ABSTRACT
Introduction
Pressure injuries (PIs) are a global health concern. Current PI care standards, including skin tissue assessments (STA) and health care professional (HCP) clinical judgment, diagnose visibly manifested PIs on the skin’s surface, i.e. after the damage has already occurred. However, objective assessment of early-stage, non-visible, pressure-induced tissue damage is clinically impossible within the current standard of care. The SEM Scanner is the first device authorized by the Food and Drug Administration (FDA) that addresses this unmet clinical need.
Areas covered
This review describes the novel sub-epidermal moisture (SEM) scanning technology of the device and summarizes the clinical safety and efficacy data that support the use of the scanner in routine PI care practice.
Expert opinion
The clinical strategy for developing the SEM Scanner is noteworthy. SEM technology using anatomy-specific data enables HCPs to provide early PI prevention interventions before visible signs of tissue damage develop while the damage is still reversible. When adopted into routine practice, the device identifies an increased risk of developing PIs 5 days (median) earlier than STA. FDA clearance was based on bench studies and data from three foundational trials that demonstrate the diagnostic accuracy of the device algorithm significantly exceeding clinical judgment (p < 0.001).
1. Introduction
A pressure injury (PI) – also known as a pressure ulcer- is localized damage to the skin and, or underlying tissue resulting from pressure or pressure combined with shear over a bony prominence [Citation1]. Pressure injuries are a frequently reported patient harm, despite an increasing global focus on PI prevention. These pressure-induced injuries are a significant burden to hospitals and severely reduce patient quality of life, with yearly treatment costs ranging from £1.4 – £2.1 billion in the UK to exceeding $26.8 Billion (USD) in the US [Citation2–9]. Looking back at how the COVID-19 pandemic has evolved and progressed globally, epidemiological data suggest an increase in PI prevalence within COVID-19 patients [Citation10–14]. In resource-limited critical care settings, severe respiratory disorders and multi-organ dysfunctions associated with emerging pathogens like Severe acute respiratory syndrome (SARS-CoV), avian influenza A (H5N1), the 2009 influenza A (H1N1) pandemic, Middle East respiratory syndrome coronavirus (MERS‐CoV), and avian influenza A (H7N9), increase the concomitant risk factors for PI/PU incidence [Citation10,Citation15]. The pathophysiological congruity between severe respiratory disorders, etiology, PIs due to prolonged prone positioning and medical device-related PIs is evident [Citation10–21].
The pathophysiology of PI development is such that early pressure-induced cell and tissue damage remains microscopic until it visibly manifests on the surface of the skin [Citation13,Citation22–26]. Prolonged pressure to the bony prominence, such as the sacrum and heels, result in deformation-induced cell death. Sustained cell and tissue deformations trigger an inflammatory response resulting in impaired perfusion, lymphatic drainage, localized edema, and ischemic damage [Citation13,Citation25,Citation26]. As this deformation and ensuing tissue damage continues, an increase in localized tissue edema (i.e. tissue moisture content), termed sub-epidermal moisture (SEM), can be detected. Fluctuations in SEM, an etiological biomarker, are indicative of early microscopic cell damage, despite the absence of visible signs on the surface of the skin.
Assessment of pressure injury risk, as well as describing the severity of any tissue damage, has been problematic on several levels. Firstly, risk assessment scales (RAS) and visual and tactile skin tissue assessments (STAs) in conjunction with clinical judgment are not intended to assess or identify existing PI damage [Citation27–30]. They are not anatomy specific and depend on common independent factors such as age, nutrition, activity, mobility, and skin status [Citation31–33]. Secondly, STAs are limited to visible and palpable changes to the skin alone. Not only are STAs subjective in nature, but the clinical judgment of the HCP is also subjective and influenced by the level of expertise of that particular HCP. Finally, the existing definitions of the severity of tissue damage utilize ambiguous and subjective terms, again dependent upon the clinical judgment of the HCP. For example, the international clinical practice guidelines (CPG 2014 & 2019) define a Stage I PI as ‘nonblanchable erythema’ and skin ‘reddening’ [Citation1,Citation34]. These definitions, however, do not describe early microscopic damage and deep tissue injury. In contrast, the international classification of diseases ICD-10 code, L89 defines a Stage I PI as ‘persistent focal edema,’ which seems more apt [Citation35,Citation36]. The clinical paradox and challenge in appropriately identifying PIs is more apparent when diagnosing PIs in darkly pigmented skin. In other words, detecting early microscopic tissue damage and timely prevention of PI damage before it is visible on the skin surface is clinically impossible within the current standard of PI care.
The 2019 Clinical Practice Guidelines acknowledge this gap in PI clinical practice. Specifically, recommendations 2.6 (strength of evidence B2; strength of recommendation ←→) and 2.7 (strength of evidence B2; strength of recommendation ↑) suggest sub-epidermal moisture measurement as an adjunct to routine PI care pathways [Citation1].
The SEM Scanner is the only FDA Authorized and CE Marked device for this clinical purpose. Fluctuations in SEM, detectable via SEM Scanner technology, notify HCPs of early incipient damage.
2. Overview of the market
The SEM Scanner is the first FDA-authorized pressure ulcer management tool. There are no direct competitors to the SEM Scanner technology with the intended use as an adjunct to the standard of care when assessing the heels and sacrum of patients at increased risk for PIs. Impedance devices (e.g. Nova® Dermal Phase Meter, MoistureMeter D®) have examined device-dependent absolute SEM measures as an indicator of pressure-induced damage [Citation37]. These devices have not been approved for patient use and are available for research only. Thermography and ultrasound devices have been explored for their clinical utility in the detection and diagnosis of PIs. The validity and diagnostic accuracy of these devices are, however, not well established [Citation38]. Ultrasound technology is limited to detecting visible damage alone and requires comprehensive training for image-based clinical interpretation, while studies relating to Thermography require more consistent and quality research data [Citation37]. Other medical devices, such as pressure mapping systems, mattresses, and patient movement monitoring devices, assess immobility but increase the risk of medical device-related PI development. A significant limitation to these devices is that they do not provide an indication of the presence or absence of skin and tissue damage. Furthermore, other imaging-based technologies and computational models, including MRI scanning techniques and finite element (FE) models, lack validated studies in assessing early skin damage [Citation39].
3. The SEM Scanner
Logically, the early detection of pressure induced tissue damage (i.e. before it is manifest at the skin level) should provide the opportunity for the HCP to intervene early and thereby potentially reduce or even reverse tissue damage [Citation13,Citation40]. The International Clinical Practice Guidelines (CPG 2019) refer to this as the damage threshold [Citation1]. This guideline recognizes the role of localized inflammatory edema/sub-epidermal moisture (SEM) as ‘one of the earliest signs of cell death in pressure injuries’ – a profound change and shift in the paradigm. However, the current definitions of a Stage I PI include contradictory characteristics (firm/soft, warm/cold, red/not red if dark skin toned) of skin and tissue status, the presence of visual discoloration of the skin and tissue, and the subjective interpretation by the HCP [Citation1]. These conditions make a precise diagnosis, or even a differential diagnosis, highly challenging. While early detection of pressure induced tissue damage is ultimately desirable and potentially amenable to site-specific prevention interventions, the ability to do so has been limited by the definition of a Stage 1 and by available assessment tools [Citation22]. Until now, the differential diagnosis of subclinical and clinical indications of pressure-induced tissue damage was not possible.
