ABSTRACT
Objectives: To compare antimicrobial and resource utilization with T2 Magnetic Resonance (T2MR) versus blood culture (BC) in patients with suspected bloodstream infection.
Methods: We systematically searched MEDLINE, EMBASE, and CENTRAL for randomized trials or observational controlled studies of patients with suspected bloodstream infection receiving a diagnosis with T2MR or BC. Using an inverse variance meta-analysis model, we reported mortality using the risk ratio (RR) and the remaining outcomes as the mean difference (MD).
Results: Fourteen studies were included in the meta-analysis. Time to detection (MD = −81 hours; p < 0.001) and time to species identification (MD = −77 hours; p < 0.001) were faster with T2MR. Patients testing positive on T2MR received targeted antimicrobial therapy faster (−42 hours; p < 0.001) and patients testing negative on T2MR were de-escalated from empirical therapy faster (−7 hours; p = 0.02) vs. BC. Length of intensive care unit stay (MD = −5.0 days; p = 0.03) and hospital stay (MD = −4.8 days; p = 0.03) were shorter with T2MR. Mortality rates were comparable between T2MR and BC (28.9% vs. 29.9%, RR = 1.02, p = 0.86).
Conclusion: Utilization of T2MR for identification of bloodstream pathogens provides faster time to detection, faster transition to targeted microbial therapy, faster de-escalation of empirical therapy, shorter ICU and hospital stay, and with comparable mortality rate versus BC.
1. Introduction
Sepsis is characterized by life-threatening acute organ dysfunction secondary to bacterial, fungal, or viral infection [Citation1] and affects more than 49 million individuals each year [Citation2]. Sepsis is responsible for 11 million deaths annually, representing 20% of the deaths globally [Citation2]. Sepsis also presents a significant economic burden since the average hospital stay for patients receiving a sepsis diagnosis costs 18,400 USD, which is twice the average cost compared to all other diagnoses [Citation3].
Sepsis management is usually initiated with fluid resuscitation, source infection control, and broad-spectrum antibiotics [Citation4]. Delays in prescribing appropriate antibiotic therapy (AAT) are associated with progression in seps is severity, higher rates of complications, de novo resistance, increased C. difficile infection prevalence, and increased health care costs [Citation5–11]. On the other hand, overuse or misuse of antimicrobial therapy is associated with a higher risk of adverse events and contributes to antimicrobial resistance. For these reasons, it is imperative to rapidly identify the specific causative pathogens to allow earlier AAT or to rule out bloodstream infection (BSI) with subsequent antimicrobial withdrawal or rapid de-escalation.
Blood cultures (BC) followed by post-BC species identification for positive tests remain the gold standard for diagnosing bacterial and fungal bloodstream infections (BSIs). However, major limitations of BC include slow turnaround time and suboptimal sensitivity [Citation4]. Consequently, there has been recent interest in developing rapid diagnostic tests (RDTs) to identify specific pathogens responsible for BSIs and to facilitate earlier administration of AAT or de-escalation of unnecessary antibiotics. Numerous RDTs are available to detect sepsis-causing pathogens yet most depend on waiting for BC results before processing, which may hinder their adoption into clinical workflows [Citation12]. There is a clear unmet need for faster, culture-independent diagnostic methods for specific pathogen identification in patients with BSI.
A magnetic resonance (MR)-based molecular diagnostic device (T2Dx, T2 Biosystems, Lexington, MA, U.S.A.) utilizing specific panels identifies the most prevalent and deadly bacterial (T2Bacteria panel) and fungal (T2Candida panel) species directly from complex matrices including unprocessed whole blood samples without the need for BC [Citation13] (Supplement Figure 1). The nanodiagnostic panels detect microbial cells in a fully automated process utilizing standard K2-EDTA vacutainer collection tubes. The mechanism of detection involves mechanically lysing red blood cells, using polymerase-chain reaction primers to amplify target DNA sequences, and hybridization of the amplicons to probe-enriched supraparamagnetic nanoparticles to provide species identification by measuring the MR signal produced via agglomeration of these nanoparticles. The T2Bacteria panel detects E. faecium, S. aureus, K. pneumoniae, P. aeruginosa, E. coli, and A. baumannii (the latter approved in Europe only). These ESKAPE pathogens are especially problematic owing to their antibiotic resistance mechanisms and nosocomial spread. The T2Candida panel detects the most common pathogenic Candida species that account for over 95% of candidemia at most hospitals, namely C. albicans/C. tropicalis, C. glabrata/C. krusei, and C. parapsilosis [Citation14]. While numerous papers have reported the diagnostic performance of T2MR [Citation15,Citation16], a comprehensive analysis regarding changes in antimicrobial prescribing patterns and impacts on resource utilization has not been undertaken. The purpose of this systematic review with meta-analysis was to compare antimicrobial and resource utilization with T2MR versus BC in patients with suspected BSI.
