ABSTRACT
Introduction
Hepatic drug metabolism is important in improving drug dosing strategies in sepsis. Pharmacokinetics in the critically ill population are severely altered due to changes in absorption, distribution, excretion and metabolization. Hepatic drug metabolism might be altered due to changes in hepatic blood flow, drug metabolizing protein availability, and protein binding. The purpose of this review is to examine evidence on whether hepatic drug metabolism is significantly affected in septic patients, and to provide insights in the need for future research.
Areas covered
This review describes the effect of sepsis on hepatic drug metabolism in humans. Clinical trials, pathophysiological background information and example drug groups are further discussed. The literature search has been conducted in Embase, Medline ALL Ovid, and Cochrane CENTRAL register of trials.
Expert opinion
Limited research has been conducted on drug metabolism in the sepsis population, with some trials having researched healthy individuals using endotoxin injections. Notwithstanding this limitation, hepatic drug metabolism seems to be decreased for certain drugs in sepsis. More research on the pharmacokinetic behavior of hepatic metabolized drugs in sepsis is warranted, using inflammatory biomarkers, hemodynamic changes, mechanical ventilation, organ support, and catecholamine infusion as possible confounders.
1. Introduction
Sepsis is a life-threatening and dysregulated systemic response to an infection [Citation1]. Patients with sepsis or – in the case of circulatory failure – septic shock are usually admitted to the intensive care unit (ICU), for treatment with intravenous (IV) drugs, intensive monitoring, and organ support therapy [Citation2]. The average mortality in these patients ranges from 24% in the case of sepsis to 35% in the case of septic shock [Citation3,Citation4]. According to the Surviving Sepsis Campaign guidelines, several drugs are of great importance for treating sepsis, including antimicrobials, corticosteroids, sedatives, analgesics, and vasoactive drugs [Citation5,Citation6].
Drug pharmacokinetics (PK) in critically ill patients could be severely altered and vary over the period of admission [Citation7,Citation8]. Currently, drug dosing strategies in septic patients are empirically chosen. The guidelines are mostly based upon PK studies in healthy volunteers and do not take into account the extreme physiological changes in sepsis. Although some drugs are titrated based on clinical effects, for other drugs the clinical response is not directly measurable. The PK principles of absorption, distribution, metabolization, and excretion (also known as ADME) can be altered in septic patients [Citation9].
With regard to absorption, in severe and acute organ dysfunction, drugs are usually given intravenously, ensuring rapid and 100% absorption of the drugs. However, if a drug is given orally, it should be borne in mind that gastric motility and emptying are severely reduced in sepsis, which slows the absorption of drugs in the gastrointestinal (GI) tract [Citation10]. Other factors that can influence GI absorption of drugs are mucosal edema, splanchnic hypoperfusion, continuous feeding, nasogastric suctioning, and prescription of proton-pump inhibitors for stress-ulcer prophylaxis [Citation11]. When drugs are administrated intravenously, these factors are avoided.
Secondly, drug distribution may be altered as intravenous fluid therapy is a common treatment of hypotension in the case of sepsis. Due to this treatment and due to the increased capillary permeability caused by sepsis, the volume of distribution of hydrophilic drugs increases [Citation12,Citation13]. Moreover, treatment with extracorporeal devices could affect the PK parameters. Extra-corporeal membrane oxygenation (ECMO) can increase the volume of distributions of drugs due to drug sequestration with the circuit, hemodilution and aforementioned physiologic changes due to critical illness [Citation14,Citation15]. Furthermore, continuous renal replacement therapy (CRRT) is associated with changes in volume of distribution and increased elimination of drugs with high renal clearance [Citation16].
Furthermore, albumin binding and alpha1-acid glycoprotein (AGP) binding are altered in sepsis [Citation17,Citation18]. A decrease in albumin binding due to frequent hypoalbuminemia and an increase in AGP binding, since this is an acute-phase protein, is often observed [Citation9]. These factors may lead to differences in active fractions of high protein-binding drugs, since the partition that is protein bound has no pharmacological activity.
Thirdly, the excretion of drugs can be either increased or decreased during sepsis. Acute kidney injury (AKI) occurs in up to 50% of the sepsis population, with around 30% of all patients requiring renal replacement therapy (RRT) [Citation19]. Decreased excretion of hydrophilic drugs and active metabolites may lead to toxic levels and potentially harmful side-effects. On the other hand, increased drug clearance is often described in the acute phase of sepsis [Citation20,Citation21]. Although the exact mechanism is not yet clear, mostly young male patients with augmented renal creatinine clearance (ARC) are especially at risk for underdosing [Citation22,Citation23].
Lastly, drug metabolism has sparingly been researched in sepsis. To our knowledge, the potential changes in drug metabolism in septic patients have not yet been discussed and reviewed. Therefore, the main purpose of this review is to examine evidence on whether hepatic drug metabolism is significantly affected in septic patients and to provide insights into the need for future research.
2. Methodology
2.1. Search
We conducted a literature search in August 2021 without restrictions on the publication date. Three databases (Embase, Medline ALL Ovid, and Cochrane CENTRAL register of trials) were searched to assess the literature on the effect of sepsis on hepatic drug metabolism in adults. Detailed search terms can be found in supplementary file 1.
