ABSTRACT
Background
Glaucoma is one of the major irreversible blinding eye diseases in the world. Reducing intraocular pressure (IOP) is the primary treatment option, and taking eye drops daily is the common method. However, short drug duration and poor bioavailability of eye drops may lead to unsatisfied therapeutic effects and inadequate patient compliance.
Methods
A brimonidine-loaded silicone rubber insert (BRI@SR@PT) was prepared by loading brimonidine into a surface-modified silicone rubber ring, followed by polydopamine/thermoplastic polyurethane coatings. The physical properties, in vitro cytocompatibility and drug release of BRI@SR@PT were investigated. The BRI@SR@PT was administrated in the conjunctival sac of rabbit eyes and its in vivo drug release, IOP-lowering efficacy and biosafety were assessed.
Results
The BRI@SR@PT presented great thermal stability and excellent elasticity. The BRI@SR@PT was able to release BRI sustainably for 28 days with little toxicity in vitro. Compared to BRI eye drops, the BRI@SR@PT effectively lowered IOP for 21 days based on the sustained BRI release with great biosafety when administrated in conjunctival sac of rabbit eyes in a noninvasive fashion.
Conclusions
The conjunctival sac insert (BRI@SR@PT), as a promising drug-delivery platform, may provide a sustained IOP-lowering treatment for patients with ocular hypertension or glaucoma, without need for invasive procedures.
Disclaimer
As a service to authors and researchers we are providing this version of an accepted manuscript (AM). Copyediting, typesetting, and review of the resulting proofs will be undertaken on this manuscript before final publication of the Version of Record (VoR). During production and pre-press, errors may be discovered which could affect the content, and all legal disclaimers that apply to the journal relate to these versions also.1. Introduction
Glaucoma is a chronic and irreversible eye disease characterized by structural changes of optic disc and progressive degeneration of retinal ganglion cells, and has become the second leading cause of blindness worldwide[Citation1–3]. Elevated intraocular pressure (IOP) is considered to be the major risk factor for glaucoma, and lowering IOP to slow down the degeneration of optic nerve cells is the primary method for glaucoma treatment[Citation4,Citation5]. Eye drops have gradually become the primary choice for IOP-lowering medications because of their convenient, safe, user-friendly and noninvasive characteristics[Citation6]. Nowadays, conventional IOP-lowering therapeutic drugs mainly include prostaglandin analogs, β-blockers, cholinergic agonists, carbonic anhydrase inhibitors and α-adrenergic agonists[Citation2]. As the third generation α-adrenergic agonists, brimonidine (BRI) can lower IOP by increasing the outflow capacity of uveoscleral pathway and decreasing the production of aqueous humor[Citation7], and also show a neuroprotective effect against retinal ganglion cell death[Citation8]. However, due to the tear film and intraocular anatomical barriers, eye drops generally present low drug bioavailability, and multiple and frequent administrations are needed, which may cause decreased patient compliance[Citation9]. Patient compliance is even more important for glaucoma treatment which requires long-term, lifelong medication. In addition, the short time of drug action and frequent fluctuation of drug concentration in aqueous humor will cause unstable IOP and unsatisfactory therapeutic effect on patients with ocular hypertension or glaucoma[Citation10].
To enhance drug penetration through the cornea barrier into eyes and improve drug bioavailability, researchers made various attempts to develop sustained drug delivery systems (DDSs), such as implants and inserts[Citation11–15], hydrogels[Citation16–18] and nanocomposites[Citation19–22]. To this day, there has been a DDS (Bimatoprost sustained-release implants, with a commercial name of Durysta) available for clinical applications[Citation23]. However, it may result in new cornea wounds and poor patient compliance. In order to improve the intraocular bioavailability of anti-glaucoma drugs and patient compliance, our team has designed and developed a kind of IOP-lowering drug-loading inserts with non-invasive and safe manner to sustainably deliver brimonidine into eyes and reduce IOP for up to 21 d[Citation24]. However, this non-invasive insert still presented an obvious initial burst drug release at day 1, which probably resulted in transient tissue toxicity and poor treatment durability. Therefore, we further developed the second kind of non-invasive insert with a surface coating of thermoplastic polyurethane (TPU) to inhibit the initial burst release and improve the sustained drug release profile[Citation25]. However, the weak adhesion of TPU coating on the silicone insert probably made the sample standardization imperfect. Thus, a well-adhesive coating material should be introduced to enhance the surface adhesion of TPU coating on the silicone insert, which can improve the sample standardization and provide more stable and sustained drug release. Polydopamine (PDA) is a novel coating material inspired by mussel adhesion proteins[Citation26,Citation27]. With the presence of oxygen, dopamine monomers in alkaline solutions can easily deposited on the surface of organic or inorganic materials and subsequently form a thin film, exhibiting a controlled thickness and excellent stability[Citation26,Citation28]. To date, PDA coatings have been widely used for surface modification of medical devices[Citation29–33] due to their good cell adhesion, chemical reactivity, biocompatibility and antimicrobial activity[Citation34,Citation35].
Therefore, in this study, we proposed to develop a two-component surface-modified IOP-lowering insert, BRI@SR@PT, which was expected to take advantage of the characteristics of both PDA and TPU to increase the standardization of sample preparation and biocompatibility of the inserts, and further improve the BRI release profile and IOP-lowering treatment effectiveness (). We conducted the characterization of BRI@SR@PT by scanning electron microscopy (SEM), Fourier transform infrared spectroscopy (FTIR), thermal analyses (TG-DSC), and tensile testing. We also investigated the in vitro drug release profile and examined the cytocompatibility of BRI@SR@PT on human and mice corneal epithelial cells (HCECs and MICECs) through CCK-8 test and Live/Dead staining assay. Moreover, we verified the histocompatibility and sustained IOP-lowering efficacy of the inserts after administrated in the conjunctival sac of rabbit eyes during the observation period.
Figure 1. Schematic illustration of the preparation of the BRI@SR@PT and its application on rabbit eyes. (A) The fabrication process of the BRI@SR@PT including modification, BRI-loading, PDA/TPU coating. (B) Administration of the BRI@SR@PT into the conjunctival sac of rabbit eyes. (C) Investigation of the IOP-lowering effect by detecting drug concentration in aqueous humor and measuring intraocular pressure (IOP). R.T., room temperature.