2. Methods
2.1. Literature search
The systematic review methodology adhered to the guidance set forth in the Preferred Reporting Items for Systematic Reviews and Meta-Analyses (PRISMA) statement [Citation17] and was prospectively registered with the ResearchRegistry (Review Registry UIN: reviewregistry1050). We searched MEDLINE, EMBASE, and CENTRAL for randomized controlled trials or observational studies of patients with suspected BSI receiving a diagnosis with T2MR or BC. The details of the MEDLINE search strategy are listed in Supplement Table 1. The syntax for EMBASE and CENTRAL was similar and adapted accordingly. We manually searched the Directory of Open Access Journals, Google Scholar, the reference lists of included papers and relevant meta-analyses, clinical trial registries (www.ClinicalTrials.gov and www.Controlled-Trials.com), conference proceedings published in Open Forum Infectious Diseases and PubMed Central, and the T2MR manufacturer’s website [Citation18]. Grey literature was eligible for inclusion in order to minimize the influence of publication bias [Citation19]. No language or date restrictions were applied. The final search was performed on 31 December 2020.
Table 1. Characteristics of the included studies
2.2. Study selection
Study selection was performed by two researchers with extensive systematic review experience (LM, DF). Study selection discrepancies between the reviewers were resolved by discussion and consensus. Titles and abstracts were screened to exclude review articles, cost-effectiveness studies, commentaries, letters, case reports, and obvious irrelevant studies. The full-text manuscripts of the remaining articles were retrieved and reviewed. Studies were excluded if the total sample size was less than 10 to minimize the influence of small-study bias, if the sample consisted exclusively of pediatric cases or the comparison consisted of discordant cases only [e.g. T2MR(+) vs. BC(-)], if nonclinical samples (i.e. spiked samples) were tested, or if outcomes of interest were not reported or not calculable. In studies where a kin relationship was identified (multiple publications reporting identical outcomes in the same or overlapping series of patients), the manuscript with the largest sample size was considered the primary report. Secondary reports were checked for complementary data on descriptions of study participants, design characteristics, or study outcomes.
2.3. Data extraction
We initially pilot-tested and refined a database to ensure consistency with outcomes reported in the literature. Data were independently extracted from eligible articles by the same two reviewers. Data extraction discrepancies between the reviewers were resolved by discussion and consensus. The standardized data extraction forms consisted of general manuscript information, study design features, patient characteristics, risk of bias using the Newcastle–Ottawa scale [Citation40], and outcome data. The risk of bias appraisal consisted of three study attributes: selection, comparability, and outcome. For selection, we evaluated representativeness of exposed cohort, selection of non-exposed cohort; ascertainment of exposure, and demonstration that outcome of interest was not present at the start of the study. For comparability, we evaluated study controls for baseline comorbidities and disease severity. For outcomes, we evaluated adequacy of outcome assessment, adequacy of follow-up duration, and adequacy of follow-up of cohorts. Studies were classified as high (1–3 stars), intermediate (4–5 stars), or low (6–9 stars) risk of bias accordingly.
2.4. Outcomes
The main outcomes of this review were time to detection, time to species identification, time to targeted therapy among T2MR positive cases, time to empirical therapy de-escalation among T2MR negative cases, length of intensive care unit (ICU) stay, length of hospitalization, and mortality. Time to detection was defined as the number of hours from the time of diagnostic testing to the time of a positive or negative diagnosis. Time to species identification was defined as the number of hours from the time of diagnostic testing to the time of specific pathogen identification among positive cases. Time to targeted therapy was defined as the number of hours from the time of diagnostic testing to the time of AAT among T2MR positive cases with species identification. Time to empirical therapy de-escalation was defined as the number of hours from the time of diagnostic testing to the time of broad-spectrum antimicrobial or antifungal discontinuation among T2MR negative cases. Length of hospitalization and length of ICU stay (both measured in days) were reported in standard fashion. Mortality was reported as death occurring in-hospital (preferentially) or within 30 days of admission. If results were incomplete or unclear, we contacted the corresponding author for additional data.
2.5. Data analysis
Continuous outcomes were reported as the mean difference (MD) between groups and 95% confidence interval where a negative value favored T2MR (indicating faster time with T2MR) and a positive value favored BC (indicating faster time with BC). Mortality was reported as a risk ratio (RR) and 95% confidence interval, where a RR <1 indicated lower risk with T2MR and a RR >1 indicated a higher risk with T2MR. Confidence intervals were adjusted for paired sampling when appropriate. Forest plots were used to illustrate individual study findings and pooled meta-analysis results. We used the I2 statistic to estimate the heterogeneity of effects among studies with values of ≤25%, 50%, and ≥75% representing low, moderate, and high inconsistency, respectively [Citation41]. When moderate or high heterogeneity was identified (I2 ≥50%), a random-effects model with inverse variance weighting was used; otherwise, a fixed-effect inverse variance model was used [Citation42]. Publication bias was qualitatively assessed by visual inspection of funnel plots where the effect size on the x-axis was plotted against its standard error on the y-axis, and quantitatively assessed with Harbord’s test for any outcome reported in at least 10 studies [Citation43]. In accordance with Cochrane Collaboration recommendations, we planned to perform a subgroup analysis on the association of study-level factors with any outcome reported in at least 10 studies. Independent variables included in the subgroup analysis were study design (prospective vs. retrospective), risk of bias (low vs. intermediate), geographical location (Europe vs. United States), suspected pathogen (Bacteremia vs. Candidemia), sample size (above median value vs. below median value), patient age (above median value vs. below median value), and female sex (above median value vs. below median value). In order to evaluate the influence of single-study effects, we performed a one-study removed sensitivity analysis in which we iteratively removed one study at a time to determine whether conclusions were significantly influenced by outlier studies. An individual study was considered an outlier if the effect size fell outside the 95% confidence interval of the pooled meta-analysis effect size. Statistical analyses were performed by a biostatistician author (LM) using Review Manager v5.3 (Cochrane Collaboration).