3.1. How the device works
The SEM Scanner is an FDA authorized (CE class IIa) wireless, noninvasive, hand-held device for identifying changes in the Biocapacitance of skin and tissue [Citation41]. Intended for use at the bedside by HCPs, the device technology compares multiple local measurements to determine the difference in SEM values between potentially damaged and nearby healthy tissue. The device measures the biocapacitance of the local skin and subdermal tissues under its sensor by assessing SEM fluctuations. Biocapacitance is a bioelectrical property of tissue that varies with the amount of interstitial moisture content [Citation40]. A large self-biocapacitance of a tissue region indicates that this tissue region can hold more electric charge at a given voltage than a different region with a low self-biocapacitance. For tissues, as with many dielectric materials, the biocapacitance is independent of the electrical potential applied by the SEM sensor. The biocapacitance of tissues, however, is variable and highly sensitive to the interstitial water content of the tissue. The dielectric constant of water (approximately 80) is 10 to 20-times greater than that of all solid tissue components, e.g. collagen and elastin [Citation42,Citation43]. In a specific anatomical region, with in a given anatomical configuration, the device reading of biocapacitance will be predominantly affected by the dielectric tissue properties, which are, in turn, highly sensitive to the amount of water in the examined tissues. Accordingly, any inflammation-related increase in the vascular and, or lymphatic walls’ permeability will almost immediately be measurable due to its impact on the affected tissues’ effective dielectric property. Hence, there is a rapid and dramatic increase in tissue biocapacitance even if the inflammatory response has just been initiated and despite visible (clinical) signs [Citation40].
The device makes a direct steady-state measurement of its sensor’s capacitance, which is affected by the equivalent dielectric constant of the material (i.e. the layered tissue structures) within the electric field between the sensor electrodes to a depth of up to 0.15 inches (4 millimeters). The device then converts the biocapacitance from SI units to an SEM value ranging from 1.0 to 4.5 (± 0.2). Two values are displayed on the device’s screen: an individual value for every single scan and the SEM Delta (∆SEM) score. The delta value is a measure of the difference in the SEM values between potentially damaged tissue and nearby healthy tissue [Citation44,Citation45]. Calculation of a ‘delta’ value compares multiple measurements within a specific anatomy, some of which will be healthy tissue, compensates for systemic changes, overcomes the limitation of inter and intra-patient variability (such as changes in the patient’s hydration status), and provides a measure of tissue health condition [Citation45]. Clinical studies determined a threshold delta value of 0.6 as the cutoff so that a:
Δ < 0.6 indicates a lower risk for a PI at the anatomic site, while; a,
Δ ≥ 0.6 indicates an increased risk for PI at the anatomic site.
The delta value indicates compromised tissue that is likely to develop into a PI if an intervention is not implemented. As an objective measure, delta values overcome the limitations of the visual skin assessment and HCP variance in skill and technique [Citation40,Citation44–47]. Consequently, when an elevated delta value is obtained, anatomically specific interventions can be implemented for a potential reversal of tissue damage before manifesting at the skin’s surface. Compared with visual and tactile skin assessments, the device supports HCPs to identify specific anatomical areas at increased risk of PI development five days (median) earlier than visual skin assessment [Citation44,Citation45].
SEM Scanner values are recorded as an integral component of patients’ records. They remain available in the medical records following the transfer of patients between care settings, between admission, daily during the episode of care, and at discharge. The SEM Scanner 200 contains an integrated circular coaxial sensor, and a single (action) button is used to turn the device on, reset it, and turn the device off ()). The Provizio® SEM Scanner system ()) is an enhancement of the SEM 200. It is available in three variants: (a) a separately supplied single-use, non-sterile, single-patient use sensor with manual entry of patient ID, (b) a single-patient use sensor with an integrated barcode scanner for patient ID, and (c) a fixed-head sensor with an integrated barcode scanner designed for research purposes.
The Provizio® SEM Scanner and the SEM Scanner 200 are identical in their technology, intended use, and clinical interpretation [Citation48]. Hereafter, in this review, the use of ‘SEM Scanner’ will define the SEM Scanner series (Provizio® SEM Scanner and the SEM Scanner 200).
3.2. Cost-effectiveness
Cost implications of deploying the SEM Scanner in routine clinical care pathways are published in the public domain. In the NHS care setting, Gefen et al. (2020) demonstrated a cost-saving consequence when PI incidence reduction, including downstream cost-savings resulting from reduced PI treatment costs, were included in the analyses [Citation49]. Other analyses show that deploying the SEM Scanner is a dominant quality intervention in routine clinical practice. Deploying the SEM Scanner as an adjunct to routine PI care pathways results in significant PI incidence reductions. In multiple care settings, PI-related hospitalizations and subsequent high PI treatment costs are avoided resulting in the SEM Scanner being clinically more effective and less expensive than the current standard of care [Citation50].
3.3. Performance standards
The SEM Scanner was reviewed by the Center for Devices and Radiological Health (CDRH) of the Food and Drug Administration (FDA). The scanner was granted de novo FDA- authorization in 2018 as a class I prescription device under 1CFR Part 801.109. The FDA established a new class of devices under the generic name ‘pressure ulcer management tools’ (QEF) [Citation41]. The SEM Scanner is the first FDA-authorized class I device intended for patients at risk of developing PIs. As a CE-marked device (2013), the device meets the Council Directive 93/42/EEC provisions on Medical Devices for Medical Devices (EU-MDD), the European Union Medical Device Regulation of 2017 (EU-MDR), and its relevant transposition into national laws of the member states into which the device is placed.
The device was subject to a series of bench tests, hardware/software validation-verification studies, and clinical studies which demonstrated that the device: (a) reliably evaluated tissue health, (b) provided reasonable assurance of the safety and effectiveness of the device, (c) demonstrated meeting its intended requirements including usability and human factors, packaging, shipping, sterilization, shelf life, cleaning, disinfection, biostability, biocompatibility, electrical safety, and electromagnetic compatibility.
3.4. Safety and complications
The SEM Scanner is a non-significant risk, noninvasive device. A search of the FDA recall database with the term ‘capacitance’ returned one result between 1976 and 2020, the Freedom EVO, an open automation platform product for general laboratory use, that does not bear any resemblance either in principle or practice to the SEM Scanner.
A search of the FDA adverse databases (MAUDE, MDR, and MedSun) and the EU Medical and Healthcare Products Regulatory Agency Database (EU MHRA) with search dates from 1976 to February 2020 did not return any adverse events or medical device alerts for this device.