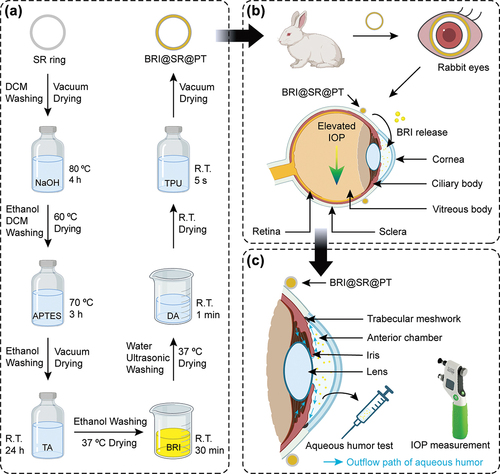
2. Materials and methods
2.1. Materials
All materials, chemicals and reagents were used as received without any further purification. Silicone rubbers (SRs) were obtained from Kangda Rubber Co., Ltd. (Shanghai, China) and brimonidine (BRI) was bought from J&K Scientific Ltd. (Shanghai, China). Aminopropyl triethoxysilane (APTES), dopamine hydrochloride (98% w/w), Tris buffer (1 M, pH 8.5) and sodium periodate (NaIO4) were purchased from Aladdin Reagent Co., Ltd. (Shanghai, China). N-hydroxysuccinimide (NHS), 1-(3-dimethylaminopropyl)-3-ethylcarbodiimide hydrochloride (EDC), tartaric acid (TA), Dulbecco’s modified eagle medium/Nutrient Mixture F-12 (DMEM/F-12) and fetal bovine serum (FBS) were all provided by Sigma-Aldrich (Shanghai, China). Thermoplastic polyurethane (TPU) was bought from Lubrizol Lifescience (Bethlehem, Palestine). Dichloromethane (DCM), tetrahydrofuran (THF), anhydrous ethanol and sodium hydroxide (NaOH) were obtained from Shanghai Lingfeng (Shanghai, China). Calcein acetoxymethyl ester (Calcein-AM)/propidium iodide (PI) double staining kit and cell counting kit-8 (CCK-8) assay were purchased from Dojindo Molecular Technologies, Inc. (Shanghai, China). Zoteil®50 (Virbac, Hamilton, New Zealand) and xylazine hydrochloride (Su-Mian-Xin II, Shengda Animal Drug Company, Jilin, China) were purchased and mixed (1: 2, v/v) for anesthesia of rabbits. Brimonidine eye drops (0.15% w/w, Allergan, USA) and oxybuprocaine hydrochloride eye drops (Santen, China) were used as purchased.
2.2. Preparation of BRI@SR@PT
2.2.1. Modification of SRs
The modification of SRs was performed according to the previous report[Citation24,Citation25]. Briefly, the SRs were washed 3 times with DCM (10 mL) to remove impurities and then dried under vacuum. Then, the SRs were immersed in NaOH ethanol-aqueous solution (4 M, 50% H2O, 100 mL) and stirred for 4 h (100 rpm) in an 80 ºC water bath (Thermostatic Magnetic Stirrer, DF-101Z, Shanghai, China). The obtained SRs were immersed in 50% ethanol solution (50 mL) for 10 min and then washed 3 times with DCM (10 mL) again. Subsequently, the SRs were put in 1% (w/w) APTES ethanol solution (80 mL) and stirred (100 rpm) for 3 h in a 70 ºC water bath. After washed with 50 mL anhydrous ethanol and dried (110 ºC, 3 h), the SRs were further vacuum-dried for 24 h. Then, the SRs were immersed in 100 mL TA solution (2.5 mM, 50% ethanol-water) with EDC (25 mM) and NHS (25 mM) for 24 h under room temperature. Finally, the obtained SRs were washed with 20% ethanol solution (50 mL) for 3 times and dried at 37 ºC for 24 h.
2.2.2. BRI-loading into modified SRs
Brimonidine (BRI) was solubilized in a mixture of methanol and DCM (1: 1, v/v) to achieve a 10 mg/mL solution. The modified SRs were incubated in the BRI solution for 30 min and then air-dried under a fume hood. This process was repeated three times to achieve the BRI loading. The above SRs loaded with BRI were rapidly sonicated in a small amount of water (5 mL) for 1 min (25 ºC, 53 kHz) to wash away the BRI crystals adhered on the SR surface and retain the internal drug molecules to the greatest extent, and the BRI@SRs were subsequently obtained after being dried at 37 ºC for 24 h.
2.2.3. Preparation of BRI@SR@PT
The BRI@SRs were coated with PDA and TPU to prepare BRI@SR@PT according to the previously reported method[Citation36]. Briefly, 10 mL of dopamine hydrochloride solution (DA, 80 mM in deionized distilled water (DDW)) was mixed with 10 mL of the oxidizer solution (200 mM in Tris buffer, 160 mM of NaIO4 and 5 mM of NaOH in DDW). The BRI@SRs were quickly immersed in the DA solution for 1 min, followed by drying at room temperature for 12 h. TPU was pre-dissolved in THF to make a 5% (w/w) solution. After a brief 5 second of immersion in the TPU solution, the PDA-coated BRI@SRs were air-dried in a fume hood for 1 h. Subsequently, the PDA and TPU-coated BRI@SRs were vacuum-dried for 24 h to remove the residual THF and obtained BRI@SR@PT.
2.3. Characterizations of BRI@SR@PT
To assess the surface and internal structures, the modified SR, BRI@SR, BRI@SR@PDA and BRI@SR@PT were examined using a digital camera and scanning electron microscopy (SEM, Zeiss Sigma 300, Oberkochen, Germany). The composition of the inserts was detected by a Fourier transform infrared spectroscopy (FTIR) spectrophotometer (Nicolet iS 20, Waltham, MA) and the test wavelength range was 500 - 4000 cm−1. Thermal analyses including thermogravimetric (TG), derivative thermogravimetry (DTG), and differential scanning calorimetry (DSC) were performed by a TG-DSC analyzer (Perkin Elmer, Waltham, MA). The tensile properties of the inserts were evaluated on a universal testing machine as the previously reported method (Instron Ernst Brinck, Canton, MA)[Citation24].
