD), is more popular and employs piezoelectric or thermal actuation to form a drop only when needed. Based on inkjet printing, the drop-on-powder (DoP) technique was developed. The process consists of distributing a powder layer in the work-bed, onto which a printing head applies a binder (ink). The grains of powder are bond together and create the layer of a final product, which is then lowered into the powder bed, and a fresh layer of powder is spread on the surface [Citation22]. This method usually creates porous structures which may require further post-processing and cannot print hollow elements because the internal cavity will always contain powder. However, the loose powder can be entrapped in a shell and become the tablet’s infill [Citation23]. Also, the type of powder and binder can be changed during the manufacturing process to produce multilayer medicaments with different compositions [Citation24]. There are numerous examples of powders used in 3DP, already well-known in classical pharmaceutics, including microcrystalline cellulose, spray-dried lactose, maltitol, maltodextrin, polyvinylpyrrolidone (PVP), and hydroxypropyl methylcellulose (HPMC) [Citation25–27].
Material extrusion-based (MEX) methods create products by depositing a semi-plastic material on a work-bed. After extrusion, the material should solidify quickly to maintain the designed shape of the product. Fused Deposition Modelling (FDM), also known as Fused Filament Fabrication (FFF), is an example of the MEX method, in which the plasticization of the material is caused by its heating. The processed material is a wire commonly referred to as a filament. The filament can be obtained by hot-melt extrusion (HME) process using single- or twin-screw extrusion, which is often coupled with the FDM. In the HME technique, the blend of a drug and polymers fed into an extruder, then melted at elevated temperature and formed into a wire [Citation28]. During the 3DP process, the filament is pushed into a heated nozzle and leaves through a round hole with a diameter much smaller than the filament itself. The nature of the FDM process limits the available range of materials to the group of thermoplastics, which at the same time should be characterized by relatively low thermal shrinkage. The limitation is that filament processing (plasticizing) temperature in the FDM machine ranges from 180 to even 400°C, and drugs added to the filament must reveal high thermal stability [Citation29]. Products manufactured using the FDM method are characterized by high roughness and porosity, which can significantly affect the drug’s release rate [Citation30].
Another example of AM method based on material extrusion is semi-solid extrusion (SSE), also known as pressure-assisted micro-syringe (PAM). This technique is used especially for treating soft and delicate materials that do not require melting [Citation31]. The material used in manufacturing is often a plastic mass extruded through a syringe-based printhead onto a working table. Syringes used for this technique could be pre-filled and disposable, which helps to avoid cross-contamination of the printed material. On the other hand, optimizing and controlling the crucial process parameters like temperature, extrusion force or mixture viscosity is needed; for instance, if the viscosity is too high, the paste will not flow, but the product may collapse if it is too low [Citation32]. Pneumatic extruders can be found in more expensive machines, but syringe pump extruders are more often used. In 3D bioprinting, this method allows the extrusion of living cells in a hydrogel matrix. However, the lack of adequate self-support of hydrogels is a significant limitation [Citation33]. The FDM, DLP, or inkjet printing methods can also be used in 4D printing (4DP) [Citation34–36]; a type of AM that includes time as a fourth dimension (4D). It requires using stimuli-responsive materials that can transform their shape under stimulants such as temperature, light, or chemical reagents. The two most widely used groups of materials for 4DP are Shape Memory Polymers (SMPs; e.g. polylactic acid (PLA), (PVA), and stimuli-responsive hydrogels (e.g. poly(N-isopropylacrylamide) (PNIPAm)). Applying a certain trigger to a printed material causes changes in its shape, like self-folding or gripping [Citation37].
2.1. Epilepsy
Studies employing 3DP techniques to obtain novel dosage forms with antiepileptic drugs focus mainly on rapid disintegration and fast onset of action, as well as high drug loading, which is explored frequently in the case of drugs with high therapeutic doses, like levetiracetam. A gap also exists in the personalized dosing of antiepileptic drugs. Children require different dosage from adults due to the difference in metabolism and possible adverse effects. On the other hand, elderly patients may require dosage adjustments in case of kidney or liver diseases. 3DP can also address the problem of the fluctuations in drug plasma concentrations. Creating the suitable release profiles can help avoid instabilities in drug concentration that may lead to crossing the therapeutic window borders and toxicity or therapy inefficacy.
The first and only, Food and Drug Administration (FDA) approved 3D-printed formulation is Spritam® that was introduced to the market in 2015 in the form of orally disintegrating tablets (ODT) with a drug content of 1000 mg. The applied manufacturing technology called ZipDose® enabled very fast product disintegration in the mouth within seconds, which is ideal for patients with swallowing difficulties [Citation38,Citation39]. Due to the still unclear regulations for the 3DP of medications, and difficulties in creating a scalable and efficient 3DP process, it is a challenge to get other 3DP drugs approved by regulatory bodies worldwide. However, recently the Triastek company received Investigational New Drug (IND) clearance from the FDA for three drugs, T19 (Rheumatoid Arthritis), T20 (Clotting Disorders) and T21 (Ulcerative Colitis). Triastek’s technology, called Melt-Extrusion Deposition (MED®) includes mixing powdered materials and semi-solids, extruding the mixture and printing dosage form LbL. The advantage of this process is one-step product fabrication, and the technology was accepted into the FDA Emerging Technology Programme (ETP) in 2020 [Citation40].
Currently, numerous examples of the use of other 3DP techniques for the production of antiepileptic drugs are described. Cui et al. [Citation41], obtained levetiracetam-loaded tablets of three different shapes and concluded that the semi-solid extrusion method (SSE) is suitable for manufacturing high drug-loaded tablets (96% w/w). Also, it was shown that printing lattice structure may even further accelerate the release of fast-release drug. The same group investigated cylindrical tablets with high levetiracetam loading using the same printing method [Citation42]. The SSE method allowed printing a dose-flexible system for easy adjustment regarding the individual dosing regimen. The authors observed linearity between the tablet mass and the volume of the model. The desired dose was obtained by printing the appropriate number of layers. However, the difference between the theoretical and actual number of printed layers of constant thickness in this method provided dosing inaccuracy. El Aita et al. [Citation43], presented a different approach to flexible dosing, as employed in a PAM technology. The linearity between the dose and the number of layers was observed, but the inaccuracy in dosing also occurred due to the over-extrusion of the material; the authors indicated that non-ideal printing settings might be a reason. In another work, El Aita et al. [Citation44], focused on the crucial limitation of the SSE method, which is using organic solvents in the formulation. The authors successfully obtained levetiracetam tablets using only water. That approach influenced the drying process and led to the tablets’ shrinkage, but the structure remained stable. The dose individualization by the SSE method was also described by Sjöholm et al. [Citation45], as a tool to obtain veterinary products with gabapentin, and the product was designed as an alternative to compounded formulations for small pets. Hong et al. [Citation46], proposed binder jet 3DP technique for drug individualization and obtained multicompartment levetiracetam and pyridoxine-loaded dispersible tablets. Levetiracetam was placed in powders mixture, while pyridoxine was in an ink component, dosed accurately to the specific part of the tablet in printing process. The drug dose could be adjusted with the number of printing layers. The product was highly porous, and both APIs were released almost completely within 5 min during in vitro dissolution studies. The procedure had numerous advantages, including relatively small amounts of materials necessary to obtain tablets, precise dosing, and the potential for therapy personalization. However, the drying procedure at 30°C was inefficient, and in higher temperature pyridoxine migrated to the edge of the tablet forming a ‘coffee ring’. This issue was solved by applying a 40°C vacuum-embedding drying method. Li et al. [Citation47], also used binder jetting technique to manufacture instant-dissolving tablets of levetiracetam. The tablets were investigated in vivo, and the obtained results were compared to commercial product. Moreover, a pharmacokinetic model for children between 4 and 6 years old was built based on the model describing adults. In this way, guidance on dose adjustments based on the effective dose range was proposed.
As has been mentioned in section 1, 3DP techniques can provide proper solutions for patients with swallowing difficulties. Panraksa et al. [Citation48] described personalized dosage forms obtained by the SSE technique designed for patients suffering from dysphagia. The formulation consisted of 3D-printed fast-disintegrating tablets (FDTs) placed in dosing syringes. Before administration, the patient should draw specific amount of water into the syringe and thus cause tablet disintegration. However, it was also revealed that the viscosity of the liquid obtained after tablet disintegration inside the syringe was too low, which may pose a risk of choking. Another 3DP approach to ODTs was presented by Conceição et al. [Citation49], where the authors used SSE to prepare carbamazepine tablets. Hydroxypropyl-β-cyclodextrin (HPβCD), capable of forming complexes with hydrophobic drugs, disintegration agent, and porous tablet design were used to improve the time of the drug dissolution. As a result, 98.9% of the drug was released in 15 min. Orodispersible minitablets (ODMTs) with carbamazepine intended for pediatric use were described by Hu et al. [Citation50], who used SSE and the composition was optimized using Design of Experiment (DoE) approach. In contrast to the previous work by Conceição et al. [Citation49], about 75% of the drug was released within one hour. However, the lack of stirring during the experiment might have affected the result. Additionally, the experiments performed using electronic tongue allowed for the detection of bitter taste only at higher drug concentrations, and tested formulations most likely would be suitable for pediatric use.