The device has been evaluated for biocompatibility and biological risks to ensure further patient safety [Citation52]. The device is categorized as a surface medical device with contact to intact skin only with a limited duration category. The only components potentially in contact with the patient’s body are the sensor assembly and the outer enclosure frame of the SEM Scanner. Biocompatibility tests showed reactivity grades of zero (0), indicating that the materials are not cytotoxic. Sensitization test results of this study showed no evidence of delayed dermal contact sensitization. Irritation tests, including Intracutaneous reactivity, demonstrated no irritation response and irritation potential in using the device on the skin, eye, and mucous membrane.
The device is designated with a class II classification (ISO 60,601: Product Safety Standards for Medical Devices Verification) as a medical device with reinforced insulation to safeguard against the possibility of the device going live during a fault (). Devices under this classification intend to protect nonprofessional users from electrical shock by approaching all home settings as if they do not have protective earth-ground wiring.
Figure 2. Wall to patient/user and the isolation barrier enabling reinforced insulation between the SEM Scanner and the patient
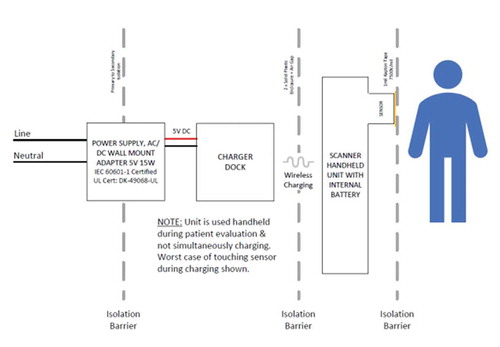
These results demonstrate that the SEM Scanner is safe. No adverse events to patients or users have been reported from SEM Scanner use during clinical studies or post-commercial use in routine clinical practice.
3.5. Contraindications
The device is not intended to be used on broken skin and is intended for use in the adult population only.
4. Clinical profile and post-marketing findings
The SEM Scanner provides significant, measurable clinical benefits to PI prevention that urgently needs objective data. There is currently no other clinically reliable tool to assess early signs of a developing PI. Clinical data of the SEM Scanner is represented in a pyramid of evidence format () to demonstrate the incremental evidence which shows that the device: 1) works as claimed; 2) has clinical utility, such that the device is equal to or better than the current standard of PI care and, ultimately, 3) that should be the standard of care device supporting nurses in their prevention of preventable PIs without any new, additional interventions or staff members. A comprehensive bibliography of the device provides details of peer-reviewed publications, oral conference presentations, or conference posters, all of which fit into one or more levels of the pyramid (https://sem-scanner.com/evidence/). Specifically, fourteen (14) publications map to the bottom of the pyramid reviewing SEM and its associated biomarkers, while four (4) relate to the ‘increasing utility’ layers [Citation44–46,Citation51]. The association of SEM as a biomarker in the development and progression of PIs is extensively reported in literature [Citation23,Citation53–57]. Particularly, ongoing clinical studies and published work by the Bates-Jensen group at the University of California in Los Angeles indicate the clinical significance of SEM in the early detection of PIs [Citation23,Citation24,Citation55,Citation58,Citation59]. Collectively, these studies contributed to SEM being identified as an important biomarker of PI etiology [Citation1,Citation23,Citation24,Citation37,Citation47,Citation53,Citation54,Citation60–65].
Figure 3. Claims-Data-Value Framework- Evidence pyramid in the development of SEM Scanner technology

4.1. Data from Pre-FDA clearance studies
A series of bench-tests, verification, and validation studies were performed to support SEM Scanner technology development. Bench testing of the SEM Scanner showed that its measurement field is responsive to materials introduced to the SEM Scanner field up to a depth of ~4-6 mm from the sensor [Citation40]. In their recent publication, Ross and Gefen (2019) comprehensively describe the underlying principles and measurement procedures of the SEM Scanner technology [Citation40].
The SEM Scanner has been formally evaluated in three foundational clinical studies in the United States and the United Kingdom, totaling 395 participants. Each study was designed with different objectives, as described below. As such, the sample sizes should be considered in the context of the specific study.
•The first was to evaluate the repeatability of the SEM Scanner readings between operators and multiple devices;
•The second was to evaluate the sensitivity, specificity, and clinical utility of spatial variation in SEM readings between healthy and pressure-damaged skin tissue;
•The third was to demonstrate in a prospective, longitudinal multi-site study within the clinical setting that measured changes in the SEM biomarker by the device are associated with the later manifestation of a PI (Stage 1, Stage 2, Deep Tissue Injury). Compared to the reference standard of clinical skin and tissue assessment, the study also demonstrated that the scanner notifies HCPs of early tissue damage prior to PI manifesting at the skin’s surface.
4.1.1. Inter-operator and inter-device agreement study [Citation51]
This study included thirty-one (31) volunteers free of PIs or broken skin at the sternum, sacrum, and heels. Three (3) operators utilized three (3) devices each to collect readings from four anatomical sites on each participant for a total of 108 readings per participant, resulting in more than 3000 SEM assessments over the course of the study. The intraclass correlation (ICC) for both inter-operator and inter-device reliability exceeded 0.80 at all anatomical sites assessed. These data demonstrated SEM Scanner technology as a repeatable and reliable tool between multiple operators and multiple devices.
4.1.2. Sensitivity, specificity, and clinical utility study [Citation45]
In this study (Study Protocol SEM200-003 & SEM200-004), the SEM Scanner was used to assess sacral and heel regions between PI damaged and healthy tissue. This study enrolled 125 participants with diagnosed PIs involving 129 wounds (e.g. Stage I/II and deep tissue injury), as well as 50 study participants unaffected by PIs (). Data from the study resulted in the development of two algorithms (A & B) with a range of cutoff thresholds indicating a sensitivity of 87–82% and a specificity of 88–51% at the conservative cutoff of SEM delta of equal to or greater than 0.6. Receiver operating characteristic (ROC) curves, representing the diagnostic ability of the test of SEM in differentiating between pressure-damaged and healthy tissues, computed areas-under-the-curve (AUC) of 0.7809–0.9181 (95% CI 0.7221–0.8817, 0.8397–0.9545), <0.0001). Both algorithms statistically significantly exceeded clinical judgment (). While algorithm A reported higher sensitivity and specificity, algorithm B, which was easier to implement in the real world, was eventually used in the scanner’s commercial development.
Figure 4. Receiver operating characteristic curve for performance of algorithms A and B relative to the gold standard of skin and tissue assessment in combination with clinical judgment. Receiver operating characteristic curve illustrating diagnostic sensitivity and specificity of the SEM Scanner algorithms in early detection of deep and early stage pressure-induced injury. AUC, area under the curve. CI, confidence Intervals. Figure adapted from Gershon et al, 2021 .[Citation45]
![Figure 4. Receiver operating characteristic curve for performance of algorithms A and B relative to the gold standard of skin and tissue assessment in combination with clinical judgment. Receiver operating characteristic curve illustrating diagnostic sensitivity and specificity of the SEM Scanner algorithms in early detection of deep and early stage pressure-induced injury. AUC, area under the curve. CI, confidence Intervals. Figure adapted from Gershon et al, 2021 .[Citation45]](/cms/asset/e78f8ae1-b05b-4a6b-9446-efd25df292b5/ierd_a_1960505_f0004_oc.jpg)
Table 1. Foundational studies of the SEM Scanner evaluated by the Food and Drug Administration (FDA) prior to regulatory De Novo approval
4.1.3. A blinded clinical study using a subepidermal moisture biocapacitance measurement device for early detection of pressure injuries [Citation44]
This study (Study protocol SEM200-008) enrolled 189 participants, of which 182 were included in the intent-to-treat population, accounting for 437 evaluable anatomical locations. This multi-site clinical study was designed to demonstrate that the SEM Scanner could detect PIs in patients before PIs were diagnosed through clinical judgment alone (‘diagnose PI before clinical judgment’) and the average number of days of early detection (‘time to detection’).