2.4. In vitro cytocompatibility of BRI@SR@PT
To assess the cytocompatibility of the inserts, the immortalized human and mice corneal epithelial cells (HCECs and MICECs) were used according to the previous report[Citation24]. HCECs were kindly provided by Shandong Eye Hospital (Prof. Weiyun Shi, Shandong Academy of Medical Sciences, Jinan, China). MICECs were bought from Ruian BioTechnologies Ltd. Co. (Shanghai, China). Briefly, the BRI@SR@PDA and BRI@SR@PT were immersed in the culture medium (0.2 g/mL culture medium, 10% FBS) for 24 h to obtain the leaching liquor. HCECs and MICECs seeded in 96-well plates at a density of 1.0 × 104 cells per well were cultivated overnight and then treated with the corresponding leaching liquor (0.1 mL per well). Following a further 24 h incubation, the leaching liquor was replaced with 100 μL of CCK-8 solution (10% in FBS-free DMEM/F-12) per well for 2 h. The cell viability was subsequently quantified through ultraviolet-visible (UV-Vis) absorbance measurement at 450 nm, performed with a microplate reader (Synergy H1 Hybrid Reader, BioTek, Winooski, USA).
In another 4 groups, HCECs and MICECs cultured in 96-well plates were stained using a Calcein-AM/PI double staining kit based on the reported methods[Citation37]. In brief, the Calcein-AM storage solution and PI storage solution were mixed in PBS (2 μmol/L of Calcein-AM, 4.5 μmol/L of PI) to obtain a final working solution. After washed with PBS twice, HCECs and MICECs were incubated with Calcein-AM/PI working solution (50 μL per well) away from light for 15 min. Each well was observed with a fluorescence microscopy (Leica, Germany) at 490 nm and 545 nm.
2.5. In vitro drug release from BRI@SR@PT
According to the previously reported method[Citation25], the drug release curves of the inserts (including BRI@SR, BRI@SR@PDA, and BRI@SR@PT) were evaluated. To be specific, each insert was put in a 50 mL centrifuge tube containing 10 mL of PBS to fully meet the sink condition (1.5 mg/mL at 25 ºC). These tubes were then placed in a shaker (DKZ-3B, Yiheng, Shanghai, China) at 37 ºC and 50 rpm. At the time point of 1, 4, 8 h and 1, 2, 4, 8, 14, 21 and 28 d, a total of 2 mL of the medium was exchanged with the same volume of fresh PBS. A UV-Vis spectrophotometer (NanoDrop 2000, Thermo Scientific, USA) was used to measure the absorbance of the medium at 246 nm to calculate the immediate BRI concentrations and the cumulative BRI release rates.
2.6. In vivo drug release from BRI@SR@PT
To ensure adherence to animal welfare regulations and ethical research principles, the researchers obtained ethical and legal approval (No. PZSHUTCM2307300004) from Shanghai University of Traditional Chinese Medicine (SHUTCM) prior to the study. With compliance to the Association for Research in Vision and Ophthalmology Statement for the Use of Animals in Ophthalmic and Vision Research and the guidelines of the Animal Care and Use Committee of Eye & ENT Hospital of Fudan University and SHUTCM, all animal experiments, encompassing transportation, care, and surgeries, were conducted ethically and responsibly. The experiment utilized male New Zealand rabbits (~2 kg) obtained from SHUTCM. Throughout the study, the rabbits were housed with unrestricted access to food, water and fresh air under standard conditions (25 ºC, 60% humidity, 12 h light/dark).
Rabbits were randomized into two groups (n = 5 rabbits per group): BRI eye drops and BRI@SR@PT. Anesthesia was attained through the intramuscular injection of a pre-mixed solution of Zoteil and xylazine hydrochloride (1: 2) at a dose of 0.1 mL/kg. Application of the insert or eye drops was performed on all right eyes of the rabbits depending on the group assignment, while all left eyes were used as the blank control. The BRI eye drops (0.15% w/w, 20 μL) were administrated into the right eyes per 10 min and for a total of five times[Citation24]. The BRI@SR@PT was placed in the conjunctival sac after the rabbit eyes were topically anesthetized with 0.5% (w/w) oxybuprocaine hydrochloride before the operations. After the insert or BRI eye drop was administrated, aqueous humor (100 μL) was obtained by paracentesis of the anterior chamber at the time point of 1, 4, 6, 24, 48, 96, and 192 h.
The obtained aqueous humor was mixed with methanol (1: 2) for 1 min and then centrifuged (16000 rpm, 10 min). The supernatant was obtained and the BRI concentrations were examined by an ultra-performance liquid chromatography-mass spectrometry (UPLC-MS/MS) system. The UPLC system (Waters Corporation, USA) was coupled with a Triple Quad 5500 triple quadrupole mass spectrometer. Chromatographic separation was performed on an ACQUITY UPLC BEH Amide column (100 × 2.1 mm, 1.7 μm; Waters Corporation, USA) with a column temperature of 40 ℃. The flow phase was a mixture solution consisting of 0.1% (w/w) formic acid in water (A) and acetonitrile (B) with a flow rate of 0.3 mL/min. The gradient was 0 - 0.5 min 5% B, 3 - 3.5 min 90% B and 3.6 - 5 min 5% B. The injected sample volume was fixed at 5 μL. The mass spectrometry detection was performed by positive electrospray ionization in multiple reaction monitoring (MRM) mode with the ion transitions monitored (m/z): 292.2→212.1 for BRI. The spray voltage was 4500 V and the ion source temperature was 500 ºC. The collision energy was set as 31 eV.
2.7. IOP-lowering effectiveness and biosafety of BRI@SR@PT
The effectiveness of BRI@SR@PT in lowering IOP and their biosafety were further investigated based on two other groups (n = 5). IOP was measured at the regular time intervals (0, 0.5, 1, 2, 4, 6 h and 1, 2, 4, 6, 8, 14, 18, 21 and 28 d) after the administration of either the insert or BRI eye drops (0.15% w/w), using an Icare® TONOVET Plus rebound tonometer (Icare Finland Oy, Helsinki, Finland). Each eye was measured four times repeatedly. During the observation period, rabbit anterior segment photographs were taken at 0, 7, 14 and 28 d. After 28 d of monitoring, the study reached its endpoint. To facilitate the detailed tissue examination, the rabbits were euthanized with an anesthetic overdose. Subsequently, their eyeballs were carefully collected and processed through a series of steps: fixation in 4% paraformaldehyde, paraffin embedding, sectioning into 3 µm-thick slices, and finally staining with hematoxylin and eosin (H&E) to reveal tissue details. The sections were examined under a microscope (Zeiss, Germany) to obtain representative images for analyses. The tissue toxicity of the BRI@SR@PT and BRI eye drops were evaluated by analyzing the taken images.