3DP can also be used to obtain the sustained release (SR) dosage forms. Lamichhane et al. [Citation51], prepared floating SR tablets with pregabalin using the FDM. The flotation was achieved by entrapment of air inside the tablets with further addition of solid layers from top and bottom to prevent water access. In the optimized formulation, one side of the tablet remained partially open, in order to combine the advantages of both open and closed systems, enabling water to fill the tablet up to 25%. The dissolution studies revealed that the drug was released completely in 24 h and release profile was close to zero-order kinetics, however the efficiency of the system needs to be confirmed in further studies in vivo, on humans. It is worth mentioning that the efficiency of floating drug delivery systems is a controversial matter. From one point of view, the floating system can increase the gastric retention of the drug, reduce the fluctuations of plasma drug concentrations, and potentially improve the drug bioavailability [Citation52]. However, the gastric retention time, and the efficiency of the drug absorption is affected by the fasted or fed state of a patient, because in the fed state the stomach emptying is extended in comparison to the fasted state [Citation53]. Homaee Borujeni et al. [Citation54], obtained a product with zero-order kinetics release profile, but with different method of manufacturing. HME, followed by FDM, was employed to create ethyl cellulose (EC) and hydroxypropyl cellulose (HPC) based tablets with carbamazepine. The study showed that tablets manufactured from 2:1 ratio of EC to HPC are able to extend the release of drug over 24 h and follow a zero-order pattern. However, the drug loading capacity was found to be very low, only 11 mg for carbamazepine, while in the marketed products, the dose usually exceeds 200 mg. However, the proposed formulation can be suitable for other substances, with lower therapeutic doses such as antihypertensive drugs (e.g. amlodipine, bisoprolol). Tagami et al. [Citation55], focused on investigating the release profile of insoluble matrix tablets (ghost tablets), with acetaminophen, theophylline, and carbamazepine. The tablets were prepared by DLP. Poly (ethylene glycol) diacrylate (PEGDA) was applied as a matrix-forming agent, to obtain the drug release without physical disintegration of the tablets. It was found that light exposure time affected the tablet size; however, it did not affect the drug release rate significantly, which was most probably related to the lack of disintegration. Moreover, the drug release was slower in the case of formulations with higher polymer content and lower amounts of water. Furthermore, the authors created a predictive model of drug release using machine learning, based on variables regarding ink composition. Such models may potentially ensure the therapeutic efficacy of a drug printed for individual patients.
3DP brings great hope in the therapy of orphan disease which occurs in less than 5 patients per 10,000 people. In such cases, it is obvious that industrial-scale manufacturing is not profitable and 3DP can be an alternative, as it allows production of small batches. One of the orphan anticonvulsant drugs is rufinamide, applied in Lennox-Gastaut syndrome, a rare and severe form of childhood epilepsy. The drug displays low water solubility associated with its non-linear pharmacokinetics, and also quite high melting point which means that in filament extrusion it will most probably retain its crystalline form. However, according to Saydam and Takka [Citation56], 3DP process involving extruded filament can also provide some advantages, as the API is stable and does not display the tendency to recrystallize. The authors prepared rufinamide-loaded tablets using FDM involving polymer filament obtained by HME. The dissolution study was performed in comparison to the marketed product, Inovelon® (Eisai GmbH). In case of the printed tablet, due to the improved solubility of rufinamide, the dissolved amount after 6 h correlated with the dose, in the range of 100–1600 mg. For commercial tablets, this relationship was not linear. The described results are promising in terms of possible improvements of dose-response linearity. Further studies involving the described formulation presented by Saydam et al. [Citation57] indicate that 3D-printed product performed better in vivo using Wistar albino male rats. The drug incorporated in the printed tablets also revealed higher permeability in Caco-2 cell lines and lower cytotoxicity than the marketed product.
2.2. Schizophrenia
Treatment of schizophrenia is associated with low compliance. This can be due to serious and persistent side effects of medications or reluctance to start treatment. Also, for people suffering from schizophrenia, the therapy regimen should be simple and drug administration should be convenient so as not to cause confusion or discourage treatment [Citation58]. The available literature reports focusing on novel oral 3D-printed dosage forms describe tablets and orodispersible films (ODFs) loaded mostly with newer antipsychotic agents, including aripiprazole and olanzapine. Lee et al. [Citation59], presented aripiprazole-loaded ODFs obtained with the by FDM and classical solvent casting. The comparison of the same films prepared with different methods revealed that solvent-cast films had higher puncture strength. However, all investigated products had sufficient mechanical durability when compared to the marketed films, paper, foil, and soft tissue. It was also shown that 3D-printed films disintegrated faster than the products obtained with the solvent-casting method. Differences between printed and cast films were visible only at the initial stage of the experiment, and printed films displayed a higher drug release rate. However, at further steps of the experiment, both types of formulation were similar. The API occurred in an amorphous form in both types of film, which significantly impacted the dissolution rate compared to the crystalline drug. Oh et al. [Citation60], presented another example of an ODF with aripiprazole, by obtaining a formulation with pneumatic HME, so the mixture was printed directly, without creating a filament. Additionally, a Quick Response (QR) code was incorporated in film manufacturing. The applied technology may be used to incorporate useful information directly in the dosage form. As aripiprazole displays pH-dependent solubility, citric acid was added as pH-modifier. In all of the investigated films, the disintegration time did not exceed 30 sec. The dissolution study revealed that the addition of citric acid indeed increased the release rate of the drug, and in the case of the films with this component, burst release was observed. Further study is needed to examine the impact of the printed QR code regarding patient’s compliance, and to establish the scope of information and its printability in the QR code form.
Łyszczarz et al. [Citation61], analyzed the impact of the manufacturing method on the properties of ODFs with aripiprazole, by comparing electrospinning, solvent casting, and FDM printing. The physicochemical studies performed for the PVA films obtained with all three methods showed that cast and 3D-printed films were stable and contained aripiprazole in an amorphous form. The physical stability of the drug was lower in the case of the formulations obtained with the electrospinning method. These films were also the least elastic and stretchable and displayed the shortest disintegration time, as they also had the highest porosity and wettability. The dissolution study revealed that the drug was released faster from all formulations when compared to pure aripiprazole. In all films, the amount of the API released within 5 min was close to 90%. The same study was repeated for the formulations stored for a month in order to check the possible physical changes affecting the dissolution process. It was found that in 3D-printed and electrospun formulations, the release rate dropped significantly. However, the observed results were still better than in the case of pure aripiprazole. The films manufactured with the casting technique did not reveal significant changes in the dissolution profile, thus they were considered the most stable; however, exhibited the longest disintegration time exceeding 3 min. The printing of ODFs required multistep processing and employing the elevated temperature, which may not be suitable for thermolabile drugs. In contrast, electrospinning requires fluid that can carry the electric charge and has proper viscosity to form fibers. Solvent casting seems to be the simplest method, although it needs the addition of a plasticizer to obtain a flexible product.
Another antipsychotic drug incorporated in 3D-printed formulations was olanzapine. Similarly, to aripiprazole, it belongs to Biopharmaceutics Classification System (BCS) class II, which means it displays poor solubility in water and good permeability through biological membranes. Bhatt et al. [Citation62], described immediate-release tablets with olanzapine prepared with the FDM technique using HME filaments composed with Kollicoat® IR, a fast disintegrating polymer. A significant enhancement of the drug release rate was depicted by in vitro dissolution studies in the case of 3D-printed tablets, with 90% of the drug released within the first 10 min. The obtained effect was related to rapid water absorption and tablet disintegration associated with strongly hydrophilic properties of the applied polymer. A formulation, with amorphous olanzapine dispersed in hydrophilic polymers was described by Cho et al. [Citation63]. ODFs were prepared with a pneumatic HME, which is similar to the classical FDM technique but does not require the application of previously extruded filament. The polymer blend is heated, and the resulting elastic mass is 3D-printed into films directly without forming the filament. It was found that the API occurred in an amorphous form, as expected, and the obtained product disintegrated rapidly and released 88% of the incorporated drug within the first 5 min. This technique can improve the dissolution of poorly water-soluble drugs, fasten the manufacturing as it requires only one step, and reduce waste. However, the process requires elevated temperature to melt the powder mixture, so it may be unsuitable for thermosensitive drugs.