3. Results
3.1. Search results
The systematic literature search identified 215 publications, we reviewed 81 full-text papers and excluded 58 papers, most commonly those reporting no outcomes relevant to this meta-analysis or review articles. Ultimately, 14 primary studies linked to 9 secondary studies were included in the meta-analysis (Supplement Figure 2). Supplementary unpublished data were provided from the lead author of one study included in the systematic review [Citation45].
Figure 1. Hours to detection with T2 magnetic resonance (T2MR) vs. blood culture (BC). The mean difference in time to detection and the 95% confidence interval are plotted for each study. The size of the square is proportional to the study weight. The pooled mean difference among studies is denoted by the diamond apex. The 95% confidence interval is denoted by the diamond width. Pooled mean difference = −81 hours (p <0.001). Heterogeneity: I2>99% (p <0.001)
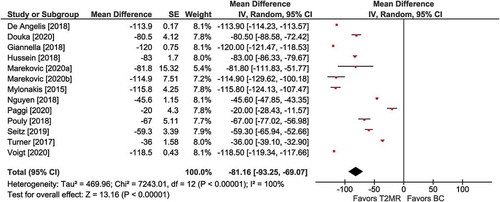
Figure 2. Hours to species identification with T2 magnetic resonance (T2MR) vs. blood culture (BC). The mean difference in time to species identification and the 95% confidence interval are plotted for each study. The size of the square is proportional to the study weight. The pooled mean difference among studies is denoted by the diamond apex. The 95% confidence interval is denoted by the diamond width. Pooled mean difference = −77 hours (p <0.001). Heterogeneity: I2Citation2>99% (p <0.001)
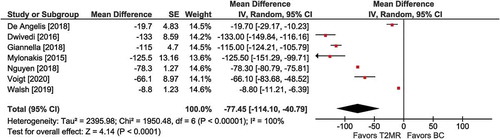
3.2. Study characteristics
Among the 14 primary studies comparing T2MR to BC, there was 1 randomized trial, 9 prospective observational studies, and 4 retrospective observational studies. T2MR was performed using the T2Bacteria panel in eight studies and the T2Candida panel in six studies. The mean patient age in each study ranged from 55 to 65 years, there was a slight male preponderance, and the most common clinical presentation was a sepsis syndrome, prompting BC collection to rule out bacteremia (). The risk of bias was low in eight studies and intermediate in six studies; no study was classified as high risk of bias ().
Table 2. Risk of bias assessed using the Newcastle–Ottawa score in included studies a.
3.3. Meta-analysis results
3.3.1. Time to detection
Among 13 comparisons, mean time to detection was faster with T2MR vs. BC (MD = −81 hours; 95% CI: −93 to −69; p <0.001). Significant heterogeneity (I2 >99%) in the magnitude of this benefit was observed among studies (). Publication bias was not evident by visual inspection of the funnel plot nor by Harbord’s test (p = 0.52) (Supplement Figure 3).
Figure 3. Hours to targeted therapy among T2MR positive cases with T2 magnetic resonance (T2MR) vs. blood culture (BC). The mean difference in time to targeted therapy and the 95% confidence interval are plotted for each study. The size of the square is proportional to the study weight. The pooled mean difference among studies is denoted by the diamond apex. The 95% confidence interval is denoted by the diamond width. Pooled mean difference = −42 hours (p <0.001). Heterogeneity: I2=99% (p <0.001)
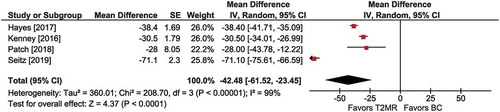
3.3.2. Time to species identification
Among seven comparisons, mean time to species identification was faster with T2MR vs. BC (MD = −77 hours; 95% CI: −114 to −41; p <0.001). Significant heterogeneity (I2 >99%) in the magnitude of the effect was observed (). Publication bias was not visually evident (Supplement Figure 4).
