ABSTRACT
Introduction: Pharmacogenomics has great potential in reducing drug-induced severe cutaneous adverse drug reactions (SCARs). Pharmacogenomic studies have revealed an association between HLA genes and SCARs including acute generalized exanthematous pustulosis (AGEP), drug reaction with eosinophilia and systemic symptoms (DRESS), Stevens-Johnson syndrome (SJS), and toxic epidermal necrolysis (TEN).
Areas covered: Pharmacogenomics-guided therapy could prevent severe drug hypersensitivity reactions. The US Food and Drug Administration (FDA), Clinical Pharmacogenetics Implementation Consortium (CPIC), and Dutch Pharmacogenetics Working Group (DPWG) provided guidelines in the translation of clinically relevant and evidence-based SCARs pharmacogenomics research into clinical practice. In this review, we intended to summarize the significant HLA alleles associated with SCARs induced by different drugs in different populations. We also summarize the SCARs associated with genetic and non-genetic factors and the cost-effectiveness of screening tests.
Expert opinion: The effectiveness of HLA screening on a wider scale in clinical practice requires significant resources, including state-of-the-art laboratory; multidisciplinary team approach and health care provider education and engagement; clinical decision support alert system via electronic medical record (EMR); laboratory standards and quality assurance; evidence of cost-effectiveness; and cost of pharmacogenomics tests and reimbursement.
1. Introduction
Severe cutaneous adverse drug reactions (SCARs) are one of the commonest medical challenges with high fatality and are considered to be a multifactorial drug side effect. The common SCARs seen in clinical practice include acute generalized exanthematous pustulosis (AGEP), drug reaction with eosinophilia and systemic symptoms (DRESS), Stevens-Johnson syndrome (SJS), and toxic epidermal necrolysis (TEN). The most common medications associated with SCARs are non-steroidal anti-inflammatory drugs (NSAIDs), antibiotics, antiepileptics, allopurinol, and antiretroviral drugs [Citation1]. AGEP consists of numerous non-follicular sterile pustules in the epidermis, fever, and peripheral-blood leukocytosis of neutrophils (neutrophilia, neutrophil leukocytosis) [Citation2,Citation3]. SJS and TEN are characterized by widespread keratinocyte death resulting in extensive epidermal loss with mucous membrane erosions. SJS and TEN are considered as severity variants of the same drug-induced disease classified by the degree of detachment over the total body surface area; less than 10% is considered SJS, 10–30% is considered as SJS-TEN overlap, and over 30% is considered as TEN. DRESS is characterized by cutaneous exanthema, fever, hematologic abnormalities (eosinophilia or atypical lymphocytes), and internal organ involvement [Citation2].
Studies have revealed the interaction between the highly polymorphic human leukocyte antigen (HLA) and drugs, and HLA genotypes have been implicated in explaining the variability and unpredictability of SCARs presentations among different individuals. A large number of studies have reported the association of the HLA alleles with AGEP, DRESS, and SJS/TEN across different populations [Citation3–5]. This review aims to provide an association between SCARs and HLA alleles in different populations. This review also provides an overview of the pathogenesis of SCARs, pathomechanisms in HLA associated drug-induced SCARs, clinical pharmacogenetics implementation guidelines and recommendations, and pharmacoeconomic perspective of HLA genotyping before drug prescription.
2. Adverse drug reactions (ADRs) associated with immunological reactions
ADRs are classified into type A and type B reactions. Type A reactions are nonimmune-mediated and are due to the pharmacological activity of the drug. Type B reactions are immune-mediated and independent of pharmacologic activity [Citation6]. The immune-mediated ADRs include urticaria, DRESS, AGEP, fixed drug eruption, SJS/TEN, drug-induced liver injury (DILI), thrombocytopenia, agranulocytosis, aplastic anemia, and drug-induced lupus [Citation7].
The knowledge of SCARs epidemiology is essential to establish timely and appropriate genetic screening measures for selected patient groups and drug classes. The prevalence of AGEP is estimated to be between 0.35–5/million [Citation8]. The incidence of AGEP is estimated between 1 and 5 cases per million inhabitants per year. For DRESS, population-based studies in Japan reported an incidence of 10 per million person-years [Citation9]. Among the patients treated with aromatic antiepileptic drugs, the risk of SJS/TEN is 1/10,000 to 1/1,000 and the risk of DRESS is 1 to 9 per 10,000. The risk of DRESS is higher among patients treated with lamotrigine (1 per 300 adults and 1 per 100 children exposed) [Citation4]. The mortality rates have been reported up to 10% in DRESS [Citation8]. On average, the mortality rate for SJS is between 1% and 5%, with global mortality rates up to 25–35% in patients with TEN.
Drugs may induce SCARs due to the CD8+ and CD4+ T-cells immune responses. SJS/TEN are caused by granulysin-producing cytotoxic CD8+ T cells, natural killer (NK) cells, and natural killer T (NKT) cells. Also, both CD4+ and CD8+ effector T-cells are activated in patients with DRESS [Citation6]. The pathogenesis of AGEP involves activation, proliferation, and migration of drug-specific CD4+ and CD8+ T-cells to the skin [Citation10].
The histology of AGEP shows typical subcorneal or intraepidermal spongiform or non-spongiform pustules with or without dermal edema, focal necrotic keratinocytes, neutrophil infiltrates sometimes with eosinophils, and mild vasculitis. SJS and TEN cases show histological features of massive epidermal necrosis (including all layers of the epidermis), focal adnexal necrosis, necrotic keratinocytes, mild mononuclear cell dermal infiltrate, and negative direct immunofluorescence test. In DRESS, the histological findings are described as nonspecific lichenoid or eczematous lesions, focal necrotic keratinocytes, dense and diffuse dermal-epidermal infiltrates with lymphocytic exocytosis, dermal edema, and superficial perivascular infiltrate of mostly lymphocytes with or without eosinophils [Citation11–13].
Drug hypersensitivity reactions (DHRs) were a strong association between HLA, drug or metabolite form, peptide and T-cell receptor (TCR). Drugs may induce an immune response as demonstrated by (1) Model 1: altered repertoire model proposes the altered self-peptides then leads to aTCR and induced the manifestations of immune system and caused by binding mechanism of non-covalent between drugs and HLA molecules (2) Model 2: hapten model is a small molecular of chemical or drug and presented as a stable hapten peptide complex and able to bind covalently to HLA which contribute to stimulate an immune response (3) Model 3: pro-hapten model is a covalent binding process of drugs metabolites and HLA molecule and stimulate the immune system and (4) Model 4: pharmacological interaction (p-i) model is an interact directly with immunologic receptors by non-covalent bonds, the mechanism of this theory include of drug bind to the HLA or TCR of T lymphocyte (CD8+) to elicit immune system [Citation3]. shows the mechanisms of these reactions contributing to a greater understanding of T-cell-mediated drug hypersensitivity.
Figure 1. Mechanisms of drug hypersensitivity models with drug binding to HLA and TCR: Model 1; altered repertoire model proposes the altered self-peptides then leads to a TCR and induced the manifestations of immune system and caused by binding mechanism of non-covalent between drugs and HLA molecules, Model 2; hapten model is a small molecular of chemical or drug and presented as a stable hapten peptide complex and able to bind covalently to HLA which contribute to stimulate an immune response, Model 3; pro-hapten model is a covalent binding process of drugs metabolites and HLA molecule and stimulate the immune system and Model 4; pharmacological interaction (p-i) model is an interact directly with immunologic receptors by non-covalent bonds, the mechanism of this theory include of drug bind to the HLA or TCR of T lymphocyte (CD8+) to elicit immune system
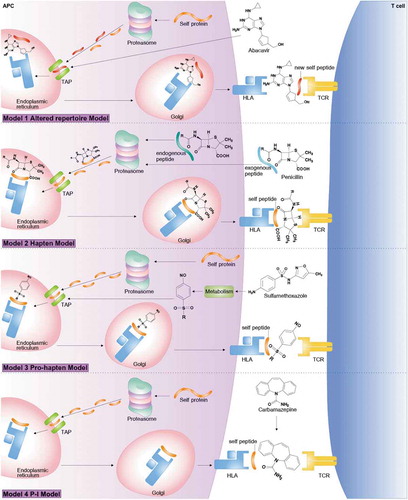
3. Genetic susceptibility to SCARs
During pathological conditions of SCARs, HLAs present antigens to the TCR and then elicit specific T cell-dependent immune responses [Citation3]. The occurrence of drug-induced SCARs is strongly associated with specific HLA alleles. These specific HLA-mediated SCARs are highly specific; hence, HLA alleles that are linked to SCARs represent biomarkers for the pharmacogenomics-guided therapy in a patient. The most important examples of this association include HLA-B*57:01 (abacavir), HLA-B*15:02/HLA-A*31:01 (carbamazepine), and HLA-B*58:01 (allopurinol) in specific ethnicities [Citation14]. The explanation of the genetic basis of SCARs may lead to an understanding of their pathogenesis and genetic screening for the risk genetic variants results in the prevention of SCARs. It has been shown that the genetic variant of the drug metabolism enzyme also plays a role in the pathogenesis of SCARs [Citation15].