2.2. Eligibility criteria and study selection
Studies reporting the effect of sepsis on hepatic drug metabolism in adults were eligible for inclusion. Titles and abstracts were screened to identify relevant publications. Articles were excluded if they exclusively researched the pediatric population, did not describe the septic population or were clinical cases, reviews, or letters. Reference lists of the included articles were screened for additional studies. The references from the dataset were imported into Endnote X9 (Clarivate, 2013).
2.3. Data extraction
We extracted the following data from each included study: author, year of publication, number of subjects, broad subject characteristics, studied drugs, the effect of sepsis on hepatic drug clearance, and the influence of inflammatory cytokines on hepatic drug clearance.
3. Hepatic drug metabolism in sepsis
3.1. Study selection
shows a flowchart of the selection process. A total of 3538 articles were extracted after removing duplicates. After screening the title and abstract, 3526 articles were excluded and 6 additional articles were identified after screening the reference list. A total of 18 articles were included for full-text analysis, of which nine were found that met the inclusion criteria () [Citation24–32]. Of these articles, three simulated sepsis in healthy subjects through injection with endotoxins [Citation24–26]. One article researched the metabolism of midazolam in consecutive ventilated ICU patients [Citation27]. Toft et al. investigated the metabolization of theophylline and ethylenediamine after infusion in six septic patients with multi-organ failure [Citation28]. Groeger et al. observed naloxone clearance in four patients admitted to the ICU with septic shock [Citation29]. Finally, three described the metabolization of drugs in healthy volunteers compared to admitted critically ill patients with sepsis or septic shock studies [Citation30–32].
Table 1. Characteristics and outcomes of the studies included in the review.
3.2. Sepsis as a predictor of metabolism
We found that sepsis had a significant influence on hepatic metabolism in eight of the nine included studies. Shedlofsky et al. showed that there was a decrease in the metabolism of phenazone, hexobarbital and theophylline in male healthy volunteers after endotoxin administration [Citation24]. This effect was at maximum for all studied drugs at 48 hours after the first endotoxin administration. After repeating this study on women, similar results were shown [Citation25].
Furthermore, Shelly et al. showed that septic shock led to a decrease in the metabolism of midazolam into the main metabolite hydroxymidazolam in ventilated ICU patients [Citation27]. Metabolism rates returned to a normal level after their conditions improved. Mann et al. observed a lower metabolism in patients with sepsis compared to healthy subjects [Citation30]. . Central venous pressure (CVP) showed a strong correlation with decreased hepatic clindamycin metabolism.
Kruger et al. observed a higher maximum concentration and area under the curve (AUC) of atorvastatin in septic patients [Citation31]. Interestingly, the influence of concomitant cytochromes P450 (CYP) 3A4-inhibitors in ICU patients was even greater on both parameters than sepsis. Toft et al. observed the clearance of theophylline and ethylenediamine after infusion with a solution of both (aminophylline). Ethylenediamine is added to theophylline to increase its solubility. The researchers saw a decrease in clearance of theophylline and a threefold increase in half-life. Ethylenediamine had a clearance of 54% compared to normal with a 6.5 times increase of distribution to the peripheral tissues. Macnab et al. observed an increased half-life of morphine in combination with a decreased liver blood flow when comparing this to ICU patients without shock [Citation32]. Groeger et al. noticed that after stopping the infusion of naloxone, the blood levels remained stable. This can occur due to drug diffusion from peripheral tissue toward the bloodstream in combination with a slow clearance of the drug [Citation29]. Finally, Polyac et al. observed no significant decrease in the metabolism of chlorzoxazone with high heterogeneity in response [Citation26].
In conclusion, we describe an association of decreased hepatic drug (phenazone, hexobarbital, theophylline, etyhlenediamine, morphine, and midazolam) metabolism during sepsis in six out of nine studies [Citation24,Citation25,Citation27,Citation28,Citation30,Citation32]. Naloxone might have decreased metabolism, but the constant plasma levels after cessation of administration might also be explained by constant diffusion from peripheral compartments [Citation29]. A higher AUC was found for oral administration of atorvastatin, indirectly indicating a decrease of metabolic clearance [Citation31].
3.3. Inflammation as a predictor of metabolism
Interleukins (IL) and tumor necrosis factor alpha (TNF-α) are cytokines that play a major role in the inflammatory response [Citation33]. Shedlofsky et al. found a strong inverse correlation between peak TNF-α levels (r = 0.84) and IL-6 levels (r = 0.86) and change in phenazone metabolism 48 hours after endotoxin injection in adult males. In women, the same relationship was found, although the correlation coefficients were slightly lower (r = 0.54 and r = 0.70, respectively). Polyac et al. found no correlation between metabolism and IL-6 levels.
3.4. Pathophysiological background
Two major enzymatic pathways are responsible for hepatic drug metabolization: phase I and phase II metabolism. The main pathway is phase I metabolism, which is mostly facilitated by CYP [Citation34]. CYP has multiple isozymes with various amounts of expression and therefore differences in metabolization capacity. Phase II metabolism consists of conjugation with an endogenous substance.