Figure 4. Hours to empirical therapy de-escalation among T2MR negative cases with T2 magnetic resonance (T2MR) vs. blood culture (BC). The mean difference in time to empirical therapy de-escalation and the 95% confidence interval are plotted for each study. The size of the square is proportional to the study weight. The pooled mean difference among studies is denoted by the diamond apex. The 95% confidence interval is denoted by the diamond width. Pooled mean difference = −7 hours (p = 0.02). Heterogeneity: I2=92% (p <0.001)
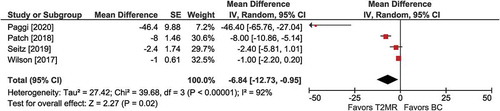
3.3.3. Time to targeted therapy in T2MR positive cases
Among four comparisons involving patients who tested positive on T2MR, mean time to receiving targeted antimicrobial therapy was faster with T2MR vs. BC (MD = −42 hours; 95% CI: −62 to −23; p <0.001). Significant heterogeneity (I2 = 99%) was identified (), and publication bias was not visually evident (Supplement Figure 5).
Figure 5. Intensive care unit (ICU) stay with T2 magnetic resonance (T2MR) vs. blood culture (BC). The mean difference in ICU days and the 95% confidence interval are plotted for each study. The size of the square is proportional to the study weight. The pooled mean difference among studies is denoted by the diamond apex. The 95% confidence interval is denoted by the diamond width. Pooled mean difference = −5.0 days (p =0.03). Heterogeneity: I2=21% (p =0.28)
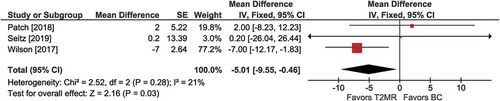
3.3.4. Time to empirical treatment de-escalation in T2MR negative cases
Among four comparisons involving patients who tested negative on T2MR, mean time to empirical therapy de-escalation was faster with T2MR vs. BC (MD =−7 hours; 95% CI: −13 to −1; p =0.02). Significant heterogeneity (I2 = 92%) among studies was detected (). Publication bias was not visually evident (Supplement Figure 6).
Figure 6. Hospital stay with T2 magnetic resonance (T2MR) vs. blood culture (BC). The mean difference in hospital days and the 95% confidence interval are plotted for each study. The size of the square is proportional to the study weight. The pooled mean difference among studies is denoted by the diamond apex. The 95% confidence interval is denoted by the diamond width. Pooled mean difference = −4.8 days (p =0.04). Heterogeneity: I2=0% (p =0.73)
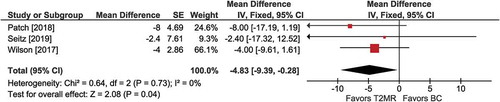
3.3.5. Length of intensive care stay
Among three comparisons, the mean length of ICU stay was shorter with T2MR vs. BC (MD =−5.0 days; 95% CI: −9.6 to −0.5; p =0.03) (). Heterogeneity was low (I2 = 21%) and publication bias was not evident (Supplement Figure 7).
Figure 7. Mortality with T2MR vs. blood cultures. The risk ratio for mortality and the 95% confidence interval are plotted for each study. The size of the square is proportional to the study weight. The pooled risk ratio among studies is denoted by the vertical line through the diamond apex. The 95% confidence interval is denoted by the diamond width. Pooled risk ratio = 1.02 (p =0.86). Heterogeneity: I2=0% (p =0.56)
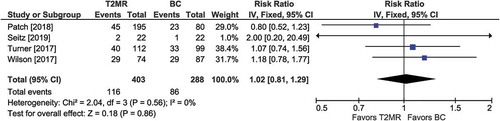
3.3.6. Length of hospital stay
Among three comparisons, the mean length of hospital stay was shorter with T2MR vs. BC (MD =−4.8 days; 95% CI: −9.4 to −0.3; p =0.04) (). Heterogeneity was low (I2 = 0%) and publication bias was not evident (Supplement Figure 8).
3.3.7. Mortality
Among four comparisons, the risk of mortality was comparable between T2MR vs. BC (28.8% vs. 29.9%; RR =1.02; 95% CI: 0.81 to 1.29; p =0.86) (). Heterogeneity was low (I2 = 0%) and publication bias was not evident (Supplement Figure 9).
3.4. Meta-analysis subgroup and sensitivity analysis
In a subgroup analysis of time to detection, we identified no patient- or study-related factors that influenced the treatment benefit of T2MR. Time to detection results statistically favored T2MR in every subgroup identified with the mean reduction ranging from 62 to 119 hours (). Subgroup analysis was not performed for other outcomes due to an insufficient number of studies. The meta-analysis results for each outcome were minimally influenced by any single study. This is evidenced by the demonstration of comparable results in the main analysis versus those identified following a sensitivity analysis utilizing iterative removal of one study at a time (Supplement Table 2).