3.1. HLA alleles associated with SCARs
HLA is located on the chromosome 6, including of HLA class I, HLA class II and HLA class III. Variations at HLA class I (HLA-A, HLA-B, and HLA-C) and class II loci (HLA-DR, HLA-DQ, and HLA-DP) are associated with drug-induced SCARs. Pharmacogenomic markers in the HLA coding genes in different ethnicities are summarized in .
Table 1. The significant HLA alleles associated with SCARs induced by different drugs in different populations
The association of HLA-B*57:01 and abacavir hypersensitivity has been reported in different ethnicities, including Australian, African American, Brazilian, British, Hispanic, African, Iranian, and Indian [Citation16,Citation17]. HLA-B*57:01 was strongly associated with abacavir hypersensitivity in Western Australian HIV-1-positive individuals treated with abacavir [Citation18]. Screening for HLA-B*57:01 for patients before initiation of abacavir therapy has been recommended by the US Food and Drug Administration (FDA), Clinical Pharmacogenetics Implementation Consortium (CPIC), and Dutch Pharmacogenetics Working Group (DPWG) abacavir to reduce the risk of a hypersensitivity reaction [Citation19,Citation20].
The HLA-B*15:02 allele is strongly associated with carbamazepine-induced SJS/TEN in Han Chinese, Thai, Malay, Indians, Vietnam populations [Citation21–27]. The HLA-A*31:01 allele is associated with carbamazepine-induced SJS/TEN, and AGEP in Caucasian, Japanese, Korean, Chinese, and patients of mixed origin [Citation28]. A study conducted among the Europeans showed that HLA-A*31:01 was associated with carbamazepine-induced MPE/DRESS, and HLA‐B*57:01 was associated with carbamazepine-induced SJS/TEN [Citation29]. Furthermore, a meta-analysis investigating the association of HLA-B alleles and carbamazepine-induced SJS/TEN demonstrated HLA-B*15:11 as a risk factor in the development of carbamazepine-induced SJS/TEN in the Asian population [Citation30]. The US FDA recommends the genetic screening of HLA-B*15:02 for all patients with ancestry in populations with increased frequency of HLA-B*15:02, before initiation of carbamazepine therapy [Citation31]. The HLA-B*15:02 allele is also strongly associated with phenytoin-induced SJS/TEN in Han Chinese [Citation32]. Other associations include the alleles HLA-B*13:01, HLA-C*08:01, and HLA-DRB1*16:02 in phenytoin-induced SJS/TEN in Han Chinese [Citation33]. The HLA-B*51:01 and HLA-C*14:02 alleles have been linked with phenytoin-induced DRESS in Thai children [Citation34]. The authors also showed that Thai children carrying HLA-B*38:02 were at high risk for phenytoin-induced SJS/TEN. Phenytoin-induced DRESS and SJS/TEN have been associated with HLA-B*15:13 in Malays [Citation35]. The association between the HLA-B*15:02 and oxcarbazepine-induced SJS has been reported in Han Chinese [Citation33].
The variant HLA-B*58:01 allele is strongly associated with allopurinol-induced SCARs. The HLA-B*58:01 allele has been linked to allopurinol-induced SJS/TEN and/or DRESS in Han Chinese, Thai, Japanese, European, and Korean [Citation36–40]. Li et al. reported a strong association between HLA-A*33:03 and HLA-C*03:02 alleles and allopurinol-induced SJS/TEN in Asian populations [Citation41]. The CPIC recommends that allopurinol should not be used in patients who are positive for the HLA-B*58:01 allele [Citation42].
An association between HLA-B*13:01 and dapsone-induced DRESS and SJS/TEN has been reported in Asian populations [Citation43,Citation44]. It was reported that HLA-B*13:01 was a predictor of the dapsone-induced HSS in Chinese [Citation45], as well as to DRESS and SJS/TEN in Thais [Citation46]. Co-trimoxazole-induced induced SJS/TEN has been strongly associated with HLA-B*15:02, HLA-C*06:02, and HLA-C*08:01 alleles in the Thai population [Citation47,Citation48]. Also, the HLA-B*13:01 allele has been associated with DRESS induced by co-trimoxazole in Thais [Citation48]. In a multicountry case-control association study involving Taiwan, Thailand, and Malaysia, the HLA-B*13:01 allele showed a strong association with co-trimoxazole-induced SJS/TEN and DRESS [Citation49]. Vancomycin is associated with a risk of AGEP, DRESS, and SJS/TEN [Citation50]. Konvinse et al. reported a strong association between HLA-A*32:01 and vancomycin-induced DRESS in Europeans [Citation51]. Cold medicines-related SJS/TEN has been strongly associated with HLA-A*02:06 and HLA-B*44:03 alleles in the Japanese population [Citation52]. HLA-B*59:01 was strongly linked with methazolamide-induced SJS/TEN in Japanese, Korean and Han Chinese [Citation53,Citation54]. HLA-C*04:01 was reported as a risk of SJS/TEN from Nevirapine in Malawian [Citation55,Citation56].
3.2. Other gene variants for SCARs
CYP2C9 variants have been associated with phenytoin-induced SCARs, and CPIC recommends consideration of at least a 25% reduction in the starting maintenance dose for patients who are CYP2C9 intermediate metabolizers and a 50% reduction for CYP2C9 poor metabolizers [Citation57,Citation58]. CYP2C9*3 allele has been reported as a marker for phenytoin-induced DRESS in Thais [Citation59–62]. A study by Chung et al. showed that CYP2C9*3 was strongly associated with phenytoin-induced SCARs in Taiwan, Japan, and Malaysia [Citation15]. The association between CYP2B6 polymorphisms and nevirapine-induced SJS/TEN was first reported in HIV-1 patients from Mozambique. In the study, the CYP2B6 T983C polymorphism was found to be significantly associated with a higher risk to develop SJS/TEN with an OR of 4.2 [Citation63].
4. Non-genetic factors for SCARs
A study by Yampayon et al. reported that omeprazole co-medication was strongly associated with phenytoin-induced DRESS in Thai patients [Citation59]. A study by Zhao et al. showed that patients taking multiple-drug combinations and with a complex medication history, which greatly contributed to the development of SCARs [Citation64]. The use of multiple drugs results in the accumulation of drugs, reactive metabolites, and drug interaction because of excessive demands on enzyme systems for oxidation or acetylation of multiple drugs [Citation65]. Reactivation of human herpesvirus (HHV)-6 has been reported to be associated with DRESS and SJS/TEN [Citation66,Citation67]. Also, Epstein–Barr virus (EBV) is associated with SJS [Citation68]. Mycoplasma pneumoniae have been implicated in the development of atypical SJS [Citation69]. Among the host factors, the presence of systemic lupus erythematosus, tuberculosis, and HIV have been found to increase the risk of SCARs. Drugs that are associated with a higher risk of SCARs include aromatic anti-epileptic agents, allopurinol, antimicrobial agents (co-trimoxazole, vancomycin, aminopenicillin, minocycline, sulfasalazine, and dapsone), and NSAIDs (celecoxib and ibuprofen) [Citation8]. Incidences of SCARs including SJS, TEN, and DRESS have been reported after immunotherapy owing to the reactivation of T-cells [Citation70,Citation71].