Reports focusing on novel formulations with haloperidol are also available in the literature. Haloperidol is first generation typical antipsychotic that has also been used in treating other psychiatric conditions, including bipolar disorder, delirium accompanying alcohol withdrawal and Tourette syndrome, among others [Citation64]. The drug reveals poor solubility in water and pH-dependent solubility. In the study described by Solanki et al. [Citation65], haloperidol was applied as a model drug for manufacturing rapidly released 3D-printed tablets. The tablets were prepared by the FDM method, and for the filament production HME process was employed. Two types of tablets, with 60% and 100% infill, were evaluated at pH 2.0 and 6.8. Both types released the API slower than the physical mixture and crushed extrudate. However, at pH 6.8 the physical mixture did not release the whole amount of the drug that was related to its limited solubility. The infill value affected the drug release rate. Even though the investigated tablets released the API slower, still met the criteria for immediate-release dosage forms. Haloperidol was also used as one of model drugs in PVA-based tablets obtained by FDM 3DP [Citation66], where dissolution of crushed extrudate, physical mixtures and 3D-printed tablets was tested. The drug diffused from the tablets at a higher rate in acidic conditions. Another factor affecting the process was the infill value, and the drug release was faster in tablets with lower values, as they revealed higher porosity.
2.3. Insomnia
Personalized flexible-dose formulations obtained with 3DP methods can also be used in withdrawal therapies. Henry et al. [Citation67], obtained zolpidem-loaded caplets (oblong tablets) using the FDM involving various immediate-release polymers. The described method and selected matrix composition were found to be useful for printing caplets with a wide range of doses (<1 mg to 10 mg), potentially valuable for the withdrawal therapy. The nozzle diameter and overlap were essential for the product. Higher nozzle diameter led to less object precision, and lower overlap caused deformations. Notably, the overlap did not affect the drug dissolution profile, while wider nozzle size delayed the release. The advantage was that the range of doses could be obtained from the same filament. However, neither for the largest tablet nor the smallest one the requirement for releasing 85% of the drug in 15 min was met. Regardless of such modifications as addition of channels, no dissolution rate increase was observed, which probably was related to the low infill value of the caplets. The addition of the disintegrants failed to reduce the release time, which might be due to the high polymer content.
Oral formulations with different shapes were prepared with the DLP technique by Adamov et al. [Citation68]. In this case, depending on the shapes, dimensions, and concentration of the API, tablets, and tiles (cuboid tablets) released either 85% of the incorporated dose within 15 min or at least 80% within 45 min. The DLP technique allows printing without applying high temperatures; however, every time the drug stability should be considered due to the use of light in curing of the product. Additionally, the tests showed up to 120% of the drug released, which clearly revealed the potential problem of non-uniform drug distribution in the photoreactive suspension, and further research in that field is needed.
Another customizable formulation loaded with zolpidem was investigated by Callede et al. [Citation69]. PAM technique was proposed for the manufacturing of printlets. In the dissolution test, the analyzed formulation released the entire amount of the active ingredient within 45 min, regardless of the incorporated dose. The PAM method had several advantages that might be useful in printing dosage forms in hospital or community pharmacy. The mixture for printing could be obtained using simple utensils such as mortar and pestle. Moreover, in the production process, the cross-contamination risk can be easily eliminated by the use of a single disposable syringe for each formulation, which is a huge advantage of the applied method over other 3DP techniques. However, it should be mentioned that environmental conditions such as temperature or humidity can influence the rheological properties of the printing mixture and lead to inaccuracy. Notably, in the mentioned study, the mass of the wet printlets had a linear correlation with the mass of the dry printlets, and the drug amount. That observation could help pharmacist to assess the printed dosage form accuracy without complex analytical methods. However, the method limitations do not allow for printing of every shape of the printlet, like the sphere shape, because of the lack of support for the lower layers of the mixture. It is also worth mentioning that, due to the equipment limitations, the height of the model, which determined the drug loading, could be changed only by changing the diameter of the tip, and the dosage form should be designed carefully as the printer could only print whole layers which should fit the model height. To print the accurate dose, the height of the model had to be precisely determined in the software. Having specific nozzle tips to choose from, the height of the model had to be divided by the height of a given layer to obtain an integer, otherwise deviations in the API dose could occur.
Another approach was presented by Tabriz et al. [Citation70]. FDM was employed to manufacture LEGO®-like compartments with melatonin, which were combined with caffeine-loaded parts and pH-dependent placebo compartments, assembled into round tablet ().
Figure 3. Step by step assembly process of the LEGO® like solid dosage forms in (a) CAD and (b) 3D printed format, (c) in silico assembly of the individual compartment in the X-ray CT space-ray CT space; reprinted from Tabriz et al. [Citation70].
![Figure 3. Step by step assembly process of the LEGO® like solid dosage forms in (a) CAD and (b) 3D printed format, (c) in silico assembly of the individual compartment in the X-ray CT space-ray CT space; reprinted from Tabriz et al. [Citation70].](/cms/asset/e85d69e4-0bbc-4204-8337-36c2166470fa/iedd_a_2292692_f0003_oc.jpg)
The presented 3D-printed dosage forms had tailored melatonin and caffeine release profiles. Melatonin was released rapidly, while the release of caffeine was modified by using the hydrophilic or pH-dependent polymers in the filament and manipulating the thickness of placebo compartments. The FDM technique and LEGO®-like approach allow versatility in adjustment of a drug dose and release profile. According to the authors, the amount of drug can be adjusted by increasing the tablet diameter. Also, the placebo compartment can be modified by changing its thickness or replacing the polymer used with another polymer having different properties (e.g. more soluble or swellable). However, the increasing of tablet dimensions has its limits, so the tablet still could be swallowed by a patient. Also, the manufacturing process is even more complex and time-consuming comparing to the one compartment tablets obtained by 3DP. Considering that the time is essential for profitable manufacturing, time-consuming production is a significant limitation.
2.4. Depression
A printed delivery system can be used for different antidepressants to provide personalized dosage forms and sustained-release profiles for effective therapy. Also, an appropriate orodispersible form can be chosen for a patient struggling with swallowing tablets and capsules. Zhao et al. [Citation71], presented an intragastric tablet able to float on the gastric juice surface. That approach helps release the drug over a prolonged time without disturbing the peristalsis. In this study, an air chamber was added to the tablet to provide floating ability. Moreover, the insoluble shell was used to prolong the drug release time, and the size of the window in the tablet controlled the release rate ().
Figure 4. Model image of an intragastric floating and sustained-release tablet with an air chamber; reprinted with permission from Zhao et al. [Citation71].
![Figure 4. Model image of an intragastric floating and sustained-release tablet with an air chamber; reprinted with permission from Zhao et al. [Citation71].](/cms/asset/8ae9c798-f16b-4970-83e1-0bc27072ca31/iedd_a_2292692_f0004_oc.jpg)
Venlafaxine hydrochloride was employed as a model drug, and the HME technique and dual FDM 3DP were used. The whole structure consisted of a core containing the API and HPMC polymer, and an insoluble shell made of PLA with an air chamber and drug release window with a 1.5 to 4.5 mm radius. The most satisfactory drug release profile showed the tablet with a window radius of 4.5, presenting the sustained release profile for 24 h, compared to a shell-less tablet releasing 100% of the loaded drug in 4 h. Moreover, the core-shell tablets presented a release profile closer to zero-order than shell-less ones. That can be explained by absorption of water, dissolution of the drug, and its release layer-by-layer. However, it should be mentioned that empty PLA shells remain after HPMC erosion. Modifying the shell thickness or using PLA of lower molecular weight should be considered to avoid the risk of any intestinal obstruction.
Figueiredo et al. [Citation72], employed HME and FDM to create a paroxetine (PRX) loaded tablet. A physical mixture of a drug, HPC, and adjuvants (e.g. dicalcium dihydrate phosphate, magnesium stearate, and triethyl citrate) prepared in a mortar and then extruded. The filaments were used to print tablets by the FDM method. With the decrease in printing speed or traveling speed, the average mass of the tablet was lowered, probably due to flow constraints and impaired material deposition. Also, reducing the infill resulted in a decreased average mass. However, temperature decrease or batch size increase caused the increase of the mass. The drug content of all experimental conditions was found to be stable. The lowest tested printing temperature, the highest speed, and the thinner layer may lead to incomplete amorphization of a drug. The authors indicated that the API is in a crystalline state in the physical mixture and, mostly, in an amorphous form in filaments and printed tablets, but the results were not entirely conclusive. The dissolution studies showed sustained-release profiles with 100% drug released and plateau after 8 h. Notably, the amorphization did not impact solubility in this case. The tablet printed at 220°C showed faster release than tablets manufactured in lower temperatures. That demonstrates the impact of the instrumental parameters on printed dosage form properties.