A factor of great importance in hepatic metabolism is the extraction ratio. Depending on the characteristics of drugs, multiple factors can affect the drug metabolization rate (). Drugs with a high metabolizing enzyme capacity and rapid diffusion toward the hepatocyte have a high extraction ratio. Metabolization is therefore mostly dependent on the hepatic flow. The influence of protein binding and metabolizing capacity is of more importance for drugs with a low ratio [Citation35]. In the case of intermediate extraction ratios blood flow, metabolizing capacity, and plasma binding are all important. A recent review examined the influence of inflammation on metabolism [Citation36], but the authors did not assess sepsis syndrome as a whole. Inflammation has been proven to play a great role in decreasing drug metabolism due to altered enzyme expression [Citation37]. Yet, the influence of the altered hepatic blood flow and changes in serum protein binding is unclear.
Figure 2. Influencing factors on hepatic metabolization rate are (1) protein binding, (2) hepatic blood flow, and (3) enzyme expression. In sepsis, protein binding can be increased or decreased depending on binding protein, such as albumin or alpha1-acid glycoprotein (AGP). Hepatic blood flow is usually decreased in sepsis, but can be increased in early sepsis. Enzyme expression is usually decreased in sepsis.
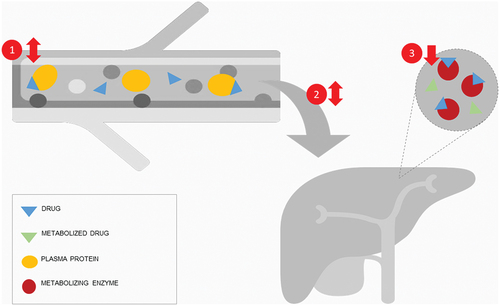
The effect of inflammation on drug metabolism has been researched thoroughly in cancer, heart failure and rheumatoid diseases [Citation36,Citation38,Citation39]. Multiple mechanisms are described to explain this decrease in hepatic drug metabolism [Citation40]. One of these mechanisms is the effect of endotoxins on Kupffer cells [Citation41]. Activation of the Kupffer cell leads to the release of cytokines near the hepatocytes, resulting in altered CYP-enzyme expression and drug metabolism [Citation42]. Furthermore, liver injury due to ischemia or endotoxins also leads to repressed drug metabolism [Citation43]. Sepsis has a variable effect on different enzymes, but they are most prominently downregulated [Citation36]. A decrease in overall and hepatic metabolism rate in sepsis was also seen in animal models [Citation44]. In addition, drug excretion into bile was similarly decreased. Whether these are translatable to humans remains unclear, specifically because of the intense hemodynamic changes in critically ill patients.
Besides the abovementioned mechanisms, sepsis and septic shock leads to a change in splanchnic blood flow and oxygen consumption [Citation45,Citation46]. Even though the splanchnic blood flow usually increases during the early phases of sepsis, splanchnic oxygen consumption is also increased, leading to a decrease in oxygen that reaches the liver through the portal vein [Citation47]. Moreover, infusion of catecholamine increases the splanchnic blood flow in sepsis but does not affect drug metabolization of the high extraction ratio drug lidocaine [Citation48]. This suggests that another factor, such as protein binding or enzyme expression, might be a limiting factor in sepsis. Another factor affecting hepatic and splanchnic circulation is the presence of mechanical ventilation [Citation49]. Due to the positive pressure in the thorax, blood flow is decreased, which could decrease the metabolism of high extraction ratio drugs.
Septic changes can also result in alterations in protein binding of the drugs and therefore changes in the free fraction. Hypoalbuminemia is prevalent in critical illness, which results in a higher free fraction of albumin-bound drugs [Citation50]. Septic shock itself may result in a larger free fraction of some albumin-bound drugs [Citation51], suggesting that the binding capacity of the available albumin also might be affected. For drugs bound to AGP, the effects are different from albumin-bound drugs: since AGP production is upregulated during inflammation, drugs bound to this protein have a lower free fraction [Citation9]. A higher free fraction could result in a higher clearance of drugs, depending on the limiting factor for metabolism.
Interestingly, Polyac et al. saw no change in hepatic drug metabolism of chlorzoxazone in healthy volunteers injected with endotoxin [Citation26]. Chlorzoxazone is a substrate of CYP2E1. Anninat et al. describes that CPY2E1 is not downregulated due to the effect of catecholamines in isolated human liver cells [Citation52]. Other CYP enzymes such as CYP3A4, CYP2B6, and CYP1A2 were significantly downregulated in the hepatocyte in the same experiment. Catecholamines induce IL-6 production, exerting a similar inflammatory effect. The fact that Polyac et al. measured no change in hepatic drug metabolism may suggest that the main mechanism of decreased metabolization in sepsis is facilitated by the effect of cytokines on CYP-enzymes [Citation26].