2.8. Statistical analysis
Each experiment was conducted in triplicate independently. All the results were calculated as mean ± SD. All analyses were performed using GraphPad Prism 9.0 (GraphPad Software, San Diego, CA, USA). The statistically significant difference among different groups was determined by two-way ANOVA, and p < 0.05 was considered statistically significant. Specifically, the multiple unpaired t-test was used for the IOP comparison.
3. Results and discussion
3.1. Characterizations of BRI@SR@PT
The structure and morphologies of the inserts (including the modified SR, BRI@SR, BRI@SR@PDA and BRI@SR@PT) were investigated using a digital camera and SEM. As shown in , all inserts had similar diameters before and after BRI-loading, PDA and TPU coating. BRI-loading turned the modified SR into the yellow BRI@SR, and then into the brownish BRI@SR@PDA after the PDA coating. In this course, sodium periodate was used as an oxidant to accelerate PDA deposition on the BRI@SR surface and reduce drug loss[Citation36]. Furthermore, SEM images showed that the modified SR presented a smooth surface, while the BRI@SR after BRI-loading gave a rough surface. This might result from the residual drug crystals attached on the BRI@SR surface though most of the BRI crystals were washed away by sonication (). The PDA-coating on BRI@SR made the rough surface covered by a dense film (BRI@SR@PDA), and the TPU-coating made the dense film further covered by a loose porous film (BRI@SR@PT). Moreover, the average diameters of the cross-section of the modified SR, BRI@SR, BRI@SR@PDA and BRI@SR@PT were 1400.7 ± 41.0 μm, 1402.3 ± 22.8 μm, 1400.51 ± 26.7 μm and 1427.7 ± 21.4 μm, respectively (). It was indicated that BRI-loading, PDA and TPU-coating had little effect on the cross-section diameter of the inserts. After TPU-coating, a distinct film was observed tightly covering the surface of BRI@SR@PT (), which was different from the previous report in which the BRI@SR was coated with TPU alone[Citation25]. Thus, it was obvious that PDA improved the adhesion of TPU on the SR surface, which increased the standardization of sample preparation.
Figure 2. Morphologies of the modified SR, BRI@SR, BRI@SR@PDA and BRI@SR@PT. (A) Representative images of the inserts before and after surface modification, drug loading and PDA/TPU coating captured by a digital camera. (B-C) Surface and cross-section SEM images of the inserts.
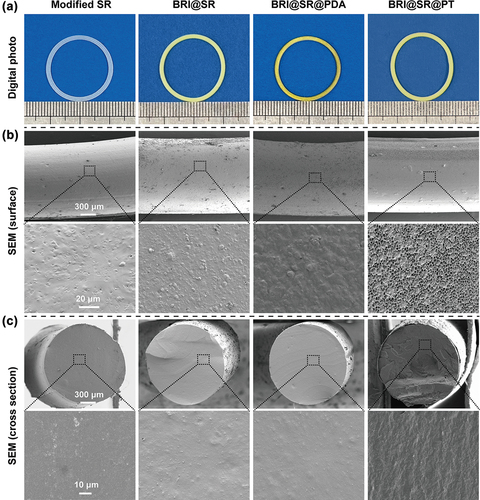
The surface modification of the SRs, BRI-loading and PDA/TPU coating on the BRI@SRs were determined by FTIR analysis. In , the FTIR spectra confirmed the successful surface-modification of the SRs by NaOH, APTES and TA. The characteristic absorption peaks of the SRs were observed at 2962, 1258, 1004 and 784 cm−1, indicating the presence of the stretching vibration of C−H groups, the bending vibration of Si−CH3 groups, and the stretching and bending vibration of Si−O−Si groups[Citation38,Citation39]. After the surface-modification of the SRs by APTES and TA, the modified SRs showed the peaks at 1745 and 1593 cm−1, which confirmed the presence of C═O and N−H groups in TA and APTES, respectively[Citation40,Citation41]. The BRI@SR and BRI@SR@PDA showed the similar characteristic peaks with BRI at 1482 cm−1 (due to the C−C groups), and 3216 and 1593 cm−1 (attributed to the N−H groups)[Citation42]. Compared to the BRI@SR, BRI@SR@PDA showed slight absorption fluctuation at approximately 3300 cm−1, which might be indicative of stretching vibration of −OH group due to dopamine polymerization[Citation43,Citation44].
Figure 3. (A) FTIR spectra of TA, BRI, APTES, and SR before and after modification, BRI-loading and PDA coating. Associated characteristic peaks have been indicated by dotted lines. (B-C) Thermogravimetric (TG) analysis, (D) differential scanning calorimetry (DSC) analysis and (E) stress-strain tensile curves of the modified SR, BRI@SR, BRI@SR@PDA and BRI@SR@PT
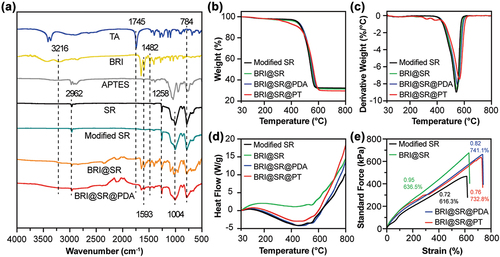
Conjunctival sac inserts should possess good thermal stability at 37 ºC. Thus, we evaluated the thermal properties of the modified SR, BRI@SR, BRI@SR@PDA and BRI@SR@PT within 30 - 800 ºC by the TG-DSC analyzer. As shown in , the TG and DTG curves showed that four kinds of samples had the fastest weight loss rate (7.5 - 9.0%/min) at about 550 ºC. It was worth noting that the BRI@SR@PT began to lose weight from 326 ºC, potentially attributed to the TPU thermal degradation. Moreover, the rapid composition change and the fastest weight loss of four kinds of samples were also confirmed by the DSC curves () in which the exothermic peaks were observed around 550 ºC corresponding to those presented in the previous TG/DTG curves[Citation25]. Furthermore, the mechanical properties of the insert were also determined through a tensile test, and the results were shown in . The interaction between BRI and TA grafted in the modified SR likely contributed to the observed reduction in elasticity, as indicated by the increased slope (0.95) of the BRI@SR compared to the SR (0.72). After PDA and TPU coating, the curve slopes decreased a little to 0.82 (BRI@SR@PDA) and 0.76 (BRI@SR@PT), respectively. BRI-loading into the modified SR made its elastic strain increase from 616.3% to 636.5% (BRI@SR). The elastic strain further increased to 741.1% (BRI@SR@PDA) after the PDA-coating and then slightly decreased to 732.8% (BRI@SR@PT) after the TPU-coating. Collectively, the BRI@SR@PT presented great thermal stability and excellent elasticity which could be administrated in rabbit conjunctival sac for IOP-lowering treatment.