Another study aimed to improve patient’s compliance by introducing a mirtazapine (MTZ) orodispersible film (ODF). In this task, syringe extrusion 3DP was employed, and the films were later compared to those obtained by the solvent-casting method. To prepare the ODF dosage form, MTZ was dissolved in a previously prepared solution of hydroxypropyl methylcellulose E15 in water and ethanol, with the addition of citric acid monohydrate. Then, films were printed (3D-MTZ) or cast (C-MTZ). 3D-MTZ showed a faster disintegration time than C-MTZ, which may be caused by a more porous structure. Moreover, both films represented longer disintegration time when loaded with MTZ than in the placebo version. Both the syringe extrusion 3DP and casting method were able to produce ODFs with drug content complied with the USP specification. The 3D-MTZ and C-MTZ did not differ significantly regarding the percentage of drug release, which was more than 80% in 5 min, although the 3D-MTZ had a faster disintegration time. Further research is needed to investigate the ODF’s stability and dosage personalization options [Citation73].
2.5. Parkinson’s disease
Dose personalization is also essential in the treatment of Parkinson’s disease (PD). Individualised doses could provide better control on reducing the symptoms of the disease. Another challenge is polytherapy, which is common among these patients, as Parkinson’s disease often affects elderly people with comorbidity. Even Parkinson’s therapy itself often requires more than one substance. Moreover, most of the patients suffering from PD struggle with dysphagia, which not only affects the quality of life but also may result in malnutrition, aspiration pneumonia or problems with swallowing medications [Citation74].
Windolf et al. [Citation75], presented a Mini-Floating-Polypill for PD, containing three drugs of different release kinetics, made by FDM ().
Figure 5. Printed polypills in various designs; reprinted from Windolf et al. [Citation70].
![Figure 5. Printed polypills in various designs; reprinted from Windolf et al. [Citation70].](/cms/asset/e0618be0-bbce-4f55-be11-17cac179e085/iedd_a_2292692_f0005_oc.jpg)
The first filament produced by HME contained levodopa (LD), benserazide (BZ), and ethylene-vinyl acetate (EVA) copolymer as a matrix for sustained release. The second was composed of dopamine agonist pramipexole (PDM) and polyvinyl alcohol (PVA) for fast release. The authors printed different geometries (two-layer, three-layer and hollow cylinder) with various drug contents from 50/12.5 mg to 200/50 mg LD/BZ, but notably, keeping the surface area to volume (SA/V) ratio quite similar allowed to obtain comparable release profiles. Also, the formulations successfully floated due to the air entrapped in structure and low EVA density, but the weak point was that the time of complete release was very long, which might be caused by the selected polymer. Also, the size of the printed tablets seemed to be unsuitable for PD patients suffering from swallowing problems.
To facilitate that challenge, a mini tablet (MiniTab) with a dimension of 4 mm was manufactured.
The doses were set at a low level, so the amount of the drug might be adjusted by taking a few mini pills. Also, in this case, LD/BZ release was similar to previously investigated tablets. To increase the fluid circulation and thus the release rate, the geometry of the mini-hollow cylinder (MiniHC) with 6 mm in dimension and increased SA/V ratio was created; thus, the LD/BZ release was improved compared to MiniTab. MiniHC could also float and was easier to swallow than previously printed tablets. This study demonstrates that 3DP allows for changing the release profile and adjusting it for a patient by using variables like SA/V ratio, selection of polymers or tablet geometry.
The influence of variables on drug release profile was also investigated by Windolf et al. [Citation76]. The hollow cylinder-based (HCb) geometry model of the 3DP dosage form was printed using FDM. The study included drugs of BCS class I, and BCS class II, in combinations with water-soluble and insoluble polymers. The hollow cylinder model was resized without the SA/V ratio change, and the authors evaluated the range of wall thickness, height, and pore diameter in which the release profiles remain similar. Regarding the drug BCS II and water-soluble polymer composition, it was indicated that in the HCb geometry model, 7.5-fold dose variation is possible without changing the dissolution profile, compared to 8-fold for water-soluble polymer and API BCS I, and 5-fold for water-insoluble polymer and API BCS I. It is worth mentioning that when the polymer is swelling during the dissolution process, the SA/V ratio changes. Swelling also influences the pore size and their contact with water. That is essential particularly for BCS II drugs which have lower solubility than BCS class I drugs. Another conclusion is that the dosage forms with the water-insoluble matrix are more sensitive to SA/V changes. Also, the pore size had a substantial influence on the release since the insoluble polymer limits the water contact with the drug.
Another personalized dosage form, a 3D-printed tablet with pramipexole, suitable for once-daily use due to the extended-release, was introduced by Gültekin et al. [Citation77]. The FDM method was employed for manufacturing, and 12 formulations of drug and polymer were obtained by HME. In vitro release studies were performed for printed tablets and tablets available on the market. Almost all prepared tablets showed extended release of up to 24 h. Moreover, printed tablets in doses 0.375 mg, 1.5 mg, and 3 mg showed a similar release profile to marketed products at the same dose. The researchers emphasized that this result was obtained without many tablet excipients that marketed tablets contain. In vivo studies on an animal model were also conducted. One group of rats received marketed tablets, while the other group 3D-printed tablets. The results showed no significant difference between the pharmacokinetic parameters of both formulations. The 3D-printed tablet was suitable for once-daily use like the marketed one. Gültekin et al. [Citation78], intended to manufacture pramipexole immediate-release tablets with different dissolution profiles using FDM. HME was employed to obtain filaments from combinations of polymers. The tablets contained no disintegrants. The influence of geometry and infill on dissolution rate was investigated. The 3D-printed tablet consisting of Eudragit EPO (70%) and POLYOX WSR N80 (30%) showed the fastest drug release, more than 90% in 25 min. Tablets printed with two holes in the structure released the drug faster than tablets with one hole or without the hole, and the release rate was higher in two-hole tablets compared to a one-hole tablet. That could be explained by the increased surface for drug diffusion in the tablets with holes. Also, the infill % showed no impact on release profiles, but the release rate increased as the tablet thickness was lower. A similar result regarding infill % was obtained in another study that aimed to manufacture immediate-release 3D-printed tablets using FDM. The infill ratio demonstrated no influence on cumulative drug release. However, the increase in thickness resulted in an increased release. In this study also the polymer ratio was investigated and the increase of POLYOX N80 amount caused slower drug release. In the same work, tablets loaded with 0.25, 0.375, 0.5, 0.75 mg, and 1 mg of pramipexole were printed to compare their dissolution and investigate if the drug load influenced the tablet properties. It was shown that all release profiles were similar, with a release of 85% of pramipexole within 15–20 min, so the dose could be modified without changing the release profile [Citation79].
3. Regulatory considerations
In the case of 3DP, the technology has outpaced reality, and thus legal regulations in relation to printed drug forms are currently widely discussed. In 2014 arose the Emerging Technology Program (ETP), which enabled collaboration between industry representatives and the Emerging Technology Team (ETT), including members of all relevant FDA quality review and inspections programs. Both sides discuss technical and regulatory matters of developing and implementing a novel technology [Citation80].
European Medicines Agency (EMA) established a multidisciplinary group named Innovation Task Force (ITF). The team ensures coordination across the Agency, discusses innovations in medicines development with applicants, addresses the Agency’s committees regarding the impact of emerging therapies on regulatory requirements, and provides regulatory advice [Citation81]. A matter of regulations for 3DP was especially highlighted during the COVID-19 pandemic. European Commission published a document regarding conformity assessment procedures for 3DP and 3D printed products for medical applications in COVID-19. The document states that manufacturers of 3D-printed medical devices must ensure that products meet the requirements of the applicable European Union (EU) legislation. Medical devices are ruled by directives regarding medical devices, in vitro diagnostic medical devices and active implantable medical devices. Also, conformity assessment procedures, a technical file, EU declaration of conformity and CE marking are necessary [Citation82]. In 2017, FDA issued the Technical Considerations for AM Medical Devices. The document presented the technical considerations and recommendations for testing and characterizing additively manufactured devices and their components. The document includes two main parts. The first part is Design and Manufacturing Considerations (Section V), which refers to fulfilling Quality System (QS) requirements according to the device’s regulatory classification. At the same time, Device Testing Considerations (Section VI) indicates the type of information that should be included in applications or premarket notification submissions. Notably, this document presents nonbinding recommendations. Requirements for a device as well as a type of premarket submission should be found in regulatory classification [Citation83].
Another issue is the dependence of healthcare facilities on an uninterrupted supply chain. In 2021, FDA published a discussion paper on 3DP Medical Devices at the Point of Care (PoC) and encouraged comments from the stakeholders [Citation84]. Consultations on the topic of PoC were also made by Medicines & Healthcare products Regulatory Agency (MHRA) in the United Kingdom (UK) [Citation85]. PoC 3DP could increase safety in case of supply chain disruption and also enable printing patient-matched devices or anatomical models to facilitate surgical planning. Although the issue is under discussion, it is clear that regulatory agencies intend to meet the growing expectations in this field.