3.5. Example drugs
3.5.1. Antibiotics and antifungals
Antimicrobials are one of the most important interventions in the treatment of sepsis. Most antibiotics are renally excreted without extensive metabolization. The surviving sepsis campaign advises adjusting antimicrobials based on PK changes in sepsis, yet little is known about drug metabolism changes in these patients. Clindamycin, a macrolide antibiotic, seems to have decreased metabolism [Citation30]. Antifungals are mostly metabolized before excretion and are frequently a subject of therapeutic drug monitoring (TDM), an individualized dosing strategy based on assessments of serum drug levels, since there is a clear correlation between dose and (hepato-)toxicity [Citation53]. Voriconazole for example, a drug metabolized by CYP2C19, was significantly less metabolized in patients with increased CRP [Citation54]. On the contrary, other studies reported an increased risk of underdosing with high inter-individual variations, suggesting an increase in hepatic metabolism [Citation55,Citation56]. TDM might prove to be a solution to individualize dosing [Citation57,Citation58].
3.5.2. Corticosteroids
Other frequently prescribed drugs in sepsis are corticosteroids as an anti-inflammatory treatment. They are advised to administer in septic shock if adequate fluid resuscitation and vasopressor therapy are not enough to restore hemodynamic stability. Side-effects, such as glucose dysregulation are possibly dose-related [Citation59]. Prednisone is a pro-drug of prednisolone, the effective compound. Metabolism of both compounds involves both phase I and phase II reactions, although the degree of involvement of both phases is still unclear [Citation35,Citation60]. Dexamethasone is metabolized by CYP3A4. In chemotherapy, blood urea and alanine transaminase (ALT) levels and co-administration of a CYP3A4 inhibitor seem to affect effect on the clearance of dexamethasone, indicating a similarly combined clearance as prednisolone [Citation61]. Methylprednisolone is also cleared by CYP3A4 [Citation62]. In acute respiratory distress syndrome (ARDS) no difference was found in clearance compared to healthy individuals, but they did find that it has a self-inducing effect, increasing clearance over time [Citation63]. Due to the unavailability of specific ICU or sepsis PK models, and the anti-inflammatory effect of these drugs that could affect hepatic metabolization, it is difficult to predict what would happen to the PK in sepsis.
3.5.3. Sedatives
Sedatives, such as midazolam, barbiturates, and propofol are often administered during ICU admission to facilitate mechanical ventilation. Midazolam clearance is significantly reduced in severe sepsis [Citation27]. Midazolam clearance is also decreased with increased APACHE II scores [Citation64]. In children similar effects are observed, C-reactive protein (CRP) and organ failure were significantly and inversely associated with drug clearance [Citation65]. A CRP of 300 mg/L was associated with a 65% lower clearance compared with 10 mg/L, and three failing organs were associated with a 35% lower clearance compared with one failing organ. However, a population PK model for adult patients with sepsis is still missing. Barbiturates are rarely used in the adult sepsis population nowadays. Shedlofsky et al. showed that metabolization of the barbiturate hexobarbital was significantly decreased after injecting endotoxin [Citation24,Citation25]. A frequently prescribed sedative is propofol. This is a drug with a high extraction ratio, which means that blood flow should have the most important influence on metabolism. However, in a small PK study in 29 patients, sepsis was not a significant covariate of clearance [Citation66]. For future research, PK and PD of benzodiazepines such as midazolam should be examined, since dosing too high could lead to side effects [Citation67].
3.5.4. Analgesics
Opioids are often needed for treatment during mechanical ventilation. Opioid accumulation is frequently a concern in ICU treatment. A generally and often prescribed opioid is morphine. Morphine is metabolized into active metabolites, which then are eliminated by renal excretion. Morphine clearance is significantly decreased in critically ill patients with severe systemic inflammatory response syndrome, suggesting a decrease in metabolism [Citation68]. In the same study the metabolism of lidocaine showed no change. Even though liver blood flow would seem to be the most important factor in the clearance of morphine due to its high extraction ratio, other mechanisms might be involved. Macnab et al. found that clearance and liver blood flow was decreased in septic shock [Citation32]. Fentanyl is another often used opioid, cleared by CYP3A4 into inactive metabolites. Another frequently used opioid is remifentanil. This drug has a unique route of metabolization through plasma esterases, which guarantees a fairly constant and short half-life, independent of organ insufficiency [Citation69].
4. Conclusion
The present review provides support for the hypothesis that hepatic drug metabolism is significantly altered in sepsis. However, limited research has been conducted on drug metabolism in this specific population, with some trials having researched healthy individuals using endotoxin injections. Notwithstanding this limitation, the present review suggests that hepatic drug metabolism is decreased for certain drugs in sepsis. More research on the pharmacokinetic behavior of hepatic metabolization of drugs in critically ill patients is warranted.
5. Expert opinion
PK in septic patients is highly variable and it seems that for some drugs, hepatic metabolism is decreased. Understanding which factors are responsible for the variability of drug metabolism in septic patients could help adjust the dosing strategy and preserve therapeutic effectiveness while decreasing toxicity. The current evidence does not fully describe which changes in sepsis are responsible for changes in hepatic metabolism in the clinical situation. This may result in dosing strategies that are not optimal for these severely ill patients. In order to optimize dosing strategies, variability and confounders for drug metabolism will need to be identified.