Sensitivity was 87.5% (95% CI: 74.8%-95.3%) and specificity 32.9% (95% CI: 28.3%-37.8%). Area Under the Receiver Operating Characteristic Curve (AUC) was 0.6713 (95% CI 0.5969–0.7457, p < 0.001) (). SEM changes were observed 4.7 (± 2.4 days) earlier than diagnosis of a PI via STA alone. Aggregate SEM sensitivity and specificity and 67.13% AUC exceeded that of clinical judgment alone.
Figure 5. Receiver operating characteristic curve for performance of the SEM Scanner relative to the gold standard of skin and tissue assessment in combination with clinical judgment. Receiver operating characteristic curve illustrating diagnostic sensitivity and specificity of the SEM Scanner in early detection of deep and early stage pressure-induced injury. AUC, area under the curve. CI, confidence Intervals. Figure reprinted with permissions from Okonkwo et al, 2020 .[Citation44]
![Figure 5. Receiver operating characteristic curve for performance of the SEM Scanner relative to the gold standard of skin and tissue assessment in combination with clinical judgment. Receiver operating characteristic curve illustrating diagnostic sensitivity and specificity of the SEM Scanner in early detection of deep and early stage pressure-induced injury. AUC, area under the curve. CI, confidence Intervals. Figure reprinted with permissions from Okonkwo et al, 2020 .[Citation44]](/cms/asset/bc44ed15-5536-4ed4-a50d-7451e7df0856/ierd_a_1960505_f0005_oc.jpg)
In summary, these data demonstrated that the SEM Scanner is safe and effective for its proposed indication for use. The likelihood of risk to patients, as assessed by FDA, is low or non-significant. Together with bench studies, these clinical data provided a strong basis for the FDA to grant the SEM Scanner De Novo authorization [Citation41].
4.2. Data from Post-FDA approval studies
Post-FDA clearance, multiple studies have demonstrated the scientific principles of observability and repeatability in adopting the SEM Scanner in routine clinical practice to achieve sustainable PI prevention at scale. This section summarizes the outputs of these initiatives, including collaborations with health systems, providers of care, and investigations initiated by independent research groups. In addition, real-world evidence from a pragmatic Pressure Injury/Ulcer Reduction Programm (PURP) is presented, highlighting the SEM Scanner’s increasing clinical utility and impact in reducing PI incidence rates in different care settings, including Acute Care, Post-Acute Care, Community Care, and Palliative Care.
4.2.1. Investigator-Initiated studies
Raizman et al. (2018) conducted a consecutive series study of 284 patients to evaluate the SEM Scanner’s clinical utility. The study was conducted in two phases to include evaluating the Hawthorne effect – decrease in PI incidence because of changes in healthcare practitioner behavior, due to being involved in the study and being observed, in contrast to the clinical utility of the SEM Scanner [Citation66]. During the first phase, patients were provided standard-of-care risk assessment and intervention and were assessed with the scanner. The resulting SEM Scanner results were not used to determine the interventions. During phase 2, patients were assessed with both the standard-of-care risk assessment and the SEM Scanner. However, interventions were based on SEM Scanner indications. During phase 1, 13.5% (12 of 89) developed pressure injuries. Of these injuries developed, four were Stage I, 6 were Stage II, 1 was Stage III, and 1 was a deep tissue injury. Only 1% (2 of 195) of the phase 2 patients developed pressure injuries when an intervention was based on SEM Scanner readings. The authors note that the patients in phase 2 were more incontinent, less mobile, and had longer lengths of stay than those in phase one. The authors concluded that using the SEM Scanner to influence clinical interventions resulted in a 93% decrease in hospital-acquired pressure injuries. The Hawthorne effect did not affect the improvements in PI incidence [Citation66].
In a prospective cohort study comparing the consistency between SEM Scanner and ultrasound examinations of deep tissue injury (DTI), the authors reported that both ultrasound and SEM Scanner results were similar when a DTI existed. However, they also observed higher reliability and better interobserver agreement with the SEM scanner when compared to interpreting ultrasound images which require expertise and comprehensive training. In an evolving case, one patient developed a heel sDTI during the study, and SEM detected the lesion two days before VSA and three days before the ultrasound [Citation46]. Similarly, in a systematic review of bedside accessible technologies (i.e. ultrasound, thermography, SEM, reflectance spectrometry, and Laser Doppler), the authors reported that the use of SEM measurement for the early identification of PI was supported, while other technologies needed further research. In addition, the authors highlighted that this technology might be particularly beneficial to provide equitable care and reduce outcome disparities in patients of color [Citation37].
A real-world case series of 35 patients followed up over two months on a single medical-surgical unit was conducted to evaluate the impact of the SEM Scanner use for early PI detection and their clinical outcomes. While several patients were assessed on admission as ‘at-risk’ by the Waterlow risk assessment tool, their SEM readings indicated no damage was present. However, during their stay, all patients developed a SEM Scanner delta value ≥0.6. Yet, none of the 35 patients went on to develop a new PI, suggesting successful PI prevention due to early interventions. Smith (2019) concluded that daily scanning proved to be a more effective method of assessing for damage objectively as opposed to using visual assessment alone [Citation67].
In a pilot study in which the SEM Scanner was integrated into the everyday care of patients in the community, Mersey Care Foundation Trust (MCFT), UK reported improved clinical decision-making, early implementation of SoC interventions as a direct result of SEM delta readings, and a 26.7% reduction in community-acquired PI incidence. As a result of this pilot, MCFT was able to directly correlate implementing SEM technology to their PI incidence reduction objectives and successfully adopted the SEM Scanner as part of routine clinical practice and patient assessment [Citation68].
The Royal College of Surgeons Ireland (RCSI) University of Medicine and Health Sciences, School of Nursing and Midwifery/Skin Wounds and Trauma (SWaT) Research Center led ten (10) research projects exploring the value of SEM measurement using the SEM Scanner across several different settings (seven acute, two residential, and one community). Seven of these studies are now complete (4 acute, two residential, one community). Several clinically significant findings emerged from these data.