However, the recommendations for medical devices may be insufficient for manufacturing dosage forms with API. Currently, there are no guidelines for manufacturing drug dosage forms using 3DP. Among AM techniques, powder solidification, liquid solidification, and extrusion techniques were mentioned as the most promising for dosage forms manufacturing in the following years [Citation86], but creating general guidelines is impeded by a variability of AM techniques. Methods have different critical parameters that should be controlled during the manufacturing process and evaluated during the quality control, such as the strength of filament in FDM, reproducibility of a drop formation process in liquid solidification, or homogeneity of a blend in powder solidification. There are also differences in designing the dosage form and postprocessing for different methods. A further issue is adequate stability control of API, as in various methods, it is exposed to different stress conditions (e.g. in the FDM method, it is high temperature, and in vat polymerization, it is UV light). On the other hand, developing guidelines dedicated to the most popular and promising branches of AM is possible separately, considering crucial process parameters and product properties for each technique. Although it indeed requires a comprehensive analysis of each process.
A serious concern in case of the individualized dosage forms is also quality assurance and quality control to ensure the drug safety. Due to the small size of batches, statistical sampling of a product and classical approach of testing based on analytical methods are unprofitable. Taking samples for destructive quality tests would mean the need to produce larger batches than intended and expose to increased costs and additional production time. Considering this, a nondestructive method for the quality assessment should be established. Also, preferable it should be fast and simple, so it could be proceeded potentially in the healthcare facility or pharmacy by the well-trained personnel. The main aspects of the interests are dose verification and content uniformity evaluation.
As a solution for reliable product, a quality by design approach could be considered. This approach assumes that quality is built into the product at the production stage, and establishes a control strategy for all aspects of the manufacturing process.
Real Time Release Testing (RTRT) is a part of a Control Strategy. This approach bases on the deep understanding of the process and dependence between the process parameters, in-process material attributes and product attributes. Validated RTRT method should be in the agreement with the product specification, and the end product test, if performed. Once the RTRT method is established, and the relationship was demonstrated, the in-process attributes can serve as the predictions for the product attributes. RTRT consists of the control of relevant material attributes and the use of process controls usually using the process analytical technologies (PAT) tools [Citation87]. For instance, the PAT tools were used in the study by Trenfield et al. [Citation88]. Researchers described the ‘point-and-shoot’ approach as a nondestructive method for quality control of 3DP dosage forms. Raman confocal microscopy was used to assess the drug distribution within the dosage form, and near infrared spectroscopy was employed for a quantitative analysis. Authors developed and validated a model to predict the concentration of the drug in the dosage form.
4. Conclusions
Various types of 3DP like laser-based, extrusion-based or inkjet-based methods are being investigated in the field of psychiatric and neurological diseases to create dosage forms. Examples 3DP solid oral dosage forms in the field of mental and neurological diseases can be found in . Different approaches attempt to personalize the dosage form mostly in terms of flexible dose and desired release profile, but also some studies focus on improving patient’s compliance. However, each of the described manufacturing processes has specific limitations and so far, Spritam® remains the only 3D-printed medication on the market.
Table 1. Summary of the attempts to obtain 3DP solid oral dosage forms in the field of mental and neurological diseases.
5. Expert opinion
The manufacturing of 3D-printed dosage forms provides a personalized approach to patient therapy and convenience. The individual patient’s response to the treatment may require many dose corrections until the proper one is selected. The 3D-printed medications could be prepared in exact dose for particular patient, and easily adjusted, if the change of dose is needed during the therapy. Also, the proper selection of a printing method, materials (e.g. polymers, excipients), and tablet geometry allows for modifying the release profile and thus improving the patient compliance and safety. Interestingly, printed tablets can show similar or even more desirable release profiles compared to the marketed products. Especially patients in polytherapy could benefit from 3DP ability to combine drugs in one dosage form. These facilities are especially relevant in mental and neurological disorders, where the treatment is often complex. However, possible interactions in drug combination should be always considered. Two basic approaches are used in manufacturing a 3DP polypills: the sandwich layered approach and the segmented approach. In the sandwich layered approach, every drug is mixed with a polymer to create a separate layer. This effect can be achieved using the FDM, PAM or SLA method, and changing the filament, printing mixture or resin after each layer is completed. The second approach is a segmented approach, where the APIs and excipients are combined in each layer. This method requires an equipment with two nozzle head extruders, enable to print simultaneously. The arrangement of the compartments can help achieving desired release profile of each drug and avoiding interactions between APIs. The inner compartment is usually reserved for a sustain release drug, while the outer compartments are more suitable for intermediate release substances, due to the exposition to body fluids [Citation89]. To avoid APIs interactions, various excipients can be applied, for instance using different polymers in each compartment provides a lag time between release of the two substances to avoid the degradation of the API in the unfavorable pH environment, and potential interaction between APIs [Citation90].
3DP is flexible and not limited to traditional tablets. It enables printing of orodispersible tablets or films, and variety of modified-release dosage forms. Printing techniques allow for creating lattice structures, compartments, air chambers and other structure modifications in easy and reproducible way, which is unique for this type of manufacturing. Also, the process is quick, and it is possible to change the type of manufactured batch quite fast, compared to cleaning and rearming the traditional tablet machine. However, the scaling-up of some techniques like the FDM method could be problematic. The speed of creating a single tablet is lower compared to tablet machine. Also, in this method one tablet is printed at one time, and for a large series more printers would be necessary. That’s why the future implementation of 3DP would probably divide into two sectors. One could be scaling-up methods more suitable for industry like binder-jetting, and another would be an introduction of 3DP to hospitals or health-care facilities.
Printing medications in health-care facilities could help avoid sudden drug supply chain interruptions. The COVID-19 pandemic showed that even a well-planned supply chain is fragile to issues of a global scale. Drug shortage can occur due to the lack of API, but also with the disruption in transport and logistics. During the pandemic, many employees of the pharmaceutical sector (e.g. specialists, drivers) were unable to work due to the infection or quarantine. Also, pharmaceutical wholesalers were struggling with personnel shortages. Moreover, in such cases as an epidemic or pandemic, the logistics of supply may change to facilitate the large hospitals and healthcare facilities with the highest number of patients. That could cause delays and changes in the supply plan for smaller and rural hospitals and pharmacies. 3DP in health-care facilities or pharmacies could provide a short backup for emergencies such as shortages and delays and enable sustained access to the most crucial medications. In case of a long-lasting lack of APIs, both big pharma companies and facilities with 3DP background are helpless. However, in a situation when there are delays in the transport, a facility could print small batches for its patients from the substances they have in stock to save patients’ life. Indeed, the matter of the supply chain of raw materials for 3DP and its storage should be addressed simultaneously. It is obvious that not every hospital with a 3D-printer would keep the stock of every API. However, a healthcare facility that prints personalized medicines for patients, and specializes in a disease entity could potentially keep a stock of the most essential APIs for their patients. Also, in non-pandemic times, there might be a situation when medications are destroyed, lost during transportation, or transported in unfavorable conditions, for instance, in heat when a temperature control system fails. Then, the printing of the medication allows for a continuation of the therapy and waiting for another delivery. 3DP certainly cannot eliminate the risk of supply chain interruptions, but it can help to survive sudden and unpredictable situations like delays in the delivery of the drug product. Also, manufacturing a dosage form at hospitals could reduce the risk associated with transport and logistics failures.
On the other hand, scaling up to industrial size raises AM’s sustainability issue. The pharmaceutical industry should follow global trends and become greener. As 3DP of a batch is previously planned and the operator can count the amount of material needed, the process is not generating a lot of excess waste besides the supports, which are critical for the print. Still, smart product designing and choosing the proper printing method can limit the amount of supports. Moreover, the LbL characteristic of the process and re-use of raw materials while possible, leads to further waste reduction and more environmental-friendly manufacturing.
On-demand printing, especially in the case of pediatric patients or orphan diseases enables manufacturing the small batches of medications similarly to the compounding process, without scaling-up and generating high costs. On the other hand, the quality control of the manufactured batches is still being discussed. Dosage forms printed in hospitals or community pharmacies require validated protocols and staff training. The dosage form could be manufactured in the hospital pharmacy and treated like other compounded drugs. Nevertheless, the regulatory issues about 3D-printed drugs are still not clear. The establishment of committees and facilitating programs may be considered as a step forward to popularize 3DP as a tool in pharmaceutical manufacturing. However, the lack of clear guidelines in manufacturing process, quality control and legislation process may discourage companies to invest in 3D-pinted product development.