3.2. In vitro cytocompatibility of BRI@SR@PT
The cytocompatibility of the inserts was examined by culturing the HCECs and MICECs with the leaching liquors of BRI@SR@PDA and BRI@SR@PT, and detecting the cell viability by CCK-8 analysis and Live/Dead cell staining assay. As shown in , compared to the control group, the BRI@SR@PDA and BRI@SR@PT demonstrated no observable toxicity on HCECs and MICECs, as evidenced by the lack of red fluorescence (dead cells) in the Calcein-AM/PI staining. Statistical analysis revealed no significant differences in relative cell viability on HCECs and MICECs exposed to the leaching liquor of the inserts and the control group (p > 0.05). After incubating with the leaching liquors for 24 h, the relative cell viability of HCECs and MICECs reached 98.1% and 98.7% respectively, compared to 99.6% and 101.5% for BRI@SR@PDA (). These results confirmed the good in vitro cytocompatibility of BRI@SR@PT.
Figure 4. In vitro cytocompatibility of BRI@SR@PDA and BRI@SR@PT. (A) Fluorescence images of HCECs and MICECs after 24 h incubation with the leaching liquors of BRI@SR@PDA or BRI@SR@PT. Calcein-AM/PI staining photos showed dead cells as red and live cells as green. (B) Histogram of cell viability of the above treated HCECs and MICECs.
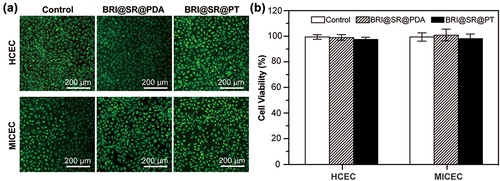
3.3. In vitro drug release from BRI@SR@PT
The average mass of the modified SR was 108.9 ± 2.4 mg, and the loaded BRI in one BRI@SR@PT was 853.0 ± 81.2 μg. As shown in , a decreased BRI Cmax (39.4 μg/mL at 1 h) was observed in the BRI@SR@PDA compared to that in the BRI@SR (Cmax: 58.3 μg/mL at 1 h). The drug release profile revealed that the BRI@SR@PT exhibited a further improved inhibition on initial burst release, and even no obvious burst release was observed. Compared with the previously reported DDSs with TPU or PDMS multilayer coating[Citation24,Citation25], the BRI@SR@PT with a layer of PDA/TPU coating was able to provide a preferable inhibition on BRI burst release. In the whole observation term (1 - 28 d), the BRI@SR@PT provided the most stable drug release and improved the immediate BRI concentration compared with the BRI@SR and BRI@SR@PDA (). As far as the BRI cumulative release curves showed in , the BRI cumulative release from the BRI@SR was approaching 100% (complete drug release) at day 8, while those in the BRI@SR@PT and BRI@SR@PDA increased steadily to 81.0% and 70.4% until day 28, respectively. It was worth explaining that the TPU coating on BRI@SR@PDA further inhibited the initial BRI burst release and simultaneously enhanced the following BRI sustained release from the BRI@SR@PT. These results suggested that the BRI@SR@PT showed the most effective release profile, which made it the top choice for the subsequent investigations.
3.4. In vivo drug release from BRI@SR@PT
After administration of the BRI@SR@PT or BRI eye drops into rabbit eyes, the BRI concentration in the aqueous humor was measured by UPLC-MS/MS to evaluate the in vivo drug release, and the results were shown in . The BRI eye drop group exhibited a fast clearance rate, reflected by a sharp decline in BRI concentration in the aqueous humor within 24 h, whereas that in the BRI@SR@PT group declined slowly up to 192 h (8 d). At 1 h, the BRI concentration in the BRI eye drop group was about 2.7 times of that in the BRI@SR@PT group (2415.0 ng/mL vs. 884.8 ng/mL, p < 0.001), and then dropped to a similar level at 4 h (395.1 ng/mL vs. 358.6 ng/mL). At 24 h, no enough BRI was detected in the aqueous humor in the BRI eye drop group, while a considerable BRI concentration (116.5 ng/mL) was still maintained in the BRI@SR@PT group. The BRI@SR@PT exhibited a sustained-release profile, reaching about 9.2 ng/mL of BRI at day 8, which was far higher than 2.9 ng/mL, the EC50 of BRI (concentration for 50% of the maximal effect) as α2-adrenergic agonist[Citation45]. The improved in vivo drug release is expected to significantly prolong BRI action time and ameliorate treatment effect on glaucoma. It should be noted that the above-mentioned BRI concentrations in the BRI eye drop group were in good agreement with those in the previous study[Citation24,Citation25,Citation46], which proved that the BRI detection method used in this study was reasonable and believable.
Figure 6. In vivo BRI concentration in rabbit aqueous humor and IOP-lowering effects after administration of the BRI@SR@PT. or BRI eye drops (0.15% w/w). (A, B) The curves and histograms of BRI concentration in aqueous humor (four rabbits survived to the final observation point and n = 4). (C, D) The short-term and long-term IOP curves (n = 5) during 28 d. Plots with * or # represent statistically significant differences compared to that in the control group (no any operation) or BRI eye drop group.