Some mechanistic alterations have been named that could affect the metabolization of drugs in sepsis. The hepatic blood flow can be either increased in early sepsis or decreased due to shock. Free drug fractions are altered based on their binding on either albumin or AGP in the case of acidic and basic drugs, respectively. Finally, metabolizing enzymes are usually downregulated, but sometimes unaffected based on the metabolic pathway of drug clearance. This variety in mechanistic response complicates predicting the alteration of drug metabolism in an individual patient.
To adjust for the wide variability of drug metabolism response, efforts to optimize dosing in the early phase of sepsis have to be made. Adjusting dosing based on measuring drug or metabolite concentrations – TDM – is a possibility [Citation58]. Furthermore, model-informed precision dosing (MIPD) represents a dosing tool in which a mathematical model in combination with individually measured patient characteristics and disease characteristics are used to calculate the optimal dose [Citation70]. If more information is known about covariates for hepatic drug metabolism during sepsis, doses could be personalized as early as the first dose using MIPD. Continuous drug-level monitoring with microneedling or microdialysis, in combination with hemodynamic and biomarker data from the patient records, could be used in these frameworks to optimize dosing during the entire admission [Citation71,Citation72]. Especially for drugs with no apparent immediate clinical effect, such as antimicrobials, early dose optimization for efficacy is important. Specifically for hepatic dysfunction, maximum liver capacity testing could be of added use for integration in an MIPD framework [Citation73,Citation74]. The effect of other pathophysiological changes during sepsis will need to be assessed before or during implementation into PK models.
Some discussed studies were conducted on healthy subjects simulating sepsis with endotoxin injections. The translation of these models toward patients with sepsis is questioned [Citation75]. Even though the clinical response seems to mimic sepsis, the cytokine response and timing are not comparable to other animal infection models, such as those induced by cecal ligation and puncture [Citation76]. Furthermore, endotoxin injections cannot simulate the complex treatment of sepsis, since the effect of admission, mechanical ventilation, organ failure, organ support, and catecholamine infusion are not considered. These parameters might be important for the estimation of the variability of metabolism, since they affect hepatic blood flow, enzyme availability or unbound drug concentrations. The response triggered by endotoxin injection might reveal the direction of change in hepatic metabolism and clearance of drugs. However, the magnitude of the effect cannot be extrapolated due to the differences in cytokine response.
Some efforts have already been made to quantify the hepatic drug metabolism rate and may be used to optimize dosing [Citation74,Citation77]. Future research will need to assess the PK of hepatic metabolized medication in the sepsis patient in the ICU. In addition, future research should also focus on collecting data on inflammatory biomarkers, hemodynamic changes, mechanical ventilation, organ support, and catecholamine infusion as possible confounders.
Conclusively, due to the sparse research on hepatic drug metabolism during sepsis, drug dosing will need to be assessed for this population. Early recognition of drugs that are at risk of toxicity or underdosing and adjusting doses using TDM play a vital role in this process.
Article highlights
Sepsis seems to result in lower clearance of most hepatically metabolized drugs.
Little research has been performed on the pharmacokinetics of drugs that are hepatically metabolized in septic patients.
Hepatic drug metabolism in sepsis is probably changed due to an alteration in protein binding, unbound fraction and/or hepatic blood flow. However, the influence of these factors is unclear and sparsely researched.
Future research should collect data on confounders, such as inflammatory biomarkers, hemodynamic changes and catecholamine infusion.
Novel techniques, such as model-informed precision dosing and liver metabolizing capacity tests, could aid in adjusting doses in septic patients.
This box summarizes key points contained in the article.
Declaration of interest
The authors have no relevant affiliations or financial involvement with any organization or entity with a financial interest in or financial conflict with the subject matter or materials discussed in the manuscript. This includes employment, consultancies, honoraria, stock ownership or options, expert testimony, grants or patents received or pending, or royalties.
Reviewer disclosures
Peer reviewers on this manuscript have no relevant financial or other relationships to disclose.
Author contributions
T Ewoldt and H Endeman conceived the presented idea. T Ewoldt gathered the data and discussed these results with A Abdulla and H Endeman. T Ewoldt then wrote the first and subsequent drafts in consultation with A A,bdulla H Endeman and B Koch. All authors discussed the results and contributed to the final manuscript.
Supplemental Material
Download MS Word (12.5 KB)Supplementary material
Supplemental data for this article can be accessed here
Additional information
Funding
References
- Singer M, Deutschman CS, Seymour CW, et al., The Third International Consensus Definitions for Sepsis and Septic Shock (Sepsis-3). Jama. 315(8): 801–810. 2016.
- Rivers E, Nguyen B, Havstad S, et al. Early Goal-Directed Therapy in the Treatment of Severe Sepsis and Septic Shock. N Engl J Med. 2001;345(19):1368–1377.
- Bauer M, Gerlach H, Vogelmann T, et al. Mortality in sepsis and septic shock in Europe, North America and Australia between 2009 and 2019—results from a systematic review and meta-analysis. Crit Care. 2020;24(1):239.
- Vincent J-L, Jones G, David S, et al. Frequency and mortality of septic shock in Europe and North America: a systematic review and meta-analysis. Crit Care. 2019;23(1):196.