3DP is still evolving and expanding its abilities. The next generation of AM is 4DP. In this method, the 3D-printed objects change into another structure over time, while exposed to different stimuli like temperature, light or pH change. This feature is possible thanks to use of smart materials and allows to manufacture a dosage form which is able to change in a programmed way, in certain time, even after is it administrated to the patient. Potential applications of 4DP in medicine include manufacturing scaffolds or implants, tissue engineering, developing smart surgical or diagnostic tools. Moreover, it could be used to create drug delivery systems. These systems could respond for an environmental stimulus, and release the drug in a desired place enabling a targeted drug delivery, or releasing of the drug in response to changes in patients body (e.g. inflammation, fever). Another approach in AM is 5D-printing (5DP), also called 5-axis printing, in which the rotation in the X- and Y-axes is possible. Thus, curved layers are printed, and models could obtain better quality, while using less supports. Taking advantage of the ability to print curved shapes with high precision, 5DP can find application in orthopedics. Printing personalized implants or artificial bones may help restoring the defects or recovering from the fractures [Citation91]. From the combination of 4DP and 5DP techniques emerged the concept of 6D printing (6DP). This technique uses 5 degrees of freedom to print an object, as in the 5DP, but the ready structure can also react and change in response to the environmental stimulus, like 4DP objects. This creates an opportunity to use 6DP in medicine, probably to manufacture personalized, and more advanced medical implants or devices. Moreover, the strength and efficiency of the product can be improved, while printing with less waste [Citation92]. Also, 7D printing (7DP) is reported in the literature. It focuses on further enhancing printing efficiency along with reducing the amount of waste, which provides a more sustainable process [Citation93]. However, so far there are no examples of applications of the 7DP in medicine. All mentioned types of AM are expected to step into medicine, to facilitate the manufacturing of dosage forms, implants, or scaffolds.
Article highlights
3D-printed dosage forms provide a personalized approach to the therapy by adjusting the dose and tablet geometry for individual needs.
3D-printing techniques allow creating a large variety of structural modifications in an easy and reproducible way to achieve modified-release dosage forms.
Variety of 3D-printing methods have been investigated in the field of psychiatric and neurological diseases to create dosage forms.
Printing medications in health-care facilities could help avoid sudden drug supply chain interruptions, enable manufacturing small batches to treat orphan diseases, and produce more sustainable formulations.
Committees and programs are arising to facilitate the implementation of new technologies in pharmaceutical sector, but there is still an emerging need to establish regulatory and quality control guidelines in 3DP.
Declaration of interest
The authors have no relevant affiliations or financial involvement with any organization or entity with a financial interest in or financial conflict with the subject matter or materials discussed in the manuscript. This includes employment, consultancies, honoraria, stock ownership or options, expert testimony, grants, or patents received or pending, or royalties.
Reviewer disclosures
Peer reviewers on this manuscript have no relevant financial or other relationships to disclose.
Additional information
Funding
References
- World mental health report: transforming mental health for all. [Internet]. Geneva: World Health Organization; 2022. Available from: https://www.who.int/publications/i/item/9789240049338
- Schiele JT, Quinzler R, Klimm H-D, et al. Difficulties swallowing solid oral dosage forms in a general practice population: prevalence, causes, and relationship to dosage forms. Eur J Clin Pharmacol. 2013;69(4):937–948. doi: 10.1007/s00228-012-1417-0
- Lipp MM, Hickey AJ, Langer R, et al. A technology evaluation of CVT-301 (inbrija): an inhalable therapy for treatment of Parkinson’s disease. Expert Opin Drug Delivery. 2021;18(11):1559–1569. doi: 10.1080/17425247.2021.1960820
- Canady VA. FDA approves esketamine treatment for MDD, suicidal ideation. Mental Health Weekly. 2020;30(31):6–7. doi: 10.1002/mhw.32471
- Lee KC, Chen JJ. Transdermal selegiline for the treatment of major depressive disorder. Neuropsychiatr Dis Treat. 2007;3(5):527–537.
- Goetz LH, Schork NJ. Personalized medicine: motivation, challenges, and progress. Fertil Sterility. 2018;109(6):952–963. doi: 10.1016/j.fertnstert.2018.05.006
- Robles-Martinez P, Xu X, Trenfield SJ, et al. 3D printing of a multi-layered polypill containing six drugs using a novel stereolithographic method. Pharmaceutics. 2019;11:274. doi: 10.3390/pharmaceutics11060274
- Goh WJ, Tan SX, Pastorin G, et al. 3D printing of four-in-one oral polypill with multiple release profiles for personalized delivery of caffeine and vitamin B analogues. Int J Pharmaceut. 2021;598:120360. doi: 10.1016/j.ijpharm.2021.120360
- Tabriz AG, Nandi U, Scoutaris N, et al. Personalised paediatric chewable Ibuprofen tablets fabricated using 3D micro-extrusion printing technology. Int J Pharmaceut. 2022;626:122135. doi: 10.1016/j.ijpharm.2022.122135
- Karavasili C, Gkaragkounis A, Moschakis T, et al. Pediatric-friendly chocolate-based dosage forms for the oral administration of both hydrophilic and lipophilic drugs fabricated with extrusion-based 3D printing. Eur J Pharmaceut Sci. 2020;147:105291. doi: 10.1016/j.ejps.2020.105291
- Scoutaris N, Ross SA, Douroumis D. 3D printed “starmix” drug loaded dosage forms for paediatric applications. Pharm Res. 2018;35:34.
- Gander C, Shi K, Nokhodchi A, et al. A review of the benefits 3D printing brings to patients with neurological diseases. Pharmaceutics. 2023;15(3):892. doi: 10.3390/pharmaceutics15030892
- Souto EB, Campos JC, Filho SC, et al. 3D printing in the design of pharmaceutical dosage forms. Pharm Dev Technol. 2019;24(8):1044–1053. doi: 10.1080/10837450.2019.1630426
- Guo N, Leu MC. Additive manufacturing: technology, applications and research needs. Front Mech Eng. 2013;8(3):215–243. doi: 10.1007/s11465-013-0248-8
- Quan H, Zhang T, Xu H, et al. Photo-curing 3D printing technique and its challenges. Bioact Mater. 2020;5(1):110–115. doi: 10.1016/j.bioactmat.2019.12.003
- El Aita I, Ponsar H, Quodbach J. A critical review on 3D-printed dosage forms. CPD. 2019;24(42):4957–4978. doi: 10.2174/1381612825666181206124206
- Melchels FPW, Feijen J, Grijpma DW. A review on stereolithography and its applications in biomedical engineering. Biomaterials. 2010;31(24):6121–6130. doi: 10.1016/j.biomaterials.2010.04.050
- Goole J, Amighi K. 3D printing in pharmaceutics: a new tool for designing customized drug delivery systems. Int J Pharmaceut. 2016;499:376–394. doi: 10.1016/j.ijpharm.2015.12.071
- Zhang J, Vo AQ, Feng X, et al. Pharmaceutical Additive manufacturing: a novel tool for complex and personalized drug delivery systems. AAPS Pharm Sci Tech. 2018;19(8):3388–3402. doi: 10.1208/s12249-018-1097-x
- Duan B, Cheung WL, Wang M. Optimized fabrication of ca–P/PHBV nanocomposite scaffolds via selective laser sintering for bone tissue engineering. Biofabrication. 2011;3(1):015001. doi: 10.1088/1758-5082/3/1/015001
- Awad A, Fina F, Goyanes A, et al. 3D printing: principles and pharmaceutical applications of selective laser sintering. Int J Pharmaceut. 2020;586:119594. doi: 10.1016/j.ijpharm.2020.119594
- Guo Y, Patanwala HS, Bognet B, et al. Inkjet and inkjet-based 3D printing: connecting fluid properties and printing performance. RPJ. 2017;23(3):562–576. doi: 10.1108/RPJ-05-2016-0076