5. Current understanding of pathomechanisms in HLA associated drug-induced SCARs
The HLA molecule contributes to the pathogenesis of the drug-induced SCARs. The HLA molecules bind to specific drug antigens and contribute to drug-induced SCARs by presenting the drug antigens to specific TCR which activate T-cell-mediated immune responses [Citation72]. In carbamazepine-induced SJS/TEN, carbamazepine can directly interact with HLA-B*15:02 protein and the endogenous peptide-loaded HLA-B*15:02 molecules present carbamazepine to cytotoxic lymphocytes (CTLs) that leads to the apoptosis of keratinocytes resulting from the degranulation of perforin and granzyme B and Fas/FasL interaction [Citation73]. In allopurinol-induced SCARs, Yun et al. explained that allopurinol and its metabolite, oxypurinol, bind to the HLA-B*58:01 molecule in the F pocket of the peptide-binding groove and activate drug-specific T cells [Citation74].
6. Databases with HLA alleles frequency
The Allele Frequency Net Database (AFND, www.allelefrequencies.net) provides frequency data on the polymorphisms of HLA, killer-cell immunoglobulin-like receptors (KIR), major histocompatibility complex class I chain-related genes (MIC), and several cytokine gene polymorphisms in worldwide populations [Citation75]. The allele frequencies data on HLA in one or many populations can be explored using the query tool HLA Allele Freq Search (http://allelefrequencies.net/hla6006a.asp) in AFND. An additional tool for exploring the HLA haplotype frequencies and HLA rare allele in a set of populations at two or more loci is available in AFND (http://allelefrequencies.net/hla6003a.asp). Also, a database for HLA adverse drug reactions (HLA-ADR, http://allelefrequencies.net/hla-adr/) has been added to the AFND. HLA-ADR is a database for the analysis of HLA and adverse drug reactions and covers 46 drugs/drug classes.
7. DHRs in Southeast Asia: regional issues and challenges
DHRs represent a significant public health problem with a prevalence of 10-20% in hospitalized patients and up to 7% in the general population exposed to drugs [Citation76]. Tang et al. surveyed the diagnostic modalities used in the evaluation and management of DHRs among member countries of the Asia Pacific Association of Allergy, Asthma and Clinical Immunology (APAAACI) which included respondents from five Southeast Asian countries [Citation77]. The authors reported that HLA genotype testing before prescribing carbamazepine, allopurinol, and abacavir was not mandatory or recommended by local pharmacy regulatory agencies in the majority of countries despite strong associations for SCARs with Asian ethnicity. In Southeast Asia, a wide knowledge gap, accurate diagnosis, and management of DHRs are the major challenge of healthcare practitioners (HCPs). There is a lack of guidelines and/or consensus documents to support the clinical management of DHRs in Southeast Asian countries. There are not collaborative research networks regarding DHRs in the Southeast Asian region. Multi-country clinical networks need to be established to include more patients with the same reaction to the same drug in the same clinical context to conclude the pathophysiology of drug allergy. There is also a gap in establishing a multidisciplinary group to facilitate the investigators, integrate basic and clinical studies, and establish guidelines for the diagnosis and management of drug allergy within South East Asia.
8. Clinical pharmacogenetics implementation guidelines and recommendations
Several pharmacogenomics guidelines and recommendations are available from international committees with expertise in the field of pharmacogenomics. However, the recommendations from these guidelines vary due to the differences in the drafting standards for these guidelines [Citation78].
CPIC guidelines suggest testing for HLA-B*15:02 and HLA-A*31:01 alleles, which are associated with SCARs among patients taking certain aromatic anticonvulsants, in populations with increased frequency of HLA-B*15:02 and HLA-A*31:01 alleles. The CPIC recommendations include avoiding the use of carbamazepine and oxcarbazepine and selecting an alternative drug if a patient is carbamazepine-naïve or oxcarbazepine-naïve and HLA-B*15:02 positive. For carbamazepine-naïve individuals testing positive for HLA-A*31:01 allele, carbamazepine should be avoided and an alternative therapy must be chosen [Citation79]. As recommended by the DPWG guidelines, carbamazepine should not be used for individuals positive for HLA-B*15:02, HLA-A*31:01, and HLA-B*15:11 alleles and, if possible, choose an alternative drug. The Canadian Pharmacogenomics Network for Drug Safety (CPNDS) strongly recommends genetic testing for HLA-B*15:02 for carbamazepine-naïve patients originating from populations where HLA-B*15:02 is common, its frequency unknown, or whose origin is unknown before initiation of carbamazepine therapy. Genetic testing for HLA-B*15:02 is optional in patients originating from populations where HLA-B*15:02 is rare. The CPNDS has also recommends genetic testing for the HLA-A*31:01 allele for all carbamazepine-naïve patients with moderate-risk ethnic backgrounds before initiation of carbamazepine therapy [Citation31]. CPIC, DPWG, and CPNDS provide similar therapeutic guidelines regarding genetic testing for HLA-B*15:02 before initiating carbamazepine therapy in the risk population.
The CPIC recommends that allopurinol should not be prescribed to allopurinol-naïve individuals who are HLA-B*58:01-positive, and an alternative drug should be considered for these patients to avoid the risk of developing SCARs [Citation42]. The American College of Rheumatology (ACR) also recommends HLA-B*58:01 screening in sub-populations where both the HLA-B*58:01 allele frequency is elevated and the HLA-B*58:01-positive subjects are at high risk for severe allopurinol hypersensitivity reaction [Citation80]. Both CPIC and ACR recommend testing all allopurinol-naïve patients for HLA-B*58:01 in the population with a high frequency of this allele.
The CPIC guidelines provide information for the interpretation of HLA-B and/or CYP2C9 genotype tests so that the results can guide the dosing and/or use of phenytoin. The CPIC recommends avoiding phenytoin (or its prodrug fosphenytoin) for HLA-B*15:02-positive patients regardless of CYP2C9 genotype, patient ancestry, or age. Also, the US FDA label recommends that consideration should be given to avoiding phenytoin as an alternative for carbamazepine in patients positive for HLA-B*1502 (https://www.accessdata.fda.gov/drugsatfda_docs/label/2011/010151s036lbl.pdf).
9. The pharmacoeconomic perspective of HLA genotyping before drug prescription
HLA genotyping has been recommended before drug prescription so that those positive for the risk allele are not given the drug associated with the SCARs. The successful translation of HLA-guided treatment strategies depends on the cost-effectiveness of routinely assessing HLA markers before initiating drug therapy [Citation81]. An economic evaluation assesses the costs and outcomes of health care interventions that may improve health care and health outcomes [Citation82]. Several studies have conducted pharmacoeconomic analyses of HLA-based approaches.
Choi et al. reported the HLA-B*15:02 screening strategy to be more cost-effective than a no screening strategy before carbamazepine therapy in epilepsy patients of Asian ancestry in the United States [Citation83]. In Thailand, HLA-B*15:02 screening was found to be a cost-effective intervention for preventing carbamazepine-induced SJS/TEN on patients with neuropathic pain, but not on patients with epilepsy [Citation84]. This finding was further supported by Tiamkao et al. by studies in Thai patients and recommended specific HLA-B*15:02 screening for carbamazepine () for implementation in the national policy [Citation85]. Among the Singaporean Chinese and Malays, Dong et al. found the screening of HLA-B*15:02 allele and providing alternate antiepileptic drugs to be more cost-effective compared with no screening of HLA-B*15:02 allele and providing carbamazepine to all [Citation86]. On the contrary, HLA-B*15:02 screening is unlikely to be cost-effective in an ethnically diverse Malaysian population and Singaporean Indians [Citation86,Citation87]. Plumpton et al. examined the total costs and quality-adjusted life-years (QALYs) by genotype-guided dosing (with lamotrigine prescribed for patients who test positive for HLA-A*31:01 allele) versus standard care of carbamazepine prescription without testing in UK populations [Citation88]. The study found that routine screening for the HLA-A*31:01 allele before initiation of carbamazepine for epilepsy likely to represent a cost-effective intervention in the UK population.