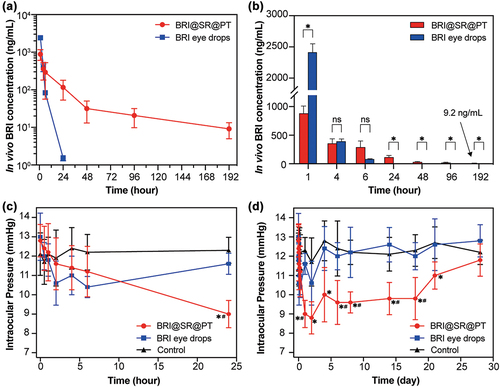
Regarding ocular drug delivery, it is inevitable that part of the drugs dissolved in tears are absorbed by conjunctival vessels and enter systemic circulation[Citation47]. In order to obtain enough BRI concentration in rabbit aqueous humor and sustained treatment effect on glaucoma, we simply calculated the purpose BRI-loading dose (> 315 µg) in one BRI@SR@PT, according to the EC50 of BRI (2.9 ng/mL) in aqueous humor[Citation45], drug delivery efficiency by trans-corneal permeation, and drug loss by trans-conjunctival permeation into systemic circulation[Citation47]. Then, we ameliorated the internal microstructure of the SRs by NaOH treatment and TA grafting, and improved the drug-loading course by repeatedly immersing the modified SRs in the BRI solution with high concentration to make BRI loaded as much as possible. As a result, the BRI-loading dose in one BRI@SR@PT was 853.0 ± 81.2 μg, which was in agreement with the designed drug-loading dose. Thus, the calculation of BRI-loading dose in one BRI@SR@PT was reasonable, and the practical drug-loading method was very successful. In order to improve the drug release profile, we further made a PDA/TPU coating on the BRI@SR to inhibit the initial BRI burst release and enhance the following BRI sustained release. In this course, TPU-coating significantly improved BRI release, and PDA obviously enhanced the stability of the BRI@SR@PT by increasing the adhesion of the BRI@SR and TPU-coating, which was supported by the previous reports[Citation25,Citation27]. As we all know, the maximum volume of aqueous humor in a rabbit eye is around 300 µL, and the production and outflow rates of aqueous humor are about 3 µL/min so as to maintain a balanced IOP[Citation48]. When 100 μL of aqueous humor was withdrawn from a rabbit eye, theoretically the deficiency of aqueous humor could rapidly be supplied in tens of minutes. The extracted drugs in the withdrawn aqueous humor were negligible to the total drugs delivered into anterior chamber because plenty of drugs were expelled away with the continuous outflow of aqueous humor in the normal ocular circulation. In this study, the long-term BRI concentrations in aqueous humor in the BRI eye drop group were not obtained due to the limit of detection. In addition, the drug concentrations in tears should be investigated in future to adequately understand the in vivo BRI release from BRI@SR@PT. By a sustained drug delivery style, the BRI@SR@PT significantly increased intraocular drug absorption and inhibited systemic absorption when compared with the BRI eye drops, which was consistent with the previous report[Citation49]. The obtained BRI@SR@PT could sustainedly release BRI for 28 d and might meet the IOP-lowering treatment need.
3.5. In vivo IOP-lowering efficacy of BRI@SR@PT
The effectiveness of BRI@SR@PT in lowering IOP was investigated, and the results were shown in . Topical application of BRI eye drops resulted in a rapid IOP reduction within 2 - 6 h (10.4 ~ 11.0 mmHg), which then returned to the normal level after 24 h and up to day 28. However, the BRI@SR@PT exhibited a slow but steadily decreasing IOP, and the IOP ultimately reached a minimum of 8.8 mmHg at day 2. Then the IOP fluctuated at a significantly low level (8.8 ~ 10.0 mmHg) till day 18. The IOP level in the BRI@SR@PT group was significantly lower than those in the other two groups. From day 18, IOP in the BRI@SR@PT group increased gradually, and ultimately reached the baseline IOP at day 28 (11.7 ~ 12.8 mmHg). It was worth mentioning that the BRI@SR@PT group still presented obviously lower IOP than the control group at day 21, which showed that the effective treatment period of the BRI@SR@PT was generally thought as 21 d ().
To investigate the in vivo drug release from the BRI@SR@PT and IOP-lowering effectiveness, we designed two experiments based on the different batches of rabbits. In the first batch of rabbits, we collected rabbits’ aqueous humor and detected the BRI concentrations. In the second batch of rabbits, we tested the rabbits’ IOP. It was noted that the collection of rabbits’ aqueous humor and the IOP measurements had to be separated because the extraction of aqueous humor might affect the accuracy of IOP measurements. In this study, we administered the eye drops multiple times initially in order to compare the efficacy and durability of IOP-lowering treatment between the BRI eye drops and BRI@SR@PT. Indeed, daily administration of BRI eye drops is more clinically realistic for patients. In subsequent experiments, we will further investigate the advantages of the BRI@SR@PT in terms of drug usage, treatment effect and tissue toxicity by daily administration of BRI eye drops.
3.6. In vivo biosafety of BRI@SR@PT
To investigate the biosafety of the BRI@SR@PT, we observed the anterior segments of rabbit eyes with a slit lamp and examined the H&E-stained eyeball tissue sections after administration of the insert or BRI eye drops. The photos of eyeball tissue in the BRI@SR@PT group shown in were similar to those in the BRI eye drop group and the control group. No obvious inflammatory reactions, such as conjunctival congestion, corneal epithelial lesions, corneal edema or secretions, were observed within 28 d, which indicated that the BRI@SR@PT had no irritation effect on rabbit eyes. Furthermore, H&E staining images of eyeball tissues at day 28 showed no pathological changes in all three groups (). No inflammatory cell infiltration or structural disorder was observed in the cornea, conjunctiva or retina tissues, which indicated great biosafety of the BRI@SR@PT.
Figure 7. (A) Representative photographs of the anterior segments of rabbit eyes in the control, BRI eye drops (0.15% w/w) and BRI@SR@PT groups. Three kinds of slit lamp modes including diffuse illumination, direct focal illumination and retro-illumination were used for the examination of the anterior segments of rabbit eyes. The BRI@SR@PT. in the conjunctival sacs of rabbit eyes were marked with black arrows. (B) Representative H&E staining images of cornea, conjunctiva and retina in three groups. CnE, corneal epithelial layer; CjE, conjunctival epithelial layer; S, stroma; EN, endothelial layer; LP, lamina propria; GCL, ganglion cell layer; INL, inner nuclear layer; ONL, outer nuclear layer.
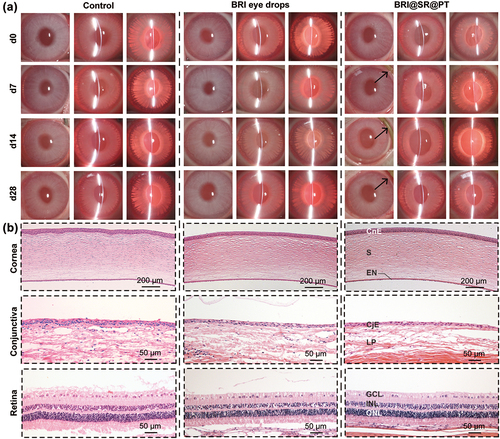
4. Conclusion
In this study, a new conjunctival sac insert (BRI@SR@PT) was designed and developed for sustainable BRI release and effective IOP reduction in glaucoma treatment. The BRI@SR@PT provided an improved inhibition effect on BRI burst release and a sustained BRI release for up to 28 d. The insert had excellent biocompatibility in vitro and in vivo. After administrated in the conjunctival sac of rabbit eyes, the BRI@SR@PT provided a consistent IOP-lowering effect up to 21 d with great biosafety, when compared with BRI eye drops. Based on the excellent properties and sustained drug release, the BRI@SR@PT presented a superior application prospect in future for the treatment of ocular hypertension and glaucoma.
