ABSTRACT
Introduction
Transcranial magnetic stimulation (TMS) uses magnetic pulses to induce electrical current in the underlying neuronal tissue. A variety of TMS coils exist on the market, differing primarily in configuration, orientation, and flexibility of the wire windings of the coil. Deep TMSTM utilizes H-Coils, flexible coils with different configurations for stimulating different brain regions implicated in different neuropsychiatric disorders. The H7 Coil, designed to target primarily the medial prefrontal cortex and the anterior cingulate cortex, is FDA-cleared for obsessive-compulsive disorder (OCD). It was chosen as the focus of this review since it recently showed promise in various neuropsychiatric populations in addition to growing understanding of its mechanism of action (MOA).
Areas covered
Here we assembled all peer-reviewed publications on the H7 Coil to showcase its efficacy in: (a) various OCD patient populations (e.g., different degrees of symptom severity, treatment resistance, comorbidities) (b) other neuropsychiatric populations (e.g., addiction, major depressive disorder and autism spectrum disorder).
Expert opinion
While substantial evidence pertaining to the H7 Coil’s efficacy as well as its MOA has accumulated, much work remains. In the final section of this review, we highlight areas of ongoing and future research that will further elucidate the coil’s MOA as well as its full efficacy potential.
1. Introduction
The BrainsWay Deep TMS system is composed of four main components: an electromagnetic H-Coil, TMS stimulator, cooling system, and positioning arm. At present, BrainsWay has 16 patented H-Coils and several stimulators in its portfolio [Citation1] (for properties of the FDA-cleared coils see ). The H1 Coil, which was designed to stimulate the bilateral prefrontal cortex with preference to the left dorsolateral prefrontal cortex (DLPFC), was cleared by the FDA in January 2013 for the treatment of major depressive disorder (MDD). The H7 Coil was designed to primarily stimulate the medial prefrontal cortex (mPFC) and anterior cingulate cortex (ACC, 3 cm3) and was cleared by the FDA in August 2018 for the treatment of obsessive-compulsive disorder (OCD). Additional cortical regions that the H7 Coil stimulates supra-threshold (>100 V/m) include the orbitofrontal cortex (8.4 cm3), DLPFC (10.5 cm3), and pre-supplementary motor area (6.8 cm3) [Citation2]. This coil has two layers of four elliptically shaped windings each one on top of the other, whose major axis ranges from 130 to 70 mm, and minor axis from 105 to 55 mm. Its subdural depth and volume of stimulation are 3 cm and 40.3 cm3, respectively [Citation2]. Like all other H-Coils, they are flexible and conform to the shape of the head for maximum magnetic coupling. Recently, the H4 Coil that was designed to target the bilateral insula and PFC was cleared by the FDA for the treatment of smoking addiction. The experimental system used in the trials that led to the clearances, has two coils in the same helmet, a sham and an active coil; the sham coil does not induce field in the brain but has a similar acoustic artifact and scalp sensations as the active coil, to maintain blinding. The system