Figure 2. Pharmacogenomic card of pharmacogenetics testing for specific drug-biomarker (positive/negative result)
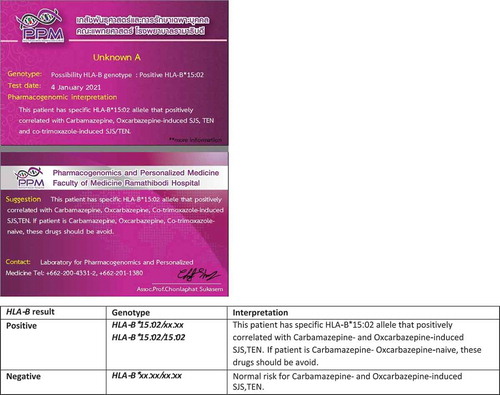
Jutkowitz et al. evaluated the cost-effectiveness of HLA-B*58:01 testing before the initiation of allopurinol in gout patients according to race/ethnicity in the US [Citation89]. The analysis found that HLA-B*58:01 testing is cost-effective for Asians, African Americans, but not for Caucasians or Hispanics. Saokaew et al. conducted the cost-effectiveness of HLA-B*58:01 testing before initiating allopurinol therapy compared with usual care (no genetic testing) in Thailand [Citation90]. The results revealed the genetic testing of HLA-B*58:01 before allopurinol therapy as a highly potential cost-effective intervention in Thailand. Contrary to the findings of Jutkowitz et al. and Saokaew et al., Chong et al. suggested that HLA-B*58:01 screening before allopurinol pharmacotherapy is unlikely to be a cost-effective intervention in Malaysia [Citation91]. With the advancement in genomic technologies and a reduction in the price of genomic testing, the pharmacogenomics-guided treatment strategy will be more cost-effective and HLA screening will be a core clinical service. HLA typing by next generation sequencing (NGS) can sequence entire HLA gene to identify novel risk loci for drug toxicities [Citation92].
10. Clinical implementation of genetic screening to prevent SCARs
Several studies have established the association of genetic variants encoding drug-metabolizing enzymes and HLA with drug-induced SCARs. Pharmacogenomics testing before the prescription of drugs responsible for SCARs has the potential to prevents SCARs and save money by improving the cost-effectiveness of health care delivery. The HLA pharmacogenomics tests have high predictive values for drug associated SCARs in several populations [Citation93]. A preemptive and multiplexed approach is suggested over-reactive and one-gene-per-test approach for addressing the disadvantages of reactive genotyping for drug-specific variants when a pharmacogenomics drug is prescribed because the genetic data can be available at the time of prescribing the culprit drugs for SCARs, genotypes for multiple pharmacogenes per test are available before prescribing related drug pharmacogenomics, data can be used over long periods, and the incremental cost to obtain multiple genetic variant genotypes is small [Citation94]. The Vanderbilt University Medical Center (VUMC) has implemented a program of preemptive genotyping, the Pharmacogenomic Resource for Enhanced Decisions in Care and Treatment (PREDICT), which uses clinical data available in the electronic medical record to calculate the risk of being prescribed a risk medication for ADRs. In PREDICT, the prescriber receives an alert that the patient may benefit from (multiplexed) genetic testing. The panel of variants is then genotyped and the selected genotypes are then released into the medical record of patients to guide clinicians who are prescribing medications [Citation95]. The clinical implementation of screening for the HLA risk alleles that represent the strongest predictor of drug-induced SCARs is successfully applied in clinical practice among the populations with a higher frequency of the risk alleles before initiation of the risk drugs. For instance, HLA-B*57:01 for abacavir, HLA-B*15:02 for carbamazepine, oxcarbazepine, and phenytoin, HLA-B*58:01 for allopurinol, HLA-B*13:01 for dapsone [Citation3,Citation96].
11. National policy for HLA screening to prevent SCARs: Thailand model
The incidence of drug-induced SJS/TEN is reported to be 10.35 per 1,000 persons while the mortality rate is reported to be 7–50% in the Thai population [Citation97]. Screening for HLA-B*15:02 is mandated in Thai patients before initiation of carbamazepine therapy to prevent carbamazepine-induced SJS/TEN (). The Thai universal health coverage scheme reimburses 28 USD per person for the HLA-B*15:02 testing [Citation98]. Currently, in Thailand, several pharmacogenomics tests are performed for the prevention of drug-induced SCARs (). These include HLA-A*31:01 for carbamazepine-induced hypersensitivity, HLA-B*57:01 for abacavir-induced drug hypersensitivity, HLA-B*13:01 for dapsone-induced SCARs, and HLA-B*58:01 for allopurinol-induced SCARs () [Citation99]. Unlike HLA-B*15:02, pharmacogenomics screening is not currently mandated for HLA-A*31:01 before initiation of carbamazepine therapy in Thailand.
12. Future direction for research and implementation in SCARs
Multiple genetic variations are responsible for the development of drug-induced SCARs. The development of a diagnostic marker for drug-induced SCARs is important for the early detection, prevention, and treatment of drug-induced SCARs patients. With the advent of next-generation sequencing, genome sequencing must be established in both clinical and research settings to prevent drug-induced SCARs [Citation100]. The genomic database containing both the genotype and the specific phenotype data for a population or ethnic group should be developed to support a clinical interpretation of genomic test results [Citation101]. Also, a big-data approach must be implemented to consolidate several current resources into larger, more dynamic, and collaborative knowledge bases in the pharmacogenomics of SCARs. Future research should aim to advance our understanding of the genetic basis of drug-induced SCARs and use that knowledge to reduce SCARs cases. A preemptive pharmacogenomics panel testing approach must be implemented to personalize therapy with high-risk drugs [Citation102].
Further research is required to evaluate the cost-effectiveness of pharmacogenomics-guided therapy. More research is required to identify genetic determinants of drug-induced SCARs to help reveal pathogenic mechanisms of drug-induced SCARs.
13. Clinical implementation of HLA genotyping for prevention of SCARs in Thailand
Several genetic variants of genes encoding drug-metabolizing enzymes (DMEs) and HLAs have been identified as the risk factors for SCARs, including SJS, TEN, maculopapular exanthema (MPE), and DRESS. Thailand has taken significant footsteps in the field of pharmacogenomics, interpreting genetic test results, and applying genetic information to drug therapy decisions in larger hospitals Several public and private hospitals offer pharmacogenetic screening for the HLA genotyping before prescription such as Ramathibodi Hospital (University Hospital) and Bumrungrad International Hospital (Private Hospital). The health insurance system in Thailand has three major schemes for the entire population, which are the Civil Servant Medical Benefit Scheme (CSMBS), the Social Security Scheme (SSS), and the Universal Coverage (UC) scheme [Citation103]. The private health insurance schemes exist in Thailand, providing coverage to the pharmacogenomics services depending on the health benefits package.
The UC scheme, operated by the National Health Security Office (NHSO), has the coverage for HLA-B*15:02 screening with a reimbursement of 28 USD per person in Thailand [Citation98]. HLA-B*15:02 testing is mandatory in Taiwan and Hong Kong and is highly recommended as standard of care in Singapore before initiation of carbamazepine therapy [Citation104,Citation105]. The reimbursement for the HLA-B*58:01 screening test is likely to include within the UC by 2021 in Thailand. Saokaew et al. reported that routine testing for HLA-B*58:01 in patients being prescribed allopurinol for gout is highly cost-effective in Thailand [Citation90]. HLA-B*58:01 testing is recommended for Southeast Asian and African-American descent individuals to reduce the occurrence of allopurinol-induced SCARs and related deaths [Citation106]. Moreover, HLA-B*13:01 for co-trimoxazole and dapsone [Citation46,Citation48,Citation107,Citation108] should be considered include in the UC and strongly recommended before starting prescription.