Declaration of Interests
The authors have no relevant affiliations or financial involvement with any organization or entity with a financial interest in or financial conflict with the subject matter or materials discussed in the manuscript. This includes employment, consultancies, honoraria, stock ownership or options, expert testimony, grants or patents received or pending, or royalties.
Reviewer Disclosures
Peer reviewers on this manuscript have no relevant financial or other relationships to disclose.
Author contributions
Z Zhang, Q Ma and Z Dai: acquisition and analysis of data for the work, drafting the work; J Hong, J Gu, R Shi and J Xu: design of the work, interpretation of data, reviewing the work; Y Ma, X Sun and J Sun: conception and design of the work, reviewing the work, supervision and resources. All authors reviewed the work, and agreed to be accountable for all aspects of the work.
Additional information
Funding
REFERENCES
- Tham YC, Li X, Wong TY, et al. Global prevalence of glaucoma and projections of glaucoma burden through 2040: a systematic review and meta-analysis. Ophthalmology. 2014;121(11):2081–2090. doi: 10.1016/j.ophtha.2014.05.013
- Stein JD, Khawaja AP, Weizer JS. Glaucoma in adults-screening, diagnosis, and management: A review. JAMA. 2021;325(2):164–174.
- Kingman S. Glaucoma is second leading cause of blindness globally. Bull World Health Organ. 2004;82(11):887–888.
- Jonas JB, Aung T, Bourne RR, et al.. Glaucoma. The Lancet. 2017;390(10108):2183–2193. doi: 10.1016/S0140-6736(17)31469-1
- Weinreb RN, Aung T, Medeiros FA. The pathophysiology and treatment of glaucoma: a review. JAMA. 2014;311(18):1901–1911. doi: 10.1001/jama.2014.3192
- Schuster AK, Erb C, Hoffmann EM, et al. The diagnosis and treatment of glaucoma. Dtsch Arztebl Int. 2020;117(13):225–234. doi: 10.3238/arztebl.2020.0225
- Oh DJ, Chen JL, Vajaranant TS, et al. Brimonidine tartrate for the treatment of glaucoma. Expert Opin Pharmacother. 2019;20(1):115–122. doi: 10.1080/14656566.2018.1544241
- Theodore K, Jeffrey ML, David SG, et al. A randomized trial of brimonidine versus timolol in preserving visual function: results from the Low-Pressure Glaucoma Treatment Study. Am J Ophthalmol. 2011;151(4):671–681.
- Robin A, Grover DS. Compliance and adherence in glaucoma management. Indian J Ophthalmol. 2011;59(1):93–96.
- Kompella UB, Hartman RR, Patil MA Extraocular, periocular, and intraocular routes for sustained drug delivery for glaucoma. Prog Retin Eye Res. 2021;82:100901. 10.1016/j.preteyeres.2020.100901
- Mohan N, Chakrabarti A, Nazm N, et al. Newer advances in medical management of glaucoma. Indian J Ophthalmol. 2022;70(6):1920–1930. doi: 10.4103/ijo.IJO_2239_21
- Brandt JD, DuBiner HB, Benza R, et al. Long-term safety and efficacy of a sustained-release bimatoprost ocular ring. Ophthalmology. 2017;124(10):1565–1566. doi: 10.1016/j.ophtha.2017.04.022
- Lewis RA, Christie WC, Day DG, et al. Bimatoprost sustained-release implants for glaucoma therapy: 6-month results from a Phase I/II clinical trial. Am J Ophthalmol. 2017;175:137–147. 10.1016/j.ajo.2016.11.020
- Di Prima G, Licciardi M, Carfì Pavia F, et al. Microfibrillar polymeric ocular inserts for triamcinolone acetonide delivery. Int J Pharm. 2019;567:118459. 10.1016/j.ijpharm.2019.118459
- Zhao JY, Xiong J, Ning Y, et al. A triple crosslinked micelle-hydrogel lacrimal implant for localized and prolonged therapy of glaucoma. Eur J Pharm Biopharm. 2023;185:44–54. 10.1016/j.ejpb.2023.02.011
- Vigani B, Rossi S, Sandri G, et al. Recent advances in the development of in situ gelling drug delivery systems for non-parenteral administration routes. Pharmaceutics. 2020;12(9):859. doi: 10.3390/pharmaceutics12090859
- Yang H, Tyagi P, Kadam RS, et al. Hybrid dendrimer hydrogel/PLGA nanoparticle platform sustains drug delivery for one week and antiglaucoma effects for four days following one-time topical administration. ACS Nano. 2012;6(9):7595–7606. doi: 10.1021/nn301873v
- Sun J, Lei Y, Dai Z, et al. Sustained release of brimonidine from a new composite drug delivery system for treatment of glaucoma. ACS Appl Mater Interfaces. 2017;9(9):7990–7999. doi: 10.1021/acsami.6b16509
- Zhai Z, Cheng Y, Hong J Nanomedicines for the treatment of glaucoma: Current status and future perspectives. Acta Biomater. 2021;125:41–56. 10.1016/j.actbio.2021.02.017
- Schnichels S, Hurst J, de Vries JW, et al. Improved treatment options for glaucoma with brimonidine-loaded lipid DNA nanoparticles. ACS Appl Mater Interfaces. 2021;13(8):9445–9456. doi: 10.1021/acsami.0c18626
- El-Salamouni NS, Farid RM, El-Kamel AH, et al. Nanostructured lipid carriers for intraocular brimonidine localisation: development, in-vitro and in-vivo evaluation. J Microencapsul. 2018;35(1):102–113. doi: 10.1080/02652048.2018.1425753
- Xu Y, Li H. In vitro and in vivo evaluation of brimonidine loaded silica nanoparticles-laden silicone contact lenses to manage glaucoma. J Biomater Appl. 2022;37(2):333–343. doi: 10.1177/08853282221090880
- Craven ER, Walters T, Christie WC, et al. 24-month phase I/II clinical trial of bimatoprost sustained-release implant (bimatoprost SR) in glaucoma patients. Drugs. 2020;80(2):167–179. doi: 10.1007/s40265-019-01248-0
- Huang C, Shen Y, Zhao Y, et al. Sustained release of brimonidine from polydimethylsiloxane-coating silicone rubber implant to reduce intraocular pressure in glaucoma. Regen Biomater. 2023;10:rbad041. 10.1093/rb/rbad041
- Zhao Y, Huang C, Zhang Z, et al. Sustained release of brimonidine from BRI@SR@TPU implant for treatment of glaucoma. Drug Deliv. 2022;29(1):613–623. doi: 10.1080/10717544.2022.2039806
- Lee H, Dellatore SM, Miller WM, et al. Mussel-inspired surface chemistry for multifunctional coatings. Science. 2007;318(5849):426–430. doi: 10.1126/science.1147241
- Palladino P, Bettazzi F, Scarano S. Polydopamine: surface coating, molecular imprinting, and electrochemistry-successful applications and future perspectives in (bio)analysis. Anal Bioanal Chem. 2019;411(19):4327–4338.