Table 3. Subgroup analysis of time to detection with T2 magnetic resonance vs. blood culture
4. Discussion
In a systematic review of 14 controlled studies comparing T2MR to BC for the detection of bacterial and fungal BSI, there were several key findings. First, time to detection and time to species identification were approximately 3 days faster when using T2MR. Second, faster diagnosis times translated to meaningful changes in patient management where patients receiving a positive diagnosis transitioned to targeted microbial therapy 42 hours faster than those diagnosed with BC, and patients receiving a negative diagnosis were removed from unnecessary empirical therapy 7 hours faster vs. BC. Finally, diagnosis with T2MR safely facilitated ICU and hospital discharge 5 days faster than with BC. These results suggest that T2MR may be an important adjunct to BC that may allow accurate and timely management of patients with BSI. The management of infection in severe patients (e.g. critically ill, immunocompromised, elderly) is challenging. Antimicrobial therapy initiation should rely on two main considerations: the need for early antimicrobial coverage and the potential harm from inappropriate microbial use. Indeed, a critical window of time exists when delivery of antimicrobial therapy may alter the biologic response to widespread systemic inflammatory injury [Citation11]. On the other hand, rapid de-escalation should occur as soon as possible based on susceptibility of causative organisms if infection is excluded. The results of this meta-analysis demonstrated that a positive T2MR diagnosis facilitated patients receiving AAT nearly 2 days faster than those diagnosed with BC. Interestingly, among negative T2MR cases, the time to broad-spectrum antimicrobial de-escalation was only 7 hours faster with T2MR vs. BC with wide variability observed among studies. One plausible explanation for this finding is that clinicians may be reluctant to de-escalate from broad-spectrum antibiotics in light of a negative T2MR test since the panels do not currently detect all potentially causative pathogens. Currently, the T2Bacteria panel detects E. faecium, S. aureus, K. pneumoniae, P. aeruginosa, E. coli, and A. baumannii (the latter approved in Europe only). The T2Candida panel detects the five most common pathogenic Candida species that account for over 95% of candidemia at most hospitals, including C. albicans/C. tropicalis, C. glabrata/C. krusei, and C. parapsilosis [Citation14]. Thus, depending on the clinical circumstances, it may be prudent to await BC results following a negative T2MR result prior to empirical therapy discontinuation, although in the interim, the test results may allow the clinician to streamline the range of empirical therapy. A next-generation, high-throughput T2MR device is under development that may address this limitation since it will utilize a panel covering 99% of all BSIs by detecting >250 species of pan-Gram positive and pan-Gram negative bacteria, in addition to bloodborne antibiotic resistant threats. Intensive care unit stay and hospital stay were, on average, 5 days shorter in patients receiving a diagnosis with the T2MR vs. BC. Considering hospital costs for sepsis patients range from 2,000 USD to 5,000 USD per day depending on sepsis severity [Citation3], implementation of T2MR could theoretically reduce hospital costs by 10,000 USD to 25,000 USD per patient tested. This estimate is in line with the 27,000 USD per patient savings reported by Bilir et al. [Citation46] for T2Candida testing in candidemia patients. Using a decision analytic model, Shehadeh et al. [Citation47] reported that RDTs for septic shock were cost saving to hospitals if length of stay was at least 2 days shorter compared to BC. A supporting analysis by Zacharioudakis and colleagues [Citation48] determined that RDTs for severe sepsis/septic shock diagnosis were cost saving when the reduction in length of stay was at least 4 days relative to BC. Given the 5-day difference in ICU and hospital stay observed in the current meta-analysis, cost savings with T2MR are likely, particularly if the technology is used judiciously in populations with the highest pretest likelihood of BSI, such as more critically ill patients and/or immunocompromised patients. Blood cultures are still necessary in order to test for antimicrobial resistance/susceptibility and the cost-effectiveness of the T2MR assays will vary in different clinical settings and depend on factors, such as assay cost and performance, patient population, and local microbiology. Therefore, it may be helpful for future studies to develop algorithmic models that may serve as a clinical decision-making tool to identify the patient characteristics and timing of the test in the clinical workflow whereby the addition of T2MR may offer the ideal balance of patient benefit and cost savings. Antibiotic stewardship support or rapid notification of results is a consistent feature of studies that found statistically significant associations between RDT and improved outcomes [Citation49]. Thus, the clinical benefit of T2MR might be further improved by involving infectious disease consultants or antimicrobial stewardship members in patient selection and test interpretation, which has been demonstrated in studies with other RDTs [Citation50].
Several systematic reviews have assessed the clinical utility of RDTs as a class. In a systematic review without meta-analysis of 25 articles describing outcomes derived from 8 different RDT platforms, D’Onofrio et al. [Citation51] concluded that RDTs offered potential benefits regarding antimicrobial management, but insufficient data were available to draw conclusions regarding the length of hospital stay. In a systematic review with meta-analysis of 31 studies, Timbrook et al. [Citation50] concluded that molecular RDTs for diagnosis of BSIs were associated with shorter length of hospital stay (2.5 days) and decreased time to effective therapy (5 hours). To the author’s knowledge, we performed the first systematic review to investigate hospital resource utilization and clinical outcomes associated with the use of T2MR in patients with suspected BSI. We noted that time-to-detection metrics were commonly reported, changes to antimicrobial regimens based on test results were reported inconsistently, and clinical outcomes were reported less commonly among included studies. We propose that in order for RDTs to gain broader acceptance, future studies should emphasize clinically relevant outcomes comparing RDTs to BC while ensuring the study design adequately controls for potentially confounding factors.