To effectively implement pharmacogenomics services across the country, some steps need to be followed to ensure that the appropriate evidence and resources are in place to facilitate the successful implementation of pharmacogenomics services. A genetic variant predictive of drug response must be identified for the first time during clinical studies. The clinical validity of the genetic variant must be replicated in an independent cohort of the observed genotype-dependent outcome. Cost-effectiveness analysis, another important characteristic influencing clinical translation, must be assessed to compare the costs of pharmacogenomics screening and health gains. After the genetic screening is proven to be cost-effective, a peer-reviewed guideline for the translation of pharmacogenomics test results is formulated to make clinical decisions. Finally, policymakers capture the potential of pharmacogenomics to meet public health goals through the integration of pharmacogenomics into public health policy [Citation109].
14. Conclusions and future perspectives
In this review, we intended to summarize the significant HLA alleles associated with SCARs induced by different drugs in different populations. Both genetic and non-genetic factors are involved in drug-induced SCARs. Considering all the evidence favoring HLA screening, it is recommended to implement routine screening for the HLA risk alleles to prevent drug-induced SCARs in the populations with a high frequency of risk HLA alleles such as Thai and South East Asian [Citation99,Citation110]. The frequencies of each HLA allele in different populations be found in the public database. We also reviewed the economic evaluations and clinical implementation of pharmacogenomics-based therapy in preventing drug-induced SCARs. In the future, the cost-effectiveness evaluation needs to be conducted for the HLA pharmacogenomics-guided treatment, allowing a wider clinical application and supporting the decision-makers and other stakeholders to make implementation decisions.
15. Expert opinion
Establishing the HLA risk alleles screening in clinical pharmacogenomics setting requires state – of-the -art laboratory and the multidisciplinary team with highly qualified personnel to perform and delivery high-quality genetic testing. The pharmacogenomics workflow must be arrangement and efficient through the process of handing over of the genotyping results to the patients. The collaboration between physicians and pharmacist is also a key to the successful implementation of pharmacogenomics in clinical settings. Pharmacists should inform and assist physicians and patients in the use and interpretation of pharmacogenomics information. Counselors should provide support and education to the patients that explain what genotyping is and why it was done to facilitate informed decision-making ().
Figure 6. The pharmacogenomics workflow must be arrangement and efficient through the process of handing over of the genotyping results to the patients
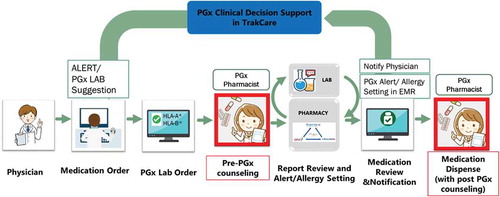
Clinical decision support (CDS) alert system is essential to overcome the barriers associated with the generation and utilization of pharmacogenomics guidelines into clinical practice. The pharmacogenomics CDS should be integrated with electronic medical record (EMR) to deliver synchronous interventions
(i.e. pop-up alert in the order entry system advising the clinician to order a pharmacogenomics screening test based on a drug order or a pop-up alert prompted by a specific drug–gene interaction) and asynchronous interventions (i.e. inbox message or e-mail when new pharmacogenomics test results are available). HLA screening results must be incorporated into EMR and CDS to aid clinicians in the prescribing process ().
Figure 7. The pharmacogenomics clinical decision support (CDS) should be integrated with EMR to deliver synchronous interventions as an example in Bumrungrad International Hospital, Bangkok, Thailand. The pop -up alert in the order entry system advising the clinician to order a pharmacogenomics screening test based on a drug order or pop -up alert prompted by a specific drug -gene interaction (7a). The HLA screening results must be incorporated into electronic medical record (EMR) and CDS to support clinicians in the prescribing process (7b)
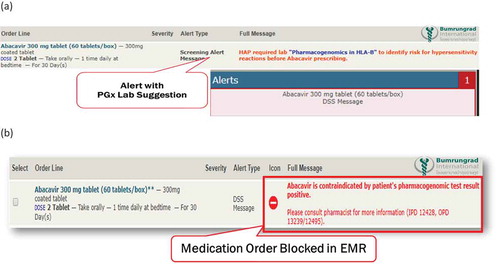
Insurance companies are reluctant to reimburse pharmacogenomics tests due to a lack of evidence. Evidence-based guidelines can be compiled from the FDA labeling and CPIC guidelines for pharmacogenomics informed therapeutic recommendations. When the routine screening for genetic variants in pharmacogenes associated with prescription drugs is found to be cost-effective, health insurance companies are likely to reimburse routine pharmacogenomics testing.
The successful implementation of pharmacogenomics-based medicine in routine clinical practice will maximize drug efficacy and minimize adverse drug reactions, and decrease the overall cost of health care with the reduction in adverse drug reactions. An increasingly more affordable pharmacogenomics testing, combined with the high risk predicted by HLA alleles, allows us to hope that in the near future, the SCARs might be prevented and eradiated by HLA screening approach. A standardized guideline does not exist, and the approach must likely to be varied for different setting, however HLA-B genotyping e.g. HLA-B*1502 (carbamazepine), HLA-B*5701(abacavir), HLA-B*5801 (allopurinol) and HLA-B*13:01 (co-trimoxazole and dapsone) is strongly recommended before starting prescription. The effective of HLA screening on a wider scale in clinical practice requires significant resources, including state-of-the-art laboratory; multidisciplinary team approach and health care provider education and engagement; clinical decision support alert system via EMR; laboratory standards and quality assurance; evidence of cost-effectiveness; and cost of pharmacogenomics tests and reimbursement.
Article highlights
Considering all the evidence favoring HLA screening, it is recommended to implement routine screening for the HLA risk alleles to prevent drug-induced SCARs in the populations with a high frequency of risk HLA alleles.
HLA-B genotyping e.g. HLA-B*15:02 (carbamazepine), HLA-B*57:01 (abacavir), HLA-B*58:01 (allopurinol) and HLA-B*13:01 (co-trimoxazole and dapsone) is strongly recommended before starting prescription.
Both genetic and non-genetic factors should be considered for prevention of drug-induced SCARs.
The cost-effectiveness evaluation needs to be conducted for the HLA pharmacogenomics-guided treatment, allowing a wider clinical application and supporting the decision-makers and other stakeholders to make implementation decisions.
A preemptive pharmacogenomics panel testing approach must be implemented to personalize therapy with high-risk drugs.
Establishing the HLA risk alleles screening in clinical pharmacogenomics setting requires state-of-the-art laboratory and the multidisciplinary team with highly qualified personnel to perform and deliver high-quality genetic testing.
Reviewer disclosures
Peer reviewers on this manuscript have no relevant financial or other relationships to disclose.
Declaration of interest
The authors have no relevant affiliations or financial involvement with any organization or entity with a financial interest in or financial conflict with the subject matter or materials discussed in the manuscript. This includes employment, consultancies, honoraria, stock ownership or options, expert testimony, grants or patents received or pending, or royalties.
Acknowledgments
The authors thank the staff of the Pharmacogenomics and Personalized Medicine laboratory, Ramathibodi Hospital, Pharmacogenomics Team, Bumrungrad International Hospital. The authors also wish to thank Dr. Montinee Sangtian for her support and suggestion. The authors would like to acknowledge and thank Mr. Samart Jamrat for drawing and graphic design of the figures.
Additional information
Funding
References
- Oshikoya KA, Ogunyinka IA, Ogar CK, et al. Severe cutaneous adverse drug reactions manifesting as Stevens-Johnson syndrome and toxic epidermal necrolysis reported to the national pharmacovigilance center in Nigeria: a database review from 2004 to 2017. Ther Adv Drug Saf. 2020;11:2042098620905998.
- Marzano AV, Borghi A, Cugno M. Adverse drug reactions and organ damage: the skin. Eur J Intern Med. 2016;28:17–24.
- Chang CJ, Chen C-B, Hung S-I, et al. Pharmacogenetic testing for prevention of severe cutaneous adverse drug reactions. Front Pharmacol. 2020;11:969.