- Alfieri ML, Weil T, Ng DYW, et al. Polydopamine at biological interfaces. Adv Colloid Interface. 2022;305:102689.
- Sun Y, Li Y, Zhang Y, et al. A polydopamine-assisted strontium-substituted apatite coating for titanium promotes osteogenesis and angiogenesis via FAK/MAPK and PI3K/AKT signaling pathways. Mater Sci Eng C-Mater. 2021;131:112482.
- Sileika TS, Kim HD, Maniak P, et al. Antibacterial performance of polydopamine-modified polymer surfaces containing passive and active components. ACS Appl Mater Interfaces. 2011;3(12):4602–4610. doi: 10.1021/am200978h
- Ma T, Wang CX, Ge XY, et al. Applications of polydopamine in implant surface modification. Macromol Biosci. 2023;23(10):e2300067. doi: 10.1002/mabi.202300067
- Liu SH, Zhao X, Tang JM, et al. Drug-eluting hydrophilic coating modification of intraocular lens via facile dopamine self-polymerization for posterior capsular opacification prevention. ACS Biomater Sci Eng. 2021;7(3):1065–1073.
- Fang QA, Qin C, Duo L, et al. Polydopamine based photothermal/photodynamic synchronous coating modified intraocular lens for efficient and safer posterior capsule opacification prevention. Biomater Adv. 2024;158:213792.
- He X, Obeng E, Sun X, et al. Polydopamine, harness of the antibacterial potentials-A review. Materials Today Bio. 2022;15:100329.10.1016/j.mtbio.2022.100329
- Liu Y, Ai K, Lu L. Polydopamine and its derivative materials: synthesis and promising applications in energy, environmental, and biomedical fields. Chem Rev. 2014;114(9):5057–5115. doi: 10.1021/cr400407a
- Hong SH, Hong S, Ryou MH, et al. Sprayable ultrafast polydopamine surface modifications. Adv Mater Interfaces. 2016;3(11):1500857.
- Ma L, Feng X, Liang H, et al. A novel photothermally controlled multifunctional scaffold for clinical treatment of osteosarcoma and tissue regeneration. Mater Today. 2020;36:48–62.
- Sreejith KJ, Prabhakaran PV, Laly KP, et al. Vinyl-functionalized poly(borosiloxane) as precursor for SiC/SiBOC nanocomposite. Ceram Int. 2016;42(14):15285–15293.
- Zhao J, Jiang N, Zhang D, et al.. Study on optimization of damping performance and damping temperature range of silicone rubber by polyborosiloxane gel. Polymers (Basel). 2020;12(5):1196. doi: 10.3390/polym12051196
- Liu W, Fei M, Ban Y, et al. Concurrent improvements in crosslinking degree and interfacial adhesion of hemp fibers reinforced acrylated epoxidized soybean oil composites. Compos Sci Technol. 2018;160:60–68.
- Chen Y, Duan Q, Zhu J, et al. Anchor and bridge functions of APTES layer on interface between hydrophilic starch films and hydrophobic soyabean oil coating. Carbohydr Polym. 2021;272:118450. 10.1016/j.carbpol.2021.118450
- Salim SA, Badawi NM, El-Moslamy SH, et al. Novel long-acting brimonidine tartrate loaded-PCL/PVP nanofibers for versatile biomedical applications: fabrication, characterization and antimicrobial evaluation. RSC Adv. 2023;13(22):14943–14957.
- Liu M, Liu T, Chen X, et al.. Nano-silver-incorporated biomimetic polydopamine coating on a thermoplastic polyurethane porous nanocomposite as an efficient antibacterial wound dressing. J Nanobiotechnol. 2018;16(1):89. doi: 10.1186/s12951-018-0416-4
- Zangmeister RA, Morris TA, Tarlov MJ. Characterization of polydopamine thin films deposited at short times by autoxidation of dopamine. Langmuir. 2013;29(27):8619–8628. doi: 10.1021/la400587j
- Acheampong AA, Shackleton M, Tangliu DD. Comparative ocular pharmacokinetics of brimonidine after a single dose application to the eyes of albino and pigmented rabbits. Drug Metab Dispos. 1995;23(7):708–712.
- Acheampong AA, Shackleton M, John B, et al. Distribution of brimonidine into anterior and posterior tissues of monkey, rabbit, and rat eyes. Drug Metab Dispos. 2002;30(4):421–429. doi: 10.1124/dmd.30.4.421
- Ramsay E, del Amo EM, Toropainen E, et al. Corneal and conjunctival drug permeability: Systematic comparison and pharmacokinetic impact in the eye. Eur J Pharm Sci. 2018;119:83–89. 10.1016/j.ejps.2018.03.034
- del Amo EM, Hammid A, Tausch M, et al. Ocular metabolism and distribution of drugs in the rabbit eye: Quantitative assessment after intracameral and intravitreal administrations. Int J Pharm. 2022;613:121361.
- Pang XC, Li JW, Pi JX, et al. Increasing efficacy and reducing systemic absorption of brimonidine tartrate ophthalmic gels in rabbits. Pharm Dev Technol. 2018;23(3):231–239. doi: 10.1080/10837450.2017.1328693