- Rhodes A, Evans LE, Alhazzani W, et al. Surviving Sepsis Campaign: international Guidelines for Management of Sepsis and Septic Shock: 2016. Intensive Care Med. 2017;43(3):304–377.
- Evans L, Rhodes A, Alhazzani W, et al. Surviving sepsis campaign: international guidelines for management of sepsis and septic shock 2021. Intensive Care Med. 2021;47(11):1181–1247.
- Smith BS, Yogaratnam D, Levasseur-Franklin KE, et al., Introduction to Drug Pharmacokinetics in the Critically III Patient. Chest. 141(5): 1327–1336. 2012.
- Roberts DJ, Hall RI. Drug absorption, distribution, metabolism and excretion considerations in critically ill adults. Expert Opin Drug Metab Toxicol. 2013;9(9):1067–1084.
- De Paepe P, Belpaire FM, Buylaert WA. Pharmacokinetic and Pharmacodynamic Considerations When Treating Patients with Sepsis and Septic Shock. Clin Pharmacokinet. 2002;41(14):1135–1151.
- Nguyen NQ, Ng MP, Chapman M, et al. The impact of admission diagnosis on gastric emptying in critically ill patients. Crit Care. 2007;11(1):R16.
- Huang L, Quartin A, Jones D, et al. Intensive Care of Patients with HIV Infection. N Engl J Med. 2006;355(2):173–181.
- Huang Y, Yang J, Xie J, et al., Association Between Pathophysiology and Volume of Distribution Among Patients With Sepsis or Septic Shock Treated With Imipenem: a Prospective Cohort Study. J Infect Dis. 221(Supplement_2): S272–S278. 2020.
- Marik PE. Aminoglycoside Volume of Distribution and Illness Severity in Critically Ill Septic Patients. Anaesth Intensive Care. 1993;21(2):172–173.
- Mulla H, Pooboni S. Population pharmacokinetics of vancomycin in patients receiving extracorporeal membrane oxygenation. Br J Clin Pharmacol. 2005;60(3):265–275.
- Cheng V, Abdul-Aziz MH, Roberts JA, et al. Optimising drug dosing in patients receiving extracorporeal membrane oxygenation. J Thorac Dis. 2018;10(Suppl 5):S629–S641.
- Pea F, Viale P, Pavan F, et al. Pharmacokinetic Considerations for Antimicrobial Therapy in Patients Receiving Renal Replacement Therapy. Clin Pharmacokinet. 2007;46(12):997–1038.
- Roberts JA, Pea F, Lipman J. The Clinical Relevance of Plasma Protein Binding Changes. Clin Pharmacokinet. 2013;52(1):1–8.
- Smith SA, Waters NJ. Pharmacokinetic and Pharmacodynamic Considerations for Drugs Binding to Alpha-1-Acid Glycoprotein. Pharm Res. 2018;36(2):30.
- Bellomo R, Kellum JA, Ronco C, et al. Acute kidney injury in sepsis. Intensive Care Med. 2017;43(6):816–828.
- Hobbs AL, Shea KM, Roberts KM, et al. Implications of Augmented Renal Clearance on Drug Dosing in Critically Ill Patients: a Focus on Antibiotics. Pharmacotherapy. 2015;35(11):1063–1075.
- Baptista JP, Sousa E, Martins PJ, et al. Augmented renal clearance in septic patients and implications for vancomycin optimisation. Int J Antimicrob Agents. 2012;39(5):420–423.
- Abdulla A, Ewoldt TMJ, Purmer IM, et al. A narrative review of predictors for β-lactam antibiotic exposure during empirical treatment in critically ill patients. Expert Opin Drug Metab Toxicol. 2021;17(4):359–368.
- Claus BOM, Hoste EA, Colpaert K, et al. Augmented renal clearance is a common finding with worse clinical outcome in critically ill patients receiving antimicrobial therapy. J Crit Care. 2013;28(5):695–700.
- Shedlofsky SI, Israel BC, and McClain CJ, et al. Endotoxin administration to humans inhibits hepatic cytochrome P450- mediated drug metabolism . J CLIN INVEST. 1994;94(6):2209–2214.
- Shedlofsky SI, Israel BC, Tosheva R, et al., Endotoxin depresses hepatic cytochrome P450-mediated drug metabolism in women. Br J Clin Pharmacol. 43(6): 627–632. 1997.
- Poloyac SM, Tosheva RT, Gardner BM, et al., The effect of endotoxin administration on the pharmacokinetics of chlorzoxazone in humans. Clin Pharmacol Ther. 66(6): 554–562. 1999.
- Shelly MP, Mendel L, Park GR. Failure of critically ill patients to metabolise midazolam. Anaesthesia. 1987;42(6):619–626.
- Toft P, Heslet L, Hansen M, et al., Theophylline and ethylenediamine pharmacokinetics following administration of aminophylline to septic patients with multiorgan failure. Intensive Care Med. 17(8): 465–468. 1991.
- Groeger JS, Inturrisi CE. High-dose naloxone: pharmacokinetics in patients in septic shock. Crit Care Med. 1987;15(8):751–756.