- Ramirez E, Bellón T, Tong HY, et al. Significant HLA class I type associations with aromatic antiepileptic drug (AED)-induced SJS/TEN are different from those found for the same AED-induced DRESS in the Spanish population. Pharmacol Res. 2017;115:168–178.
- Phillips EJ, Chung W-H, Mockenhaupt M, et al. Drug hypersensitivity: pharmacogenetics and clinical syndromes. J Allergy Clin Immunol. 2011;127(3 Suppl):S60–6.
- Karnes JH, Miller MA, White KD, et al. Applications of immunopharmacogenomics: predicting, preventing, and understanding immune-mediated adverse drug reactions. Annu Rev Pharmacol Toxicol. 2019;59(1):463–486.
- Uetrecht J. Immune-mediated adverse drug reactions. Chem Res Toxicol. 2009;22(1):24–34.
- Mustafa SS, Ostrov D, Yerly D. Severe Cutaneous adverse drug reactions: presentation, risk factors, and management. Curr Allergy Asthma Rep. 2018;18(4):26.
- Hoetzenecker W, Nägeli M, Mehra ET, et al. Adverse cutaneous drug eruptions: current understanding. Semin Immunopathol. 2016;38(1):75–86.
- Feldmeyer L, Heidemeyer K, Yawalkar N. Acute generalized Exanthematous Pustulosis: pathogenesis, genetic background, clinical variants and therapy. Int J Mol Sci. 2016;17(8):1214.
- Duong TA, Valeyrie-Allanore L, Wolkenstein P, et al. Severe cutaneous adverse reactions to drugs. Lancet. 2017;390(10106):1996–2011.
- Lerch M, Mainetti C, Terziroli Beretta-Piccoli B, et al. Current perspectives on Stevens-Johnson syndrome and toxic epidermal necrolysis. Clin Rev Allergy Immunol. 2018;54(1):147–176.
- Orime M. Immunohistopathological findings of severe cutaneous adverse drug reactions. J Immunol Res. 2017;2017:6928363.
- Jung JW, Kim J-Y, Park I-W, et al. Genetic markers of severe cutaneous adverse reactions. Korean J Intern Med. 2018;33(5):867–875.
- *Chung WH, Chang W-C, Lee Y-S, et al., Genetic variants associated with phenytoin-related severe cutaneous adverse reactions. JAMA. 312(5): 525–534. 2014.
- Fan WL, Shiao M-S, Hui RC-Y, et al. HLA association with Drug-Induced adverse reactions. J Immunol Res. 2017;2017:3186328.
- Sousa-Pinto B, Pinto-Ramos J, Correia C, et al. Pharmacogenetics of abacavir hypersensitivity: a systematic review and meta-analysis of the association with HLA-B*57:01. J Allergy Clin Immunol. 2015;136(4):1092–4 e3.
- Mallal S, Nolan D, Witt C, et al. Association between presence of HLA-B*5701, HLA-DR7, and HLA-DQ3 and hypersensitivity to HIV-1 reverse-transcriptase inhibitor abacavir. Lancet. 2002;359(9308):727–732.
- Martin MA, Hoffman JM, Freimuth RR, et al. Clinical pharmacogenetics implementation consortium guidelines for HLA-B Genotype and Abacavir dosing: 2014 update. Clin Pharmacol Ther. 2014;95(5):499–500.
- Manson LEN, Swen JJ, Guchelaar HJ. Diagnostic test criteria for HLA genotyping to prevent drug hypersensitivity reactions: a systematic review of actionable HLA recommendations in CPIC and DPWG guidelines. Front Pharmacol. 2020;11:567048.
- Wang Q, Zhou J-Q, Zhou L-M, et al. Association between HLA-B*1502 allele and carbamazepine-induced severe cutaneous adverse reactions in Han people of southern China mainland. Seizure. 2011;20(6):446–448.
- Shi YW, Min F-L, Qin B, et al. Association between HLA and Stevens-Johnson syndrome induced by carbamazepine in Southern Han Chinese: genetic markers besides B*1502? Basic Clin Pharmacol Toxicol. 2012;111(1):58–64.
- *Sukasem C, Chaichan C, Nakkrut T, et al. Association between HLA-B alleles and carbamazepine-induced maculopapular exanthema and severe cutaneous reactions in Thai patients. J Immunol Res. 2018;2018:2780272.
- *Chung WH, Hung S-I, Hong H-S, et al., Medical genetics: a marker for Stevens-Johnson syndrome. Nature. 428(6982): 486. 2004.
- Lim KS, Kwan P, Tan CT. Association of HLA-B*1502 allele and carbamazepine-induced severe adverse cutaneous drug reaction among Asians, a review. Neurol Asia. 2008;13:15–21.
- Mehta TY, Prajapati L, Mittal B, et al. Association of HLA-BFNx011502 allele and carbamazepine-induced Stevens-Johnson syndrome among Indians. Indian J Dermatol Venereol Leprol. 2009;75(6):579–582.
- Nguyen DV, Chu HC, Nguyen DV, et al. HLA-B*1502 and carbamazepine-induced severe cutaneous adverse drug reactions in Vietnamese. Asia Pac Allergy. 2015;5(2):68–77.
- Amstutz U, Shear NH, Rieder MJ, et al. Recommendations for HLA-B*15:02 and HLA-A*31:01 genetic testing to reduce the risk of carbamazepine-induced hypersensitivity reactions. Epilepsia. 2014;55(4):496–506.
- Mockenhaupt M, Wang C-W, Hung S-I, et al. HLA-B*57:01 confers genetic susceptibility to carbamazepine-induced SJS/TEN in Europeans. Allergy. 2019;74(11):2227–2230.
- Wang Q, Sun S, Xie M, et al. Association between the HLA-B alleles and carbamazepine-induced SJS/TEN: a meta-analysis. Epilepsy Res. 2017;135:19–28.
- Dean L. Carbamazepine therapy and HLA genotype, in medical genetics summaries. Pratt VM., Scott SA., Pirmohamed M., et al., Editors. Bethesda (MD): National Center for Biotechnology Information (US); 2012.
- Cheung YK, Cheng S-H, Chan EJM, et al. HLA-B alleles associated with severe cutaneous reactions to antiepileptic drugs in Han Chinese. Epilepsia. 2013;54(7):1307–1314.
- Hung SI, Chung W-H, Liu Z-S, et al. Common risk allele in aromatic antiepileptic-drug induced Stevens-Johnson syndrome and toxic epidermal necrolysis in Han Chinese. Pharmacogenomics. 2010;11(3):349–356.
- Manuyakorn W, Likkasittipan P, Wattanapokayakit S, et al. Association of HLA genotypes with phenytoin induced severe cutaneous adverse drug reactions in Thai children. Epilepsy Res. 2020;162:106321.
- Chang CC, Ng -C-C, Too C-L, et al. Association of HLA-B*15:13 and HLA-B*15:02 with phenytoin-induced severe cutaneous adverse reactions in a Malay population. Pharmacogenomics J. 2017;17(2):170–173.
- Hung SI, Chung W-H, Liou L-B, et al. HLA-B*5801 allele as a genetic marker for severe cutaneous adverse reactions caused by allopurinol. Proc Natl Acad Sci U S A. 2005;102(11):4134–4139.
- *Sukasem C., Jantararoungtong T., Kuntawong P., et al. HLA-B*58:01 for Allopurinol-Induced Cutaneous Adverse Drug Reactions: implication for Clinical Interpretation in Thailand. Front Pharmacol. 2016;7:186.
- Kaniwa N., Saito Y., Aihara M., et al. HLA-B locus in Japanese patients with anti-epileptics and allopurinol-related Stevens-Johnson syndrome and toxic epidermal necrolysis. Pharmacogenomics. 2008;9(11):1617–1622.
- Stamp LK, Day RO, Yun J. Allopurinol hypersensitivity: investigating the cause and minimizing the risk. Nat Rev Rheumatol. 2016;12(4):235–242.