This meta-analysis has certain limitations pertaining to the quality of studies available for analysis that may influence interpretation. First, diagnostic performance metrics, such as sensitivity and specificity, were not included. Previous studies have reported diagnostic performance with the T2Bacteria [Citation16] and T2Candida [Citation15] panels, but to the authors' knowledge, ours is the first to determine hospital resource utilization and clinical outcomes associated with the test panel results. Second, this review only included controlled studies comparing T2Dx to BC. However, many single-arm studies reporting experiences with the T2Dx have been published that were not included in our evidence synthesis, but that may provide useful data for determining how best to incorporate this diagnostic device into clinical workflows, including serial monitoring of patients in order to optimize treatment duration and improve prognosis assessment [Citation52]. Third, the time to detection with T2MR is known to vary depending on the number of test panels that are run simultaneously [Citation53]. However, even when running a full batch of test panels concurrently, time to detection results increase by only 3 to 4 hours and, therefore, the main conclusions of this review would likely be unchanged had these results been routinely reported. Third, patients presented with a wide range of diagnoses among the included studies and there was an insufficient number of studies available to explore sources of heterogeneity for most outcomes. Fourth, the T2Bacteria and T2Candida panels identify most, but not all, pathogens contributing to BSI. Therefore, BC is still required due to the risk of a false negative T2Dx diagnosis. Finally, while time to detection and positive species identification are objective endpoints, how these results influence antimicrobial prescribing patterns and patient care overall is uncertain. It is well known that there is considerable variation in the management of BSIs by infection specialists that may be dependent on numerous factors, such as patient presentation, physician experience, regional practice guidelines, local flora patterns, and presence of a hospital antimicrobial stewardship program [Citation54]. Such unmeasured factors may have contributed to the heterogeneity observed among some of the outcomes in this meta-analysis and their potential influence on patent management decisions warrants further study.
5. Conclusions
Utilization of T2MR for identification of bloodstream pathogens provides faster time to detection, faster transition to targeted microbial therapy, faster de-escalation of empirical therapy, shorter ICU and hospital stay, and with comparable mortality rate versus BC.
Reviewer Disclosures
Peer reviewers on this manuscript have no relevant financial or other relationships to disclose.
Declaration of interest
The authors have no relevant affiliations or financial involvement with any organization or entity with a financial interest in or financial conflict with the subject matter or materials discussed in the manuscript. This includes employment, consultancies, honoraria, stock ownership or options, expert testimony, grants or patents received or pending, or royalties.
Supplemental Material
Download MS Word (896.9 KB)Acknowledgments
The authors thank David Fay, Ph.D., for systematic review and data extraction assistance. We also thank Alexander Zoufaly, M.D., and Joshua Templeton, B.S.N., R.N., for providing a critical review of the manuscript.
2.6. Role of the funding source and data availability statement
No funding was received for this study. The manufacturer of the T2Dx device (T2 Biosystems) was not involved in any aspect of this review. The underlying data from this meta-analysis may be made available for research purposes upon receipt of a proposal to the corresponding author in accordance with the National Institutes of Health Data Sharing Policy [Citation44].
Supplementary material
Supplemental data for this article can be accessed here.
Additional information
Funding
References
- Singer M, Deutschman CS, Seymour CW, et al. The third international consensus definitions for sepsis and septic shock (sepsis-3). JAMA. 2016;315(8):801–810.
- Rudd KE, Johnson SC, Agesa KM, et al. Global, regional, and national sepsis incidence and mortality, 1990-2017: analysis for the global burden of disease study. Lancet. 2020;395(10219):200–211.
- Paoli CJ, Reynolds MA, Sinha M, et al. Epidemiology and costs of sepsis in the United States-An analysis based on timing of diagnosis and severity level. Crit Care Med. 2018;46(12):1889–1897.
- She RC, Alrabaa S, Lee SH, et al. Survey of physicians’ perspectives and knowledge about diagnostic tests for bloodstream infections. PLoS One. 2015;10(3):e0121493.
- Slimings C, Riley TV. Antibiotics and hospital-acquired Clostridium difficile infection: update of systematic review and meta-analysis. J Antimicrob Chemother. 2014;69(4):881–891.
- Bell BG, Schellevis F, Stobberingh E, et al. A systematic review and meta-analysis of the effects of antibiotic consumption on antibiotic resistance. BMC Infect Dis. 2014;14(1):13.
- Kumar A, Roberts D, Wood KE, et al. Duration of hypotension before initiation of effective antimicrobial therapy is the critical determinant of survival in human septic shock. Crit Care Med. 2006;34(6):1589–1596.
- Marquet K, Liesenborgs A, Bergs J, et al. Incidence and outcome of inappropriate in-hospital empiric antibiotics for severe infection: a systematic review and meta-analysis. Crit Care. 2015;19(1):63.
- Harbarth S, Garbino J, Pugin J, et al. Inappropriate initial antimicrobial therapy and its effect on survival in a clinical trial of immunomodulating therapy for severe sepsis. Am J Med. 2003;115(7):529–535.