SEM delta elevations correspond to immobility in patients [Citation69]. In general and surgical hospital patients, the SEM scanner enables identifying anatomical areas that are adversely affected by pressure and shear during immobility [Citation70]. Implementing the SEM Scanner as an adjunct to daily PI care has a ‘two-fold’ benefit. The first is that the SEM Scanner enables early identification of tissue damage and enables practitioners to provide improved anatomically specific interventions [Citation71]. The second is that this early and appropriate intervention of non-visible pressure-induced tissue damage prevents sub-cellular damage from progressing and manifesting itself on the surface of the skin as a diagnosed visible Stage I PI [Citation62]. Based on these findings, further research is being conducted to investigate the outcomes of these targeted interventions at specific anatomies, in addition to observational studies comparing the SEM scanner to other current measures of PI progression such as ultrasound, temperature, and pain.
These data suggest that identifying elevated SEM deltas can trigger the ramping up of clinically relevant care bundle interventions. This bundled approach to PI prevention could also potentially lead to immediate cost savings relating to dressing and antibiotic costs associated with PI treatment and downstream primary and secondary costs related to prolonged length of stay and the need for further community care interventions [Citation5,Citation49,Citation70,Citation72].
4.2.2. Device-generated evidence from everyday practice
In daily clinical practice, implementing the SEM Scanner as an adjunct to clinical judgment has resulted in consistent PI reductions in multiple health care settings. The Pressure Injury Reduction Program (PURP), introduced by the device manufacturers (Bruin Biometrics, LLC), provides an opportunity for health systems and care providers to conduct real-world, pragmatic evaluations of the device. This approach to real-world implementation of the device was structured to align with the General Data P279/279 [Citation73], clinical data practices described in the Food & Drug Administration’s 2017 Guidance on the use of Real World Evidence [Citation74], and NICE guidelines on Real World Evidence from the National Institute of Health and Care Excellence (DSU Technical Support Document 17, 2015). The program aimed at measuring the impact of the SEM Scanner across all care settings, driving significant outcomes toward eliminating avoidable PI incidence rates in individual departments or care units. The program evaluates the impact of the SEM Scanner on the rate of acquired pressure injuries and the ability to incorporate use of the SEM Scanner into the clinical workflow over a period of one to twelve months.
In daily PI care practice, a total of 2,439 patients across 34 care facilities (31 acute care, one palliative care, one community care) have been assessed using the SEM Scanner [Citation75]. In acute care sites, implementing the SEM Scanner into routine clinical practice has resulted in an overall 90.5% reduction compared to prior incidence rates (). This pragmatic approach is structured around standardized protocols and training workshops that provide easy adoption of the SEM Scanner into existing care pathways in several facilities. Except for the addition of the SEM Scanner, no changes are made to the existing SoC [Citation71].
Figure 6. Pressure injury (PI) incidence reduction rates using the SEM Scanner as an adjunct in daily PI care practice
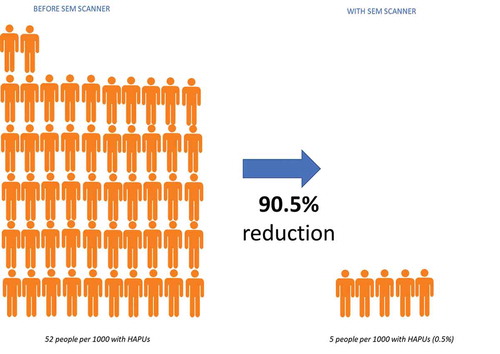
In acute care settings (n = 31):
• 74% (23/31) of the hospitals had zero (0) acquired PIs during the evaluations: a 100% reduction rate,
• 87% (27/31) of the hospitals had a reduction in PIs of >80%,
• 94% (29/31) of the hospitals had a PI incidence rate of 3% or less.
Intervention data was collected for 1830 patients across 27 acute care PURPS. Additional interventions because of device-generated data were provided to 72% patients (n = 1,323/1,830). Clinical decision making was recorded in 1,591 patients across 22 acute care settings. In 69% patients (n = 1,091/1,591), the SEM Scanner readings influenced the nurse’s decision in increasing SoC interventions. In these 1,091 patients, mobilization or turning was increased in 79% of patients (n = 862/1,091), and a specialist surface or mattress was introduced in 47% of patients (n = 513/1,091). Heel support or elevation of heels was introduced in 71% of patients (n = 775/1,091), and a prophylactic dressing or barrier cream was introduced in 66% of patients (n = 719/1,091). One palliative care setting reported a 47% HAPI/PU reduction from prior incidence using the SEM Scanner, while a 26.7% HAPI/PU reduction was recorded in a community care setting. These results demonstrate that implementing the SEM Scanner in routine clinical practice as an adjunct to the current standard of PI care can result in up to a 100% decrease in acquired PIs.
5. Regulatory status
The SEM Scanner has been assessed and certified with a CE mark as meeting the requirements of Directive 93/42/EEC on Medical Devices, Annex II (excluding Section 4) on November 11th, 2013. In the US, the device was approved as a Class I de novo device (DEN170021) on December 20th, 2018, as a ‘Pressure Ulcer Management Tool’ with a product code ‘QEF’ [Citation41]. The device is a prescription-only device intended for patients at risk for developing PIs. Current approvals and new commercialization applications in progress are detailed in .
Table 2. Regulatory approval of the SEM Scanner series – SEM Scanner 200 and the Provizio® SEM Scanner
6. Limitations
The clinical utility of this device and its SEM Scanning technology, profiled herein, should be considered in the light of some limitations. First, Okonwo et al. (2020) reported low specificity (32.9%) in using the device in their study [Citation44]. The epistemological problem in validating the sensitivity and specificity of the test of SEM is the lack of a direct comparator to assess changes in sub-epidermal moisture. At the time of measurement, the device technology will report a positive finding (Δ ≥ 0.6) based on subclinical changes to SEM at the anatomy, indicative of early pressure induced damage. In contrast, the current gold standard, a confirmatory diagnosis via skin tissue assessments, is based on the appearance of a visually identifiable PI – damage that has already occurred. Additionally, while withholding preventive measures is ethically unacceptable, intervention strategies are effective in reducing PI incidence [Citation76] resulting in apparent false positives. Despite this limitation, the diagnostic accuracy of the test of SEM (AUC 67.13%) far exceeds that of clinical judgment () [Citation28,Citation44,Citation45]. In everyday practice, the practical risk of high false positives, at best, is a potential overuse of preventive strategies in patients who are already deemed at risk for developing PIs. The alternative is a time and cost-intensive PI treatment strategy which adversely affects patients’ quality of life. Treatments can cost up to 5 times more than the cost of prevention [Citation77].
Second, although the device technology is supported by an abundance of clinical evidence, including real-world data from everyday practice, there are no published randomized clinical trials. Future research should consider a controlled randomized trial to comprehensively evaluate the clinical benefits of the device.
Third, the device and SEM scanning technology have progressed from proof-of-concept to adoption into everyday practice. Health economics data published in the literature were based on probabilistic models. Further work should focus on empirical health economic evaluations.
Finally, future generations of the device series should consider expanding indications for use beyond the sacrum and heels and validate appropriate design changes to allow accurate measurement of specific anatomies such as the bridge of the nose, which is a common site for medical device-related PIs.