- Kang HR, Jee YK, Kim Y-S, et al. Positive and negative associations of HLA class I alleles with allopurinol-induced SCARs in Koreans. Pharmacogenet Genomics. 2011;21(5):303–307.
- Li X, Zhao Z, Sun SS. Association of human leukocyte antigen variants and allopurinol-induced Stevens-Johnson syndrome and toxic epidermal necrolysis: a meta-analysis. Am J Health Syst Pharm. 2017;74(9):e183–e192.
- Hershfield MS, Callaghan JT, Tassaneeyakul W, et al. Clinical Pharmacogenetics Implementation Consortium guidelines for human leukocyte antigen-B genotype and allopurinol dosing. Clin Pharmacol Ther. 2013;93(2):153–158.
- Tangamornsuksan W, Lohitnavy M. Association Between HLA-B*1301 and Dapsone-Induced Cutaneous adverse drug reactions: a systematic review and Meta-analysis. JAMA Dermatol. 2018;154(4):441–446.
- Park HJ, Park J-W, Kim SH, et al. The HLA-B*13:01 and the dapsone hypersensitivity syndrome in Korean and Asian populations: genotype- and meta-analyses. Expert Opin Drug Saf. 2020;19(10):1349–1356.
- Zhang FR, Liu H, Irwanto A, et al. HLA-B*13:01 and the Dapsone hypersensitivity syndrome. N Engl J Med. 2013;369(17):1620–1628.
- Tempark T, Satapornpong P, Rerknimitr P, et al. Dapsone-induced severe cutaneous adverse drug reactions are strongly linked with HLA-B*13: 01 allele in the Thai population. Pharmacogenet Genomics. 2017;27(12):429–437.
- Kongpan T, Mahasirimongkol S, Konyoung P, et al. Candidate HLA genes for prediction of co-trimoxazole-induced severe cutaneous reactions. Pharmacogenet Genomics. 2015;25(8):402–411.
- *Sukasem C, Pratoomwun J, Satapornpong P, et al., Genetic Association of Co-Trimoxazole-Induced Severe cutaneous adverse reactions is Phenotype-Specific: HLA Class I genotypes and haplotypes. Clin Pharmacol Ther. 108(5): 1078–1089. 2020.
- Wang CW., Tassaneeyakul W., Chen CB., et al. Whole genome sequencing identifies genetic variants associated with co-trimoxazole hypersensitivity in Asians. J Allergy Clin Immunol. 2020;147 (4): 1402-1412.
- De Luca JF, Holmes NE, Trubiano JA. Adverse reactions to vancomycin and cross-reactivity with other antibiotics. Curr Opin Allergy Clin Immunol. 2020;20(4):352–361.
- Konvinse KC, Trubiano JA, Pavlos R, et al. HLA-A*32:01 is strongly associated with vancomycin-induced drug reaction with eosinophilia and systemic symptoms. J Allergy Clin Immunol. 2019;144(1):183–192.
- Ueta M, Kaniwa N, Sotozono C, et al. Independent strong association of HLA-A*02:06 and HLA-B*44:03 with cold medicine-related Stevens-Johnson syndrome with severe mucosal involvement. Sci Rep. 2014;4(1):4862.
- Kim SH, Kim M, Lee KW, et al. HLA-B*5901 is strongly associated with methazolamide-induced Stevens–Johnson syndrome/toxic epidermal necrolysis. Pharmacogenomics. 2010;11(6):879–884.
- Yang F, Xuan J, Chen J, et al. HLA-B*59:01: a marker for Stevens-Johnson syndrome/toxic epidermal necrolysis caused by methazolamide in Han Chinese. Pharmacogenomics J. 2016;16(1):83–87.
- Carr DF, Chaponda M, Jorgensen AL, et al. Association of human leukocyte antigen alleles and nevirapine hypersensitivity in a Malawian HIV-infected population. Clin Infect Dis. 2013;56(9):1330–1339.
- Carr DF, Bourgeois S, Chaponda M, et al. Genome-wide association study of nevirapine hypersensitivity in a sub-Saharan African HIV-infected population. J Antimicrob Chemother. 2017;72(4):1152–1162.
- Dean L, Kane M. Phenytoin Therapy and HLA-B*15:02 and CYP2C9 genotypes, in medical genetics summaries. Pratt VM., Scott SA., Pirmohamed M., et al., Editors. Bethesda (MD): National Center for Biotechnology information (US); 2012
- Fohner AE, Rettie AE, Thai KK, et al. Associations of CYP2C9 and CYP2C19 Pharmacogenetic variation with phenytoin-Induced cutaneous adverse drug reactions. Clin Transl Sci. 2020;13(5):1004–1009.
- Yampayon K, Sukasem C, Limwongse C, et al. Influence of genetic and non-genetic factors on phenytoin-induced severe cutaneous adverse drug reactions. Eur J Clin Pharmacol. 2017;73(7):855–865.
- Suvichapanich S, Jittikoon J, Wichukchinda N, et al. Association analysis of CYP2C9*3 and phenytoin-induced severe cutaneous adverse reactions (SCARs) in Thai epilepsy children. J Hum Genet. 2015;60(8):413–417.
- Sukasem C, Sririttha S, Tempark T, et al. Genetic and clinical risk factors associated with phenytoin-induced cutaneous adverse drug reactions in Thai population. Pharmacoepidemiol Drug Saf. 2020;29(5):565–574.
- Su SC, Chen C-B, Chang W-C, et al. HLA Alleles and CYP 2C9*3 as predictors of phenytoin hypersensitivity in East Asians. Clin Pharmacol Ther. 2019;105(2):476–485.
- Ciccacci C, Di Fusco D, Marazzi MC, et al. Association between CYP2B6 polymorphisms and Nevirapine-induced SJS/TEN: a pharmacogenetics study. Eur J Clin Pharmacol. 2013;69(11):1909–1916.
- Zhao J, Hu L, Zhang L, et al. Causative drugs for drug-induced cutaneous reactions in central China: a 608-case analysis. An Bras Dermatol. 2019;94(6):664–670.
- Gomes ESR, Marques ML, Regateiro FS. Epidemiology and risk factors for severe delayed drug hypersensitivity reactions. Curr Pharm Des. 2019;25(36):3799–3812.
- Chen YC, Chiang -H-H, Cho Y-T, et al. Human herpes virus reactivations and dynamic cytokine profiles in patients with cutaneous adverse drug reactions --a prospective comparative study. Allergy. 2015;70(5):568–575.
- Teraki Y, Murota H, Izaki S. Toxic epidermal necrolysis due to zonisamide associated with reactivation of human herpesvirus 6. Arch Dermatol. 2008;144(2):232–235.
- Ishida T, Kano Y, Mizukawa Y, et al. The dynamics of herpesvirus reactivations during and after severe drug eruptions: their relation to the clinical phenotype and therapeutic outcome. Allergy. 2014;69(6):798–805.
- Yachoui R, Kolasinski SL, Feinstein DE. Mycoplasma pneumoniae with atypical stevens-johnson syndrome: a diagnostic challenge. Case Rep Infect Dis. 2013;2013:457161.
- Torres-Navarro I, de Unamuno-Bustos B, Botella-Estrada R. Systematic review of BRAF/MEK inhibitors-induced Severe Cutaneous Adverse Reactions (SCARs). J Eur Acad Dermatol Venereol. 2021;35(3):607–614.
- Plachouri KM, Vryzaki E, Georgiou S. Cutaneous adverse events of immune checkpoint inhibitors: a summarized overview. Curr Drug Saf. 2019;14(1):14–20.
- Pavlos R, Mallal S, Ostrov D, et al. Fever, rash, and systemic symptoms: understanding the role of virus and HLA in severe cutaneous drug allergy. J Allergy Clin Immunol Pract. 2014;2(1):21–33.
- Nguyen DV, Vidal C, Chu HC, et al. Human leukocyte antigen-associated severe cutaneous adverse drug reactions: from bedside to bench and beyond. Asia Pac Allergy. 2019;9(3):e20.