- Lueangarun S, Leelarasamee A. Impact of inappropriate empiric antimicrobial therapy on mortality of septic patients with bacteremia: a retrospective study. Interdiscip Perspect Infect Dis. 2012;2012:765205.
- Kumar A, Haery C, Paladugu B, et al. The duration of hypotension before the initiation of antibiotic treatment is a critical determinant of survival in a murine model of Escherichia coli septic shock: association with serum lactate and inflammatory cytokine levels. J Infect Dis. 2006;193(2):251–258.
- Cendejas-Bueno E, Romero-Gomez MP, Mingorance J. The challenge of molecular diagnosis of bloodstream infections. World J Microbiol Biotechnol. 2019;35(4):65.
- Zacharioudakis IM, Zervou FN, Mylonakis E. T2 magnetic resonance assay: overview of available data and clinical implications. J Fungi (Basel). 2018;4(2):45.
- Pfaller MA, Wolk DM, Lowery TJ. T2MR and T2Candida: novel technology for the rapid diagnosis of candidemia and invasive candidiasis. Future Microbiol. 2016;11(1):103–117.
- Tang DL, Chen X, Zhu CG, et al. Pooled analysis of T2 Candida for rapid diagnosis of candidiasis. BMC Infect Dis. 2019;19(1):798.
- Nguyen MH, Clancy CJ, Pasculle AW, et al. Performance of the T2Bacteria panel for diagnosing bloodstream infections: a diagnostic accuracy study. Ann Intern Med. 2019;170(12):845–852.
- Liberati A, Altman DG, Tetzlaff J, et al. The PRISMA statement for reporting systematic reviews and meta-analyses of studies that evaluate health care interventions: explanation and elaboration. Ann Intern Med. 2009;151(4):W65–94.
- T2 Biosystems. Publications and Presentations. 2021; Accessed 2021 Jan 2. Available from: https://www.t2biosystems.com/news-resources/publications/
- Paez A. Gray literature: an important resource in systematic reviews. J Evid Based Med. 2017;10(3):233–240.
- De Angelis G, Posteraro B, De Carolis E, et al. T2Bacteria magnetic resonance assay for the rapid detection of ESKAPEc pathogens directly in whole blood. J Antimicrob Chemother. 2018;73:iv20–iv26.
- Douka E, Papachatzakis I, Vrettou C, et al. Evaluation of T2MR in a Greek university intensive care unit. Paper presented at: 30th ECCMID 2020; 2020. Paris, France.
- Giannella M, Paolucci M, Roncarati G, et al. Potential role of T2Candida in the management of empirical antifungal treatment in patients at high risk of candidaemia: a pilot single-centre study. J Antimicrob Chemother. 2018;73(10):2856–2859.
- Hayes JF, Turner OD, McCarty TP, et al. Influence of T2 Candida testing for rapid diagnosis of Candida infections on antifungal stewardship efforts at a large academic medical center: a retrospective single-center study. Paper presented at: IDWeek 2017; 2017. San Diego, CA.
- Turner OD, Hayes JF, McCarty TP, et al. Relationship of T2 Candida panel to disease severity, mortality and time to therapy in patients with candidemia. Paper presented at: IDWeek 2017; 2017. San Diego, CA.
- Marekovic I, Bosnjak Z, Plesko S, et al. T2Bacteria panel in diagnostics of sepsis: the first experience at the University Hospital Centre Zagreb. Paper presented at: 30th ECCMID 2020; 2020. Paris, France.
- Mylonakis E, Clancy CJ, Ostrosky-Zeichner L, et al. T2 magnetic resonance assay for the rapid diagnosis of candidemia in whole blood: a clinical trial. Clin Infect Dis. 2015;60(6):892–899.
- Nguyen MH, Pasculle W, Pappas PG, et al. Clinical performance of T2Bacteria among patients with bloodstream infections due to five common bacterial species. Paper presented at: ASM Microbe 2018; 2018. San Diego, CA.
- Paggi R, De Socio G, Belati A, et al. T2 magnetic resonance technology in the diagnosis of sepsis and clinical impact in patient management. Paper presented at: 30th ECCMID 2020; Paris, France.
- Patch ME, Weisz E, Cubillos A, et al. Impact of rapid, culture-independent diagnosis of candidaemia and invasive candidiasis in a community health system. J Antimicrob Chemother. 2018;73(suppl_4):iv27–iv30.
- Pouly O, Rouze A, Voisin B, et al. Accuracy of T2MR in diagnosing candidemia in critically ill patients: a prospective observational study. Intensive Care Med Exp. 2018;6(Suppl 2):40.
- Voigt C, Silbert S, Widen RH, et al. The T2Bacteria assay is a sensitive and rapid detector of bacteremia that can be initiated in the emergency department and has potential to favorably influence subsequent therapy. J Emerg Med. 2020;58(5):785–796.
- Guzman O, Estrada S, Clark J, et al. Blood culture-independent rapid diagnostic technology for improvement of time to bacterial species ID and earlier targeted antibiotic therapy. Paper presented at: MAD-ID 2019; 2019. Orlando, FL.