- Yu D-G, Branford-White C, Yang Y-C, et al. A novel fast disintegrating tablet fabricated by three-dimensional printing. Drug Dev Ind Pharm. 2009;35(12):1530–1536. doi: 10.3109/03639040903059359
- Yu DG, Yang XL, Huang WD, et al. Tablets with material gradients fabricated by three-dimensional printing. J Pharmaceut sci. 2007;96(9):2446–2456. doi: 10.1002/jps.20864
- Katstra WE, Palazzolo RD, Rowe CW, et al. Oral dosage forms fabricated by three dimensional printing™. JControlled Release. 2000;66(1):1–9. doi: 10.1016/S0168-3659(99)00225-4
- Lee K-J, Kang A, Delfino JJ, et al. Evaluation of Critical Formulation Factors in the Development of a Rapidly Dispersing Captopril Oral Dosage Form. Drug Dev Ind Pharm. 2003;29(9):967–979. doi: 10.1081/DDC-120025454
- Wang C-C, Tejwani (Motwani) MR, Roach WJ, et al. Development of near zero-order release dosage forms using three-dimensional printing (3-DP™) technology. Drug Dev Ind Pharm. 2006;32(3):367–376. doi: 10.1080/03639040500519300
- Dumpa N, Butreddy A, Wang H, et al. 3D printing in personalized drug delivery: An overview of hot-melt extrusion-based fused deposition modeling. Int J Pharmaceut. 2021;600:120501. doi: 10.1016/j.ijpharm.2021.120501
- Pietrzak K, Isreb A, Alhnan MA. A flexible-dose dispenser for immediate and extended release 3D printed tablets. Eur J Pharm Biopharm. 2015;96:380–387. doi: 10.1016/j.ejpb.2015.07.027
- Goyanes A, Robles Martinez P, Buanz A, et al. Effect of geometry on drug release from 3D printed tablets. Int J Pharmaceut. 2015;494(2):657–663. doi: 10.1016/j.ijpharm.2015.04.069
- Auriemma G, Tommasino C, Falcone G, et al. Additive manufacturing strategies for personalized drug delivery systems and medical devices: fused filament Fabrication and semi solid extrusion. Molecules. 2022;27(9):2784. doi: 10.3390/molecules27092784
- Díaz-Torres E, Rodríguez-Pombo L, Ong JJ, et al. Integrating pressure sensor control into semi-solid extrusion 3D printing to optimize medicine manufacturing. Int J Pharm X. 2022;4:100133. doi: 10.1016/j.ijpx.2022.100133
- Tashman JW, Shiwarski DJ, Feinberg AW. A high performance open-source syringe extruder optimized for extrusion and retraction during FRESH 3D bioprinting. HardwareX. 2021;9:e00170. doi: 10.1016/j.ohx.2020.e00170
- Zhang Q, Yan D, Zhang K, et al. Pattern transformation of heat-shrinkable polymer by three-dimensional (3D) printing technique. Sci Rep. 2015;5(1):8936. doi: 10.1038/srep08936
- Solis DM, Czekanski A. The effect of the printing temperature on 4D DLP printed pNIPAM hydrogels. Soft Matter. 2022;18(17):3422–3429. doi: 10.1039/D2SM00201A
- Cui C, Kim D-O, Pack MY, et al. 4D printing of self-folding and cell-encapsulating 3D microstructures as scaffolds for tissue-engineering applications. Biofabrication. 2020;12(4):045018. doi: 10.1088/1758-5090/aba502
- Chu H, Yang W, Sun L, et al. 4D printing: a review on recent progresses. Micromach. 2020;11:796. doi: 10.3390/mi11090796
- Fitzgerald S. FDA approves first 3D-Printed epilepsy drug experts assess the benefits and caveats. Neurology Today. 2015;15(18):26. doi: 10.1097/01.NT.0000472137.66046.b5
- Home - SPRITAM ® (levetiracetam) [Internet]. [cited 2023 Mar 1]. Available from: https://spritam.com/
- Triastec Inc. About Triastek [Internet]. [cited 2023 Jul 17]. Available from: https://www.triastek.com/
- Cui M, Pan H, Fang D, et al. Fabrication of high drug loading levetiracetam tablets using semi-solid extrusion 3D printing. J Drug Delivery Sci Technol. 2020;57:101683. doi: 10.1016/j.jddst.2020.101683
- Cui M, Li Y, Wang S, et al. Exploration and preparation of a dose-flexible Regulation system for levetiracetam tablets via novel semi-solid extrusion three-dimensional printing. J Pharmaceut sci. 2019;108(2):977–986. doi: 10.1016/j.xphs.2018.10.001
- El Aita I, Rahman J, Breitkreutz J, et al. 3D-Printing with precise layer-wise dose adjustments for paediatric use via pressure-assisted microsyringe printing. Eur J Pharm Biopharm. 2020;157:59–65. doi: 10.1016/j.ejpb.2020.09.012
- El Aita I, Breitkreutz J, Quodbach J. On-demand manufacturing of immediate release levetiracetam tablets using pressure-assisted microsyringe printing. Eur J Pharm Biopharm. 2019;134:29–36. doi: 10.1016/j.ejpb.2018.11.008
- Sjöholm E, Mathiyalagan R, Lindfors L, et al. Semi-solid extrusion 3D printing of tailored ChewTs for veterinary use - a focus on spectrophotometric quantification of gabapentin. Eur J Pharmaceut Sci. 2022;174:106190. doi: 10.1016/j.ejps.2022.106190
- Hong X, Han X, Li X, et al. Binder jet 3D printing of compound LEV-PN dispersible tablets: an innovative approach for Fabricating drug systems with multicompartmental structures. Pharmaceutics. 2021;13(11):1780. doi: 10.3390/pharmaceutics13111780
- Li X, Liang E, Hong X, et al. In vitro and in vivo bioequivalence study of 3D-Printed instant-dissolving levetiracetam tablets and subsequent personalized dosing for Chinese children based on Physiological pharmacokinetic modeling. Pharmaceutics. 2022;14(1):20. doi: 10.3390/pharmaceutics14010020
- Panraksa P, Zhang B, Rachtanapun P, et al. ‘Tablet-in-syringe’: a novel dosing mechanism for dysphagic patients containing fast-disintegrating tablets fabricated using semisolid extrusion 3D printing. Pharmaceutics. 2022;14(2):443. doi: 10.3390/pharmaceutics14020443
- Conceição J, Farto-Vaamonde X, Goyanes A, et al. Hydroxypropyl-β-cyclodextrin-based fast dissolving carbamazepine printlets prepared by semisolid extrusion 3D printing. Carbohydr Polym. 2019;221:55–62. doi: 10.1016/j.carbpol.2019.05.084
- Hu J, Fitaihi R, Abukhamees S, et al. Formulation and characterisation of carbamazepine orodispersible 3D-Printed mini-tablets for paediatric use. Pharmaceutics. 2023;15(1):250. doi: 10.3390/pharmaceutics15010250
- Lamichhane S, Park J-B, Sohn DH, et al. Customized novel design of 3D printed Pregabalin tablets for intra-gastric floating and controlled release using fused deposition modeling. Pharmaceutics. 2019;11(11):564. doi: 10.3390/pharmaceutics11110564
- Rajora A, Nagpal K. A critical review on floating tablets as a tool for achieving better gastric retention. Crit Rev Ther Drug Carrier Syst. 2022;39(1):65–103. doi: 10.1615/CritRevTherDrugCarrierSyst.2021038568
- Stops F, Fell JT, Collett JH, et al. The use of citric acid to prolong the in vivo gastro-retention of a floating dosage form in the fasted state. Int J Pharmaceut. 2006;308(1–2):8–13. doi: 10.1016/j.ijpharm.2005.09.036
- Homaee Borujeni S, Mirdamadian SZ, Varshosaz J, et al. Three-dimensional (3D) printed tablets using ethyl cellulose and hydroxypropyl cellulose to achieve zero order sustained release profile. Cellul. 2020;27(3):1573–1589. doi: 10.1007/s10570-019-02881-4
- Tagami T, Morimura C, Ozeki T. Effective and simple prediction model of drug release from “ghost tablets” fabricated using a digital light projection-type 3D printer. Int J Pharmaceut. 2021;604:120721. doi: 10.1016/j.ijpharm.2021.120721
- Saydam M, Takka S. Improving the dissolution of a water-insoluble orphan drug through a fused deposition modelling 3-dimensional printing technology approach. Eur J Pharmaceut Sci. 2020;152:105426. doi: 10.1016/j.ejps.2020.105426
- Saydam M, Timur SS, Vural İ, et al. Cell culture and pharmacokinetic evaluation of a solid dosage formulation containing a water-insoluble orphan drug manufactured by FDM-3DP technology. Int J Pharmaceut. 2022;628:122307. doi: 10.1016/j.ijpharm.2022.122307
- Wirshing DA, Buckley PF. Schizophrenia Treatment Challenges. Psychiatric Times. 2003;20. https://www.psychiatrictimes.com/view/schizophrenia-treatment-challenges
- Lee J-H, Park C, Song I-O, et al. Investigation of patient-centric 3D-Printed orodispersible films containing amorphous aripiprazole. Pharmaceuticals. 2022;15(7):895. doi: 10.3390/ph15070895
- Oh B-C, Jin G, Park C, et al. Preparation and evaluation of identifiable quick response (QR)-coded orodispersible films using 3D printer with directly feeding nozzle. Int J Pharmaceut. 2020;584:119405. doi: 10.1016/j.ijpharm.2020.119405