- Yun J, Marcaida MJ, Eriksson KK, et al. Oxypurinol directly and immediately activates the drug-specific T cells via the preferential use of HLA-B*58:01. J Immunol. 2014;192(7):2984–2993.
- Gonzalez-Galarza FF, McCabe A, Santos EJMD, et al. Allele frequency net database (AFND) 2020 update: gold-standard data classification, open access genotype data and new query tools. Nucleic Acids Res. 2020;48(D1):D783–D788.
- Mendes D, Alves C, Loureiro M, et al. Drug-induced hypersensitivity: a 5-year retrospective study in a hospital electronic health records database. J Clin Pharm Ther. 2019;44(1):54–61.
- Tang MM, Fok JS, Thong BY-H, et al. Diagnostic procedures & practices in drug allergy/hypersensitivity: a survey of 13 Asian countries. Asia Pac Allergy. 2020;10(4):e36.
- Guo C, Xie X, Li J, et al. Pharmacogenomics guidelines: current status and future development. Clin Exp Pharmacol Physiol. 2019;46(8):689–693.
- Phillips EJ, Sukasem C, Whirl‐Carrillo M, et al. Clinical pharmacogenetics implementation consortium guideline for HLA genotype and use of Carbamazepine and Oxcarbazepine: 2017 update. Clin Pharmacol Ther. 2018;103(4):574–581.
- Khanna D, Fitzgerald JD, Khanna PP, et al. 2012 American College of Rheumatology guidelines for management of gout. Part 1: systematic nonpharmacologic and pharmacologic therapeutic approaches to hyperuricemia. Arthritis Care Res (Hoboken). 2012;64(10):1431–1446.
- Sorich MJ, Wiese MD, Pekarsky B. Cost-effectiveness of genotyping to guide treatment. Pharmacogenomics. 2014;15(6):727–729.
- Hoomans T, Severens JL. Economic evaluation of implementation strategies in health care. Implement Sci. 2014;9(1):168.
- Choi H, Mohit B. Cost-effectiveness of screening for HLA-B*1502 prior to initiation of carbamazepine in epilepsy patients of Asian ancestry in the United States. Epilepsia. 2019;60(7):1472–1481.
- Rattanavipapong W, Koopitakkajorn T, Praditsitthikorn N, et al. Economic evaluation of HLA-B*15:02 screening for carbamazepine-induced severe adverse drug reactions in Thailand. Epilepsia. 2013;54(9):1628–1638.
- Tiamkao S, Jitpimolmard J, Sawanyawisuth K, et al. Cost minimization of HLA-B*1502 screening before prescribing carbamazepine in Thailand. Int J Clin Pharm. 2013;35(4):608–612.
- Dong D, Sung C, Finkelstein EA. Cost-effectiveness of HLA-B*1502 genotyping in adult patients with newly diagnosed epilepsy in Singapore. Neurology. 2012;79(12):1259–1267.
- Chong HY, Mohamed Z, Tan LL, et al. Is universal HLA-B*15:02 screening a cost-effective option in an ethnically diverse population? A case study of Malaysia. Br J Dermatol. 2017;177(4):1102–1112.
- Plumpton CO, Yip VLM, Alfirevic A, et al. Cost-effectiveness of screening for HLA-A*31:01 prior to initiation of carbamazepine in epilepsy. Epilepsia. 2015;56(4):556–563.
- Jutkowitz E, Dubreuil M, Lu N, et al. The cost-effectiveness of HLA-B*5801 screening to guide initial urate-lowering therapy for gout in the United States. Semin Arthritis Rheum. 2017;46(5):594–600.
- Saokaew S, Tassaneeyakul W, Maenthaisong R, et al. Cost-effectiveness analysis of HLA-B*5801 testing in preventing allopurinol-induced SJS/TEN in Thai population. PLoS One. 2014;9(4):e94294.
- Chong HY, Lim YH, Prawjaeng J, et al. Cost-effectiveness analysis of HLA-B*58: 01 genetic testing before initiation of allopurinol therapy to prevent allopurinol-induced Stevens-Johnson syndrome/toxic epidermal necrolysis in a Malaysian population. Pharmacogenet Genomics. 2018;28(2):56–67.
- Kishore A, Petrek M. Next-Generation sequencing based HLA typing: deciphering immunogenetic aspects of Sarcoidosis. Front Genet. 2018;9:503.
- Wei CY, Lee MT, Chen YT. Pharmacogenomics of adverse drug reactions: implementing personalized medicine. Hum Mol Genet. 2012;21(R1):R58–65.
- Roden DM, Van Driest SL, Mosley JD, et al. Benefit of Preemptive Pharmacogenetic Information on Clinical Outcome. Clin Pharmacol Ther. 2018;103(5):787–794.
- Schildcrout JS, Denny JC, Roden DM. On the potential of preemptive genotyping towards preventing Medication-Related adverse events: results from the South Korean National Health Insurance Database. Drug Saf. 2017;40(1):1–2.
- Su SC, Hung S-I, Fan W-L, et al. Severe cutaneous adverse reactions: the pharmacogenomics from research to clinical implementation. Int J Mol Sci. 2016;17(11):1890.
- Dilokthornsakul P, Sawangjit R, Inprasong C, et al. Healthcare utilization and cost of Stevens-Johnson syndrome and toxic epidermal necrolysis management in Thailand. J Postgrad Med. 2016;62(2):109–114.
- *Sukasem C, Chantratita W. A success story in pharmacogenomics: genetic ID card for SJS/TEN. Pharmacogenomics. 2016;17(5):455–458.
- Satapornpong P, Jinda P, Jantararoungtong T, et al. Human Leukocyte Antigen Class I and Class II in Thai population: implication for clinical pharmacogenomics implementation. Front Pharmacol. 2020;11:78.
- Kumar D. The genomic and precision medicine in clinical practice –Current perspectives and future directions. The Physician. 2020;6(3):1–10.
- Huser V, Sincan M, Cimino JJ. Developing genomic knowledge bases and databases to support clinical management: current perspectives. Pharmgenomics Pers Med. 2014;7:275–283.
- Keeling NJ, Rosenthal MM, West-Strum D, et al. Preemptive pharmacogenetic testing: exploring the knowledge and perspectives of US payers. Genet Med. 2019;21(5):1224–1232.
- Ngorsuraches S, Meng W, Kim B-Y, et al. Drug reimbursement decision-making in Thailand, China, and South Korea. Value Health. 2012;15(1 Suppl):S120–5.
- Sung C, Tan L, Limenta M, et al. Usage pattern of Carbamazepine and Associated Severe Cutaneous Adverse Reactions in Singapore following implementation of HLA-B*15:02 genotyping as standard-of-Care. Front Pharmacol. 2020;11:527.
- Thong BY. Stevens-Johnson syndrome/toxic epidermal necrolysis: an Asia-Pacific perspective. Asia Pac Allergy. 2013;3(4):215–223.
- Dean L, Kane M. Allopurinol Therapy and HLA-B*58:01 genotype. In: Pratt VM, Scott SA, Pirmohamed M., et al. editors. Medical Genetics Summaries. Bethesda (MD): National Center for Biotechnology Information (US); 2012.
- Satapornpong P, Pratoomwun J, Rerknimitr P, et al. HLA-B*13:01 is a predictive marker of Dapsone-Induced severe cutaneous adverse reactions in Thai patients. Front Immunol. 2021;12:661135.
- Pratoomwun J, Thomson P, Jaruthamsophon K. Characterization of T-Cell responses to SMX and SMX-NO in Co-Trimoxazole Hypersensitivity patients expressing HLA-B*13:01. Front Immunol. 2021;12:658593.
- Sukasem C, Jantararoungtong T, Koomdee N, et al. Pharmacogenomics research and its clinical implementation in Thailand: lessons learned from the resource-limited settings. Drug Metab Pharmacokinet. 2021;39:100399.
- Puangpetch A, Koomdee N, Chamnanphol M, et al. HLA-B allele and haplotype diversity among Thai patients identified by PCR-SSOP: evidence for high risk of drug-induced hypersensitivity. Front Genet. 2015;5:478.