7. Conclusion
The current standard of PI care, skin assessments supplemented by clinical judgment, is inadequate in diagnosing early-stage pressure-induced tissue damage. Visual and tactile confirmation of skin redness, i.e. confirmed diagnosis of a Stage I PI, indicates that damage has already occurred and has manifested onto the skin surface. Healthcare practitioners have no objective, accurate, and anatomically specific options to identify an increased risk of PI, specifically early-stage, non-visible, pressure-induced cell and tissue damage. The SEM Scanner informs HCPs of this incipient damage which, until now, has been subclinical. This early insight into deep tissue viability can be used to direct anatomically specific, targeted interventions and gives the HCPs the ability to monitor the response at an anatomical location over time.
Prevention using the SEM Scanner is a dominant quality intervention which subordinates all others in a PI care pathway. Assessed with a low to no risk safety profile, the SEM Scanner objectively alerts HCPs to specific anatomical areas of a patient’s body at risk for deep and early-stage pressure-induced injuries, which allows for preventive interventions before visible damage manifests at the skin surface. When used as intended, the SEM Scanner’s performance augments clinical decision making and is statistically significantly better than clinical judgment alone in assessing PI risk at specific body sites.
8. Expert opinion
The SEM Scanner and the diagnostic value of SEM scanning technology is backed by extensive clinical trials with more than 30 peer reviewed publications recognizing sub-epidermal moisture as an early biomarker of impending pressure-induced skin and tissue damage. The scientific rationale that stems from foundational studies of the SEM Scanner is comprehensive and complete, validating the test of SEM as a reliable, repeatable, and objective tool in the early detection of non-visible pressure-induced damage. In the aggregate, clinical intuitions from studies relating to the device add to the SEM body of knowledge in that:
Healthy tissue is not inflamed;
SEM measures between healthy tissue versus pressure damaged tissue is significantly different;
SEM is recognized as one of the earliest signs of deep and early pressure-induced skin and tissue damage;
Repeated SEM measures at and around bony prominences of specific anatomy provide a measure of tissue health, not just at the bony prominences but also in surrounding tissues within the anatomy;
SEM algorithms significantly exceed diagnostic accuracy of current clinical judgment alone;
The test of SEM, where an SEM delta ≥0.6 indicates anatomically specific skin and tissue damage, aids HCPs in determining healthy versus damaged tissue; even more so, when subjective visual and palpation cues are ambiguous in diagnosing deep tissue injuries and dark skin tone patients.
Modern understanding of PI etiology and pathophysiology has catalyzed a shift in PI prevention pathways. The 2014 and 2019 International Clinical Guidelines describe a subcellular damage threshold beyond which pressure damage manifests [Citation1,Citation34]. Timely, anatomy-specific interventions, applied before this damage threshold is breached, encourage skin and tissue to return to homeostasis [Citation13]. In light of these new insights into PI development where early-stage and deep pressure-related damage can be reversed when detected and intervened upon early enough, consider the current classification system that guides HCPs in their diagnosis of a PI. A Stage I PI is ‘Intact skin with non-blanchable redness of a localized area usually over a bony prominence. Darkly pigmented skin may not have visible blanching; its color may differ from the surrounding area. The area may be painful, firm, soft, warmer, or cooler than adjacent tissue.’ [Citation35]. How could a healthcare practitioner reliably diagnose a condition with inherent contradictory characteristics in its definition, such as redness, but not if dark skin toned, firm or soft, and warmer or cooler?
Current PI prevention pathways rely heavily on whole-body risk assessments, i.e. anatomy-specific assessments are not possible. Furthermore, universal prevention measures are cost and time intensive. Selecting anatomically specific interventions gives HCPs a better chance of preventing skin breakdown due to pressure. In other words, relying on STAs and clinical judgment alone, without an awareness of the condition of the deep tissue, it is not feasible for an HCP to detect developing PIs, nor take appropriate, anatomy-specific interventions in a timely way [Citation22]. Therefore, PI prevention – keeping the skin intact – seems clinically impossible under the current standard of care [Citation13]. In a care pathway where STAs combined with clinical judgment are ineffective in achieving timely detection of sub-epidermal injuries, the clinical challenge has been to know whether, where, and when to intervene when the signs of early-stage pressure damage are sub-clinical. The SEM Scanner solves this clinical challenge by informing HCPs of this subclinical data in identifying SEM changes early before they develop into a PI. Deploying the device into routine PI care pathways allows for microscopic assessments of a localized area exposed to pressure and shear and precise interventions, yielding dramatically better clinical outcomes.
The device is already leading to radical clinical transformations in everyday practice by significantly impacting hospital-acquired PI incidence rates for all patients deemed at risk or higher across multiple care settings. Following the lines of translational science, HCPs informed by SEM Scanner data are able to redesign existing care pathways to prioritize prevention as a quality outcome. With the SEM Scanner providing significant, measurable clinical benefits to a field that urgently needs objective metrics and evidence, it may be possible that the time to modernize PI care pathways has arrived.
Declaration of interest
R Bryant acts as the President for the Association for the Advancement of Wound Care (AAWC)and is a member of the Global Scientific Advisory Board to Bruin Biometrics, LLC, whose device, the SEM Scanner series (Provizio® SEM Scanner and SEM Scanner 200) is profiled in this article.
Z Moore is a trustee of the European Pressure Ulcer Advisory Panel, where she is a member of the Executive Board, Chair of the Scientific Committee, a member of the International Guideline Governance Group and the Advocacy group, and a member of the Global Scientific Advisory Board to Bruin Biometrics, LLC.
V Iyer is a Senior R&D and Medical Affairs Manager at Bruin Biometrics, LLC.
The authors have no other relevant affiliations or financial involvement with any organization or entity with a financial interest in or financial conflict with the subject matter or materials discussed in the manuscript apart from those disclosed.
Reviewer Disclosures
Peer reviewers on this manuscript have no relevant financial or other relationships to disclose.
Additional information
Funding
References
- EPUAP/NPIAP/PPPIA, European pressure ulcer advisory panel, national pressure injury advisory panel and Pan Pacific pressure injury alliance. Prevention and treatment of pressure ulcers/injuries: Clinical Practice Guideline. The International Guideline. EPUAP/NPIAP/PPPIA, 2019.
- Degenholtz HB, Rosen J, Castle N, et al. The association between changes in health status and nursing home resident quality of life. Gerontologist. 2008;48(5):584–592.
- Essex HN, Clark M, Sims J, et al. Health-related quality of life in hospital inpatients with pressure ulceration: assessment using generic health-related quality of life measures. Wound Repair Regen. 2009;17(6):797–805.
- Jackson DE, Durrant LA, Hutchinson M, et al. Living with multiple losses: insights from patients living with pressure injury. Collegian. 2018;25(4):409–414.
- Padula WV, Delarmente BA. The national cost of hospital-acquired pressure injuries in the United States. Int Wound J. 2019;16(3):634–640.
- Guest JF, Vowden K, Vowden P. The health economic burden that acute and chronic wounds impose on an average clinical commissioning group/health board in the UK. J Wound Care. 2017;26(6):292–303.