- Mann HJ, Townsend RJ, Fuhs DW, et al. Decreased hepatic clearance of clindamycin in critically ill patients with sepsis. Clin Pharm. 1987;6(2):154–159.
- Kruger PS, Freir NM, Venkatesh B, et al., A preliminary study of atorvastatin plasma concentrations in critically ill patients with sepsis. Intensive Care Med. 35(4): 717–721. 2009.
- Macnab MS, Macrae DJ, Guy E, et al., Profound reduction in morphine clearance and liver blood flow in shock. Intensive Care Med. 12(5): 366–369. 1986.
- Chaudhry H, Zhou J, and Zhong YIN, et al. Role of cytokines as a double-edged sword in sepsis. vivo. 2013;27(6):669–684.
- Zanger UM, Schwab M. Cytochrome P450 enzymes in drug metabolism: regulation of gene expression, enzyme activities, and impact of genetic variation. Pharmacol Ther. 2013;138(1):103–141.
- Bergmann TK, Barraclough KA, Lee KJ, et al. Clinical pharmacokinetics and pharmacodynamics of prednisolone and prednisone in solid organ transplantation. Clin Pharmacokinet. 2012;51(11):711–741.
- Shah RR, Smith RL. Inflammation-induced phenoconversion of polymorphic drug metabolizing enzymes: hypothesis with implications for personalized medicine. Drug Metab Dispos. 2015;43(3):400–410.
- Morgan ET. Impact of Infectious and Inflammatory Disease on Cytochrome P450–Mediated Drug Metabolism and Pharmacokinetics. Clin Pharmacol Ther. 2009;85(4):434–438.
- Rivory LP, Slaviero KA, Clarke SJ. Hepatic cytochrome P450 3A drug metabolism is reduced in cancer patients who have an acute-phase response. Br J Cancer. 2002;87(3):277–280.
- Frye RF, Schneider VM, Frye CS, et al. Plasma levels of TNF-α and IL-6 are inversely related to cytochrome P450-dependent drug metabolism in patients with congestive heart failure. J Card Fail. 2002;8(5):315–319.
- Lv C, Huang L. Xenobiotic receptors in mediating the effect of sepsis on drug metabolism. Acta Pharm Sin B. 2020;10(1):33–41.
- Jacob A, Zhou M, Wu R, et al. The role of hepatic cytochrome P-450 in sepsis. Int J Clin Exp Med. 2009;2(3):203–211.
- de Jong LM, Jiskoot W, Swen JJ, et al. Distinct Effects of Inflammation on Cytochrome P450 Regulation and Drug Metabolism: lessons from Experimental Models and a Potential Role for Pharmacogenetics. Genes (Basel). 2020;11(12):1509.
- Guo Y, Hu B, Xie Y, et al. Regulation of drug-metabolizing enzymes by local and systemic liver injuries. Expert Opin Drug Metab Toxicol. 2016;12(3):245–251.
- Yang KH, Lee MG. Effects of endotoxin derived from Escherichia coli lipopolysaccharide on the pharmacokinetics of drugs. Arch Pharm Res. 2008;31(9):1073–1086.
- Reinelt H, Radermacher P, Kiefer P, et al. Impact of exogenous β-adrenergic receptor stimulation on hepatosplanchnic oxygen kinetics and metabolic activity in septic shock. Crit Care Med. 1999;27(2):325–331.
- Dahn MS, Lange MP, Wilson RF, et al. Hepatic blood flow and splanchnic oxygen consumption measurements in clinical sepsis. Surgery. 1990;107(3):295–301.
- Pastor CM, Suter PM. Hepatic hemodynamics and cell functions in human and experimental sepsis. Anesthesia Analg. 1999;89(2):344–352.
- Jakob SM, Ruokonen E, Rosenberg PH, et al. Effect of dopamine-induced changes in splanchnic blood flow on MEGX production from lidocaine in septic and cardiac surgery patients. Shock. 2002;18(1):1–7.
- Dorinsky PM, Hamlin RL, Gadek JE. Alterations in regional blood flow during positive end- expiratory pressure ventilation. Crit Care Med. 1987;15(2):106–113.
- Vree TB, Shimoda M, Driessen JJ, et al. Decreased plasma albumin concentration results in increased volume of distribution and decreased elimination of midazolam in intensive care patients. Clin Pharmacol Ther. 1989;46(5):537–543.
- Ewoldt T, Abdulla A, and Gommers D, et al. Large variability of unbound active fraction of ceftriaxone in contrast to ciprofloxacin in plasma of critically ill patients. 2020;5078:ECCMID.
- Aninat C, Seguin P, Descheemaeke PN, et al. Catecholamines induce an inflammatory response in human hepatocytes. Crit Care Med. 2008;36(3):848–854.
- Tverdek FP, Kofteridis D, Kontoyiannis DP. Antifungal agents and liver toxicity: a complex interaction. Expert Rev Anti Infect Ther. 2016;14(8):765–776.
- Encalada Ventura MA, Span LFR, van den Heuvel ER, et al. Influence of inflammation on voriconazole metabolism. Antimicrob Agents Chemother. 2015;59(5):2942–2943.