- Łyszczarz E, Brniak W, Szafraniec-Szczęsny J, et al. The impact of the preparation method on the properties of orodispersible films with Aripiprazole: electrospinning vs. Casting and 3D printing methods. Pharmaceutics. 2021;13(8):1122. doi: 10.3390/pharmaceutics13081122
- Bhatt U, Malakar TK, Murty US, et al. 3D printing of immediate-release tablets containing olanzapine by filaments extrusion. Drug Dev Ind Pharm. 2021;47(8):1200–1208. doi: 10.1080/03639045.2021.1879833
- Cho H-W, Baek S-H, Lee B-J, et al. Orodispersible polymer films with the poorly water-soluble drug, olanzapine: hot-melt pneumatic extrusion for single-process 3D printing. Pharmaceutics. 2020;12(8):692. doi: 10.3390/pharmaceutics12080692
- Rahman S, Marwaha RH StatPearls [Internet]. Treasure Island (FL): StatPearls Publishing; 2023 [cited 2023 Apr 18]. Available from: http://www.ncbi.nlm.nih.gov/books/NBK560892/
- Solanki NG, Tahsin M, Shah AV, et al. Formulation of 3D printed tablet for rapid drug release by fused deposition modeling: screening polymers for drug release, drug-polymer miscibility and printability. J Pharmaceut sci. 2018;107(1):390–401. doi: 10.1016/j.xphs.2017.10.021
- Wei C, Solanki NG, Vasoya JM, et al. Development of 3D printed tablets by fused deposition modeling using Polyvinyl alcohol as polymeric matrix for rapid drug release. J Pharmaceut sci. 2020;109(4):1558–1572. doi: 10.1016/j.xphs.2020.01.015
- Henry S, De Vadder L, Decorte M, et al. Development of a 3D-Printed dosing platform to aid in zolpidem withdrawal therapy. Pharmaceutics. 2021;13(10):1684. doi: 10.3390/pharmaceutics13101684
- Adamov I, Stanojević G, Medarević D, et al. Formulation and characterization of immediate-release oral dosage forms with zolpidem tartrate fabricated by digital light processing (DLP) 3D printing technique. Int J Pharmaceut. 2022;624:122046. doi: 10.1016/j.ijpharm.2022.122046
- Callede N, Masciotti T, Casettari L, et al. Development and evaluation of a 3D printing protocol to produce zolpidem-containing printlets, as compounding preparation, by the pressurized-assisted microsyringes technique. Int J Pharmaceut. 2022;621:121756. doi: 10.1016/j.ijpharm.2022.121756
- Tabriz AG, Mithu MS, Antonijevic MD, et al. 3D printing of LEGO® like designs with tailored release profiles for treatment of sleep disorder. Int J Pharmaceut. 2023;632:122574. doi: 10.1016/j.ijpharm.2022.122574
- Zhao X, Wei W, Niu R, et al. 3D printed intragastric floating and sustained-release tablets with air chambers. J Pharmaceut sci. 2022;111(1):116–123. doi: 10.1016/j.xphs.2021.07.010
- Figueiredo S, Fernandes AI, Carvalho FG, et al. Performance and paroxetine stability in tablets manufactured by fused deposition modelling-based 3D printing. J Pharm Pharmacol. 2022;74(1):67–76. doi: 10.1093/jpp/rgab138
- Chaiwarit T, Aodsab N, Promyos P, et al. Fabrication of Hydroxypropyl methylcellulose orodispersible film loaded mirtazapine using a syringe extrusion 3D printer. Sci Pharm. 2022;90(4):68. doi: 10.3390/scipharm90040068
- Suttrup I, Warnecke T. Dysphagia in Parkinson’s Disease. Dysphagia. 2016;31(1):24–32. doi: 10.1007/s00455-015-9671-9
- Windolf H, Chamberlain R, Breitkreutz J, et al. 3D printed mini-floating-polypill for Parkinson’s disease: combination of levodopa, Benserazide, and pramipexole in various dosing for personalized therapy. Pharmaceutics. 2022;14(5):931. doi: 10.3390/pharmaceutics14050931
- Windolf H, Chamberlain R, Quodbach J. Dose-independent drug release from 3D printed oral medicines for patient-specific dosing to improve therapy safety. Int J Pharmaceut. 2022;616:121555.
- Gültekin HE, Tort S, Tuğcu-Demiröz F, et al. 3D printed extended release tablets for once daily use: an in vitro and in vivo evaluation study for a personalized solid dosage form. Int J Pharmaceut. 2021;596:120222. doi: 10.1016/j.ijpharm.2021.120222
- Gültekin HE, Tort S, Acartürk F. An effective technology for the development of immediate release solid dosage forms containing low-dose drug: fused deposition modeling 3D printing. Pharm Res. 2019;36(9):128. doi: 10.1007/s11095-019-2655-y
- Gültekin HE, Tort S, Acartürk F. Fabrication of three dimensional printed tablets in flexible doses: a comprehensive study from design to evaluation. J Drug Delivery Sci Technol. 2022;74:103538. doi: 10.1016/j.jddst.2022.103538
- Emerging Technology Program [Internet]. 2022 [cited 2023 Mar 13]. Available from: https://www.fda.gov/about-fda/center-drug-evaluation-and-research-cder/emerging-technology-program
- Mandate of the EMA innovation task force (ITF) [Internet]. European Medicines Agency; Human Medicines Research and Development Support Division; 2014. Available from: https://www.ema.europa.eu/en/human-regulatory/research-development/innovation-medicines#ema’s-innovation-task-force-(itf)-section
- Conformity assessment procedures for 3D printing and 3D printed products to be used in a medical context for COVID-19 [Internet]. European Commission; 2020 [cited 2023 Mar 13]. Available from: https://ec.europa.eu/docsroom/documents/40562
- Technical Considerations for Additive Manufactured Medical Devices. U.S. Department of health and human Services Food and drug administration. Rockville, MD: Center for Devices and Radiological Health. Center for Biologics Evaluation and Research; 2017.
- U.S. Food and Drug Administration’s (FDA’s). Center for devices and Radiological health. Discussion paper: 3D printing medical devices at the point of care [Internet]. 2021 [cited 2023 Mar 15]. Available from: https://www.fda.gov/medical-devices/3d-printing-medical-devices/3d-printing-medical-devices-point-care-discussion-paper
- Consultation on Point of Care manufacturing [Internet]. [cited 2023 Mar 15]. Available from: https://www.gov.uk/government/consultations/point-of-care-consultation/consultation-on-point-of-care-manufacturing
- Committee to Identify Innovative Technologies to Advance Pharmaceutical Manufacturing, Board on Chemical Sciences and Technology, Division on Earth and Life Studies, et al. Innovations in pharmaceutical manufacturing on the horizon: technical challenges, regulatory issues, and recommendations [Internet]. WA (D.C): National Academies Press; 2021 [cited 2023 Mar 15]. p. 26009. Available from: https://www.nap.edu/catalog/26009
- Guideline on Real time release Testing (formerly guideline on Parametric release). European Medicines Agency; 2012. https://www.ema.europa.eu/en/documents/scientific-guideline/guideline-real-time-release-testing-formerly-guideline-parametric-release-revision-1_en.pdf
- Trenfield SJ, Goyanes A, Telford R, et al. 3D printed drug products: non-destructive dose verification using a rapid point-and-shoot approach. Int J Pharmaceut. 2018;549(1–2):283–292. doi: 10.1016/j.ijpharm.2018.08.002
- Kara A, Cerda JR, Yuste I, et al. 3D printing technologies for personalized drug delivery. Emerging Drug Delivery And Biomedical Engineering Technologies [Internet]. 1st ed. Boca Raton: CRC Press; 2023 [cited 2023 Nov 7]. p. 31–47. Available from: https://www.taylorfrancis.com/books/9781003224464/chapters/10.1201/9781003224464-3
- Ghanizadeh Tabriz A, Nandi U, Hurt AP, et al. 3D printed bilayer tablet with dual controlled drug release for tuberculosis treatment. Int J Pharmaceut. 2021;593:120147. doi: 10.1016/j.ijpharm.2020.120147
- Vasiliadis AV, Koukoulias N, Katakalos K. From three-dimensional (3D)- to 6D-Printing technology in orthopedics: science fiction or scientific reality? JFB. 2022;13(3):101. doi: 10.3390/jfb13030101
- Georgantzinos SK, Giannopoulos GI, Bakalis PA. Additive manufacturing for effective smart structures: the idea of 6D printing. J Compos Sci. 2021;5(5):119. doi: 10.3390/jcs5050119
- Srivastava S, Pandey VK, Singh R, et al. Recent insights on advancements and substantial transformations in food printing technology from 3 to 7D. Food Sci Biotechnol [Internet]. 2023 [[cited 2023 Aug 25]; Available from];32:(13).1783–1804. doi: 10.1007/s10068-023-01352-8