- Guest JF, Fuller GW, Vowden P. Cohort study evaluating the burden of wounds to the UK’s National Health Service in 2017/2018: update from 2012/2013. BMJ Open. 2020;10(12):e045253.
- Bennett G, Dealey C, Posnett J. The cost of pressure ulcers in the UK. Age Ageing. 2004;33(3):230–235.
- Dealey C, Posnett J, Walker A. The cost of pressure ulcers in the United Kingdom. J Wound Care. 2012;21(6):261–2, 264, 266.
- Gefen A, Ousey K. COVID-19: pressure ulcers, pain and the cytokine storm. J Wound Care. 2020;29(10):540–542.
- Team V, Team L, Jones A, et al. Pressure injury prevention in COVID-19 patients with acute respiratory distress syndrome. Front Med (Lausanne). 2020;7:558696.
- Tang J, Li B, Gong J, et al. Challenges in the management of critical ill COVID-19 patients with pressure ulcer. Int Wound J. 2020;17(5):1523–1524.
- Gefen A, Ousey K. Update to device-related pressure ulcers: SECURE prevention. COVID-19, face masks and skin damage. J Wound Care. 2020;29(5):245–259.20.
- Moore Z, Patton D, Avsar P, et al. Prevention of pressure ulcers among individuals cared for in the prone position: lessons for the COVID-19 emergency. J Wound Care. 2020;29(6):312–320.
- Kim RS, Mullins K. Preventing facial pressure ulcers in acute respiratory distress syndrome (ARDS). J Wound Ostomy Continence Nurs. 2016;43(4):427–429.
- Clay P, Cruz C, Ayotte K, et al. Device related pressure ulcers pre and post identification and intervention. J Pediatr Nurs. 2018;41:77–79.
- Zhang W, Liu X, Wang S, et al. Mechanism and prevention of facial pressure injuries: a novel emergent strategy supported by a multicenter controlled study in frontline healthcare professionals fighting COVID-19. Wound Repair Regen. 2021;29(1):45–52.
- Shelhamer MC, Wesson PD, Solari IL, et al. Prone positioning in moderate to severe acute respiratory distress syndrome due to COVID-19: a cohort study and analysis of physiology. J Intensive Care Med. 2021;36(2):241–252.
- Padula WV, Cuddigan J, Ruotsi L, et al. Best-Practices for preventing skin injury beneath personal protective equipment during the COVID-19 pandemic: a position paper from the National Pressure Injury Advisory Panel (NPIAP). J Clin Nurs. 2021. Advance online publication. DOI:https://doi.org/10.1111/jocn.15682.
- Peko L, Barakat-Johnson M, Gefen A. Protecting prone positioned patients from facial pressure ulcers using prophylactic dressings: a timely biomechanical analysis in the context of the COVID-19 pandemic. Int Wound J. 2020;17(6):1595–1606.
- Nani FS, Stefani KC, Busnardo FF, et al. Ulcer pressure prevention and opportunity for innovation during the COVID-19 crisis. Clinics (Sao Paulo). 2020;75:e2292.
- Gefen A. The sub-epidermal moisture scanner: the principles of pressure injury prevention using novel early detection technology. Wound Int. 2018;9(3):30–35.23.
- Bates-Jensen BM, Mccreath HE, Pongquan V. Subepidermal moisture is associated with early pressure ulcer damage in nursing home residents with dark skin tones: pilot findings. J Wound Ostomy Continence Nurs. 2009;36(3):277–284.
- Bates-Jensen BM, Mccreath HE, Pongquan V, et al. Subepidermal moisture differentiates erythema and stage I pressure ulcers in nursing home residents. Wound Repair Regen. 2008;16(2):189–197.
- Oomens CW, Bader DL, Loerakker S, et al. Pressure induced deep tissue injury explained. Ann Biomed Eng. 2015;43(2):297–305.
- Bouten CV, Oomens CW, Baaijens FP, et al. The etiology of pressure ulcers: skin deep or muscle bound? Arch Phys Med Rehabil. 2003;84(4):616–619.
- Pieper B, Mott M. Nurses’ knowledge of pressure ulcer prevention, staging, and description. Advances in Wound Care. J Prev Heal. 1995;8(3): 34, 38, 40 passim.
- Pancorbo-Hidalgo PL, Garcia-Fernandez FP, Lopez-Medina IM, et al. Risk assessment scales for pressure ulcer prevention: a systematic review. J Adv Nurs. 2006;54(1):94–110.
- Hiser B, Rochette J, Philbin S, et al. Implementing a pressure ulcer prevention program and enhancing the role of the CWOCN: impact on outcomes. Ostomy Wound Manage. 2006;52(2):48–59.
- Moore ZE, Patton D. Risk assessment tools for the prevention of pressure ulcers. Cochrane Database Syst Rev. 2019;1(1):Cd006471.
- Waterlow J. From costly treatment to cost-effective prevention: using Waterlow. Br J Community Nurs. 2005;10(Sup3):S25–S30.
- Bergstrom N, Braden BJ, Laguzza A, et al. The braden scale for predicting pressure sore risk. Nurs Res. 1987;36(4):205–210.
- Norton D. Calculating the risk: reflections on the Norton Scale. Decubitus. 1989;2(3):24–31.
- EPUAP/NPUAP/PPPIA, European pressure ulcer advisory panel, national pressure ulcer advisory panel and Pan Pacific pressure injury alliance. Prevention and treatment of pressure ulcers/injuries: clinical practice guideline. The international guideline. EPUAP/NPUAP/PPPIA, 2014.
- NPUAP, Prevention and treatment of pressure ulcers: Quick Reference Guide. National Pressure Ulcer Advisory Panel, European Pressure Ulcer Advisory Panel and Pan Pacific Pressure Injury Alliance, 2014.
- World Health Organization. International statistical classification of diseases and related health problems (10th ed). 2016. https://icd.who.int/browse10/2016/en (accessed 14 April 2020).
- Scafide KN, Narayan MC, Arundel L. Bedside technologies to enhance the early detection of pressure injuries: a systematic review. J Wound Ostomy Continence Nurs. 2020;47(2):128–136.
- Gefen A, Cohen L, Amrani G, et al. The roles of infrared thermography in pressure ulcer research with focus on skin microclimate induced by medical devices and prophylactic dressings. Wound Int. 2019;10(1):8–15.
- Bader DL, Worsley PR. Technologies to monitor the health of loaded skin tissues. Biomed Eng Online. 2018;17(1):40.
- Ross G, Gefen A. Assessment of sub-epidermal moisture by direct measurement of tissue biocapacitance. Med Eng Phys. 2019;73:92–99.
- FDA. De Novo Classification Decision Summary For SEM Scanner (MODEL 200) 2018. [cited 2021 Apr 08]. Available from: https://www.accessdata.fda.gov/cdrh_docs/reviews/DEN170021.pdf.
- Li L, Li C, Zhang Z, et al. On the dielectric “constant” of proteins: smooth dielectric function for macromolecular modeling and its implementation in DelPhi. J Chem Theory Comput. 2013;9(4):2126–2136.