- Spriet I, Annaert P, Meersseman P, et al. Pharmacokinetics of caspofungin and voriconazole in critically ill patients during extracorporeal membrane oxygenation. J Antimicrob Chemother. 2009;63(4):767–770.
- Hoenigl M, Duettmann W, Raggam RB, et al. Potential Factors for Inadequate Voriconazole Plasma Concentrations in Intensive Care Unit Patients and Patients with Hematological Malignancies. Antimicrob Agents Chemother. 2013;57(7):3262–3267.
- Neely M, Margol A, Fu X, et al. Achieving Target Voriconazole Concentrations More Accurately in Children and Adolescents. Antimicrob Agents Chemother. 2015;59(6):3090–3097.
- Li H, Li M, Yan J, et al. Voriconazole therapeutic drug monitoring in critically ill patients improves efficacy and safety of antifungal therapy. Basic Clin Pharmacol Toxicol. 2020;127(6):495–504.
- Gurwitz JH, Bohn RL, Glynn RJ, et al. Glucocorticoids and the risk for initiation of hypoglycemic therapy. Arch Internal Med. 1994;154(1):97–101.
- Schijvens AM, Ter Heine R, de Wildt SN, et al. Pharmacology and pharmacogenetics of prednisone and prednisolone in patients with nephrotic syndrome. Pediatr Nephrol. 2019;34(3):389–403.
- Nakade S, Ohno T, Kitagawa J, et al. Population pharmacokinetics of aprepitant and dexamethasone in the prevention of chemotherapy-induced nausea and vomiting. Cancer Chemother Pharmacol. 2008;63(1):75–83.
- Szefler SJ, Rose JQ, Ellis EF, et al. The effect of troleandomycin on methylprednisolone elimination. J Allergy Clin Immunol. 1980;66(6):447–451.
- Yates CR, Vysokanov A, Mukherjee A, et al. Time-variant increase in methylprednisolone clearance in patients with acute respiratory distress syndrome: a population pharmacokinetic study. J Clin Pharmacol. 2001;41(4):415–424.
- Swart EL, Zuideveld KP, de Jongh J, et al. Comparative population pharmacokinetics of lorazepam and midazolam during long-term continuous infusion in critically ill patients. Br J Clin Pharmacol. 2004;57(2):135–145.
- Vet NJ, Brussee JM, de Hoog M, et al. Inflammation and Organ Failure Severely Affect Midazolam Clearance in Critically Ill Children. Am J Respir Crit Care Med. 2016;194(1):58–66.
- Smuszkiewicz P, Wiczling P, Przybyłowski K, et al. The pharmacokinetics of propofol in ICU patients undergoing long-term sedation. Biopharm Drug Dispos. 2016;37(8):456–466.
- Devlin JW, Skrobik Y, Gélinas C, et al. Clinical Practice Guidelines for the Prevention and Management of Pain, Agitation/Sedation, Delirium, Immobility, and Sleep Disruption in Adult Patients in the ICU. Crit Care Med. 2018;46(9):e825–e873.
- Berkenstadt H, Segal E, Mayan H, et al. The pharmacokinetics of morphine and lidocaine in critically ill patients. Intensive Care Med. 1999;25(1):110–112.
- Wilhelm W, Kreuer S. The place for short-acting opioids: special emphasis on remifentanil. Crit Care. 2008;12(3):1–9.
- Wicha SG, Märtson A-G, Nielsen EI, et al. From Therapeutic Drug Monitoring to Model-Informed Precision Dosing for Antibiotics. Clin Pharmacol Ther. 2021;109(4):928–941.
- Kiang TKL, Ranamukhaarachchi SA, Ensom MHH. Revolutionizing therapeutic drug monitoring with the use of interstitial fluid and microneedles technology. Pharmaceutics. 2017;9(4):43.
- van der Mast JE, Nijsten MW, Alffenaar JWC, et al. In vitro evaluation of an intravenous microdialysis catheter for therapeutic drug monitoring of gentamicin and vancomycin. Pharmacol Res Perspect. 2019;7(4):e00483.
- Wicha SG, Frey OR, Roehr AC, et al. Linezolid in liver failure: exploring the value of the maximal liver function capacity (LiMAx) test in a pharmacokinetic pilot study. Int J Antimicrob Agents. 2017;50(4):557–563.
- Kirchner C, Sibai J, Schwier E, et al. Dosing of Antimycotic Treatment in Sepsis-Induced Liver Dysfunction by Functional Liver Testing with LiMAx. Case Rep Crit Care. 2019;2019. 10.1155/2019/5362514.
- Remick DG, Ward PA. Evaluation of endotoxin models for the study of sepsis. Shock. 2005;24:7–11.
- Remick DG, Newcomb DE, Bolgos GL, et al. Comparison of the mortality and inflammatory response of two models of sepsis: lipopolysaccharide vs. cecal ligation and puncture. Shock. 2000;13(2):110–116.
- Kaffarnik MF, Lock JF, Vetter H, et al. Early diagnosis of sepsis-related hepatic dysfunction and its prognostic impact on survival: a prospective study with the LiMAx test. Crit Care. 2013;17(5):R259.