- Walsh TJ, Helfgott D, Van Besien K, et al. The T2Bacteria panel is a rapid detector of bacteremia and has potential to guide therapy in patients with hematological malignancies and hematopoietic stem cell transplantation (HSCT): a pilot study of non-culture molecular diagnostics. Paper presented at: 30th ECCMID 2019; 2019.
- Wilson NM, Alangaden G, Tibbetts RJ, et al. T2 Magnetic Resonance assay improves timely management of candidemia. Journal of Antimicrobial Stewardship. 2017;1:12–18.
- Chaudhry Z, Vahia A, Askar S, et al. Impact of T2 Candida panel on species specific anti-fungal de-escalation. Paper presented at: IDWeek 2018; 2018. San Francisco, CA.
- Chaudhry Z, Vahia A, Kathuria P, et al. Evaluation of the T2Candida panel as an antifungal stewardship tool in transplant and non-transplant patients at a tertiary care center. Paper presented at: IDWeek 2019; 2019. Washington, D.C.
- Dwivedi S, Ordaya E, Kezlarian B, et al. Novel T2 magnetic resonance assay compared to standard blood cultures for detection of candidemia. Paper presented at: IDWeek 2016; 2016. New Orleans, LA.
- Hussain N, Vahia A, Chaudhry Z, et al. Novel T2 Candida assay compared to blood cultures for detection of candidemia in transplant and non-transplant patients. Paper presented at: IDWeek 2018; 2018. San Francisco, CA.
- Kenney R, Dwivedi S, Kendall R, et al. Implementation of T2 magnetic resonance into the antimicrobial stewardship program improves management of candidemia at HFHS. Paper presented at: IDWeek 2016; 2016. New Orleans, LA.
- Stang A. Critical evaluation of the Newcastle-Ottawa scale for the assessment of the quality of nonrandomized studies in meta-analyses. Eur J Epidemiol. 2010;25(9):603–605.
- Higgins JP, Thompson SG, Deeks JJ, et al. Measuring inconsistency in meta-analyses. BMJ. 2003;327(7414):557–560.
- Lau J, Ioannidis JP, Schmid CH. Quantitative synthesis in systematic reviews. Ann Intern Med. 1997;127(9):820–826.
- Harbord RM, Egger M, Sterne JA. A modified test for small-study effects in meta-analyses of controlled trials with binary endpoints. Stat Med. 2006;25(20):3443–3457.
- National Library of Medicine. NIH data sharing policies. 2020; https://www.nlm.nih.gov/NIHbmic/nih_data_sharing_policies.html. Accessed 2020 Dec 19.
- Seitz T, Baumgartner S, Wenisch C, et al. Evaluation of the clinical impact of the T2MR for the diagnosis of bloodstream infections. Open Forum Infect Dis. 2019;6:S89.
- Bilir SP, Ferrufino CP, Pfaller MA, et al. The economic impact of rapid Candida species identification by T2Candida among high-risk patients. Future Microbiol. 2015;10(7):1133–1144.
- Shehadeh F, Zacharioudakis IM, Zervou FN, et al. Cost-effectiveness of rapid diagnostic assays that perform directly on blood samples for the diagnosis of septic shock. Diagn Microbiol Infect Dis. 2019;94(4):378–384.
- Zacharioudakis IM, Zervou FN, Shehadeh F, et al. Cost-effectiveness of molecular diagnostic assays for the therapy of severe sepsis and septic shock in the emergency department. PLoS One. 2019;14(5):e0217508.
- Barlam TF, Cosgrove SE, Abbo LM, et al. Implementing an antibiotic stewardship program: guidelines by the infectious diseases society of America and the Society for Healthcare Epidemiology of America. Clin Infect Dis. 2016;62(10):e51–77.
- Timbrook TT, Morton JB, McConeghy KW, et al. The effect of molecular rapid diagnostic testing on clinical outcomes in bloodstream infections: a systematic review and Meta-analysis. Clin Infect Dis. 2017;64(1):15–23.
- D’Onofrio V, Salimans L, Bedenic B, et al. The clinical impact of rapid molecular microbiological diagnostics for pathogen and resistance gene identification in patients with sepsis: a systematic review. Open Forum Infect Dis. 2020;7(10):ofaa352.
- Mylonakis E, Zacharioudakis IM, Clancy CJ, et al. Efficacy of T2 magnetic resonance assay in monitoring candidemia after initiation of antifungal therapy: the Serial Therapeutic and Antifungal Monitoring Protocol (STAMP) Trial. J Clin Microbiol. 2018;56(4):56.
- Roberts KM, Lapp HS, Barringer GE, et al. T2Bacteria panel: a rapid, culture-independent assay for identification of sepsis causative agents. Paper presented at: ASM Microbe 2018; 2018. Atlanta, GA.
- Diallo K, Thilly N, Luc A, et al. Management of bloodstream infections by infection specialists: an international ESCMID cross-sectional survey. Int J Antimicrob Agents. 2018;51(5):794–798.