- Tomaselli VP, Shamos MH. Electrical properties of hydrated collagen. I. Dielectric properties. Biopolymers. 1973;12(2):353–366.
- Okonkwo H, Bryant R, Milne J, et al. A blinded clinical study using a subepidermal moisture biocapacitance measurement device for early detection of pressure injuries. Wound Repair Regen. 2020;28(3):364–374.
- Gershon S, Okonkwo H. Evaluating the sensitivity, specificity and clinical utility of algorithms of spatial variation in sub-epidermal moisture (SEM) for the diagnosis of deep and early-stage pressure-induced tissue damage. J Wound Care. 2021;30(1):41–53.
- Gefen A, Gershon S. An observational, prospective cohort pilot study to compare the use of subepidermal moisture measurements versus ultrasound and visual skin assessments for early detection of pressure injury. Ostomy Wound Manage. 2018;64(9):12–27.
- Gershon S. Using subepidermal moisture level as an indicator of early pressure damage to local skin and tissue. Adv Skin Wound Care. 2020;33(9):469–475.
- Peko L, Gefen A. Sensitivity and laboratory performances of a second-generation sub-epidermal moisture measurement device. Int Wound J. 2020;17(3):864–867.
- Gefen A, Kolsi J, King T, et al. Modelling the cost-benefits arising from technology-aided early detection of pressure ulcers. Wound Int. 2020;11(1):22–29.
- Padula WV, Malaviya S, Hu E, et al. The cost-effectiveness of sub-epidermal moisture scanning to assess pressure injury risk in U.S. health systems. J Patient Saf Risk Manag. 2020;25(4):147–155.
- Clendenin M, Jaradeh K, Shamirian A, et al. Inter-operator and inter-device agreement and reliability of the SEM Scanner. J Tissue Viability. 2015;24(1):17–23.
- Food and Drug Administration. Use of International Standard ISO 10993-1, “Biological evaluation of medical devices - Part 1: evaluation and testing within a risk management process.” Issued by CDRH:2020.
- Park S, Kim CG, Ko JW. The use of sub-epidermal moisture measurement in predicting blanching erythema in jaundice patients. J Wound Care. 2018;27(5):342–349.
- Kim CG, Park S, Ko JW, et al. The relationship of subepidermal moisture and early stage pressure injury by visual skin assessment. J Tissue Viability. 2018;27(3):130–134.
- Bates-Jensen BM, Mccreath HE, Patlan A. Subepidermal moisture detection of pressure induced tissue damage on the trunk: the pressure ulcer detection study outcomes. Wound Repair Regen. 2017;25(3):502–511.
- Swisher SL, Lin MC, Liao A, et al. Impedance sensing device enables early detection of pressure ulcers in vivo. Nat Commun. 2015;6(1):6575.
- Zhang L, Liu P, Dong X, et al. A method for in vivo detection of abnormal subepidermal tissues based on dielectric properties. Biomed Mater Eng. 2014;24(6):3455–3462.
- Bates-Jensen BM, Mccreath HE, Nakagami G, et al. Subepidermal moisture detection of heel pressure injury: the pressure ulcer detection study outcomes. Int Wound J. 2018;15(2):297–309.
- Bates-Jensen BM, Mccreath HE, Kono A, et al. Subepidermal moisture predicts erythema and stage 1 pressure ulcers in nursing home residents: a pilot study. J Am Geriatr Soc. 2007;55(8):1199–1205.
- Harrow JJ, Mayrovitz HN. Subepidermal moisture surrounding pressure ulcers in persons with a spinal cord injury: a pilot study. J Spinal Cord Med. 2014;37(6):719–728.
- Guihan M, Bates-Jenson BM, Chun S, et al. Assessing the feasibility of subepidermal moisture to predict erythema and stage 1 pressure ulcers in persons with spinal cord injury: a pilot study. J Spinal Cord Med. 2012;35(1):46–52.
- Budri AMV, Moore Z, Patton D, et al. Sub-epidermal moisture measurement: an evidence-based approach to the assessment for early evidence of pressure ulcer presence. Int Wound J. 2020;17(6):1615–1623.
- Moore ZE, Cowman S. Risk assessment tools for the prevention of pressure ulcers. Cochrane Database Syst Rev. 2014 Feb 5;(2):CD006471.
- O’Brien G, Moore Z, Patton D, et al. The relationship between nurses assessment of early pressure ulcer damage and sub epidermal moisture measurement: a prospective explorative study. J Tissue Viability. 2018;27(4):232–237.
- Moore Z, Patton D, Rhodes SL, et al. Subepidermal moisture (SEM) and bioimpedance: a literature review of a novel method for early detection of pressure-induced tissue damage (pressure ulcers). Int Wound J. 2017;14(2):331–337.
- Raizman R, Macneil M, Rappl L. Utility of a sensor-based technology to assist in the prevention of pressure ulcers: a clinical comparison. Int Wound J. 2018;15(6):1033–1044.
- Smith G. Improved clinical outcomes in pressure ulcer prevention using the SEM scanner. J Wound Care. 2019;28(5):278–282.
- Ore N, Carver T. Implementing a new approach to pressure ulcer prevention. J Clin Nurs. 2020;34(4):52–57.
- O’Connor R, Moore ZE, O’Connor T. Pressure ulcer detection and risk assessment: the use of sub-epidermal moisture scanning versus existing methodologies, in European Wound Management Association 2016. Bremen, Germany; EWMA. 2016.
- Ana Lucia M. DO, The role of sub-epidermal moisture measurement versus traditional risk assessment and visual skin assessment in the prediction of pressure ulcer risk among adults undergoing surgery. Royal College of Surgeons in Ireland, Thesis, 2018. DOI:https://doi.org/10.25419/rcsi.10763948.v1.
- Musa L, Ore N, Raine G, et al. Clinical impact of a sub-epidermal moisture scanner: what is the real-world use? J Wound Care. 2021;30(3):198–208.
- Padula WV, Mishra MK, Makic MB, et al. Improving the quality of pressure ulcer care with prevention: a cost-effectiveness analysis. Med Care. 2011;49(4):385–392.
- GDPR. REGULATION (EU) 2016/679 OF THE EUROPEAN PARLIAMENT AND OF THE COUNCIL - General data protection regulation. Official Journal of the European Union; 2016.
- FDA. Gov, Use of real-world evidence to support regulatory decision-making for medical devices, C.f.D.a.R.H. U.S. Department of Health and Human Services Food and Drug Administration, Center for Biologics Evaluation and Research, Editor. 2017.
- Burns M, Reducing pressure injury/ulcer (PI/U) ulcer through the introduction of technology, in European Wound Management Association. 2020, EWMA: Virtual.
- Defloor T, Maria FHG. Validation of pressure ulcer risk assessment scales: a critique. J Adv Nurs. 2004;48(6):613–621.
- Padula W, Berke C, Bryant R, NPIAP-WOCN-AAWC Joint Policy Task Force. A collaborative call for changes in reimbursement policies to achieve improvements in hospital safety related to pressure injuries. J Patient Saf. 2021;17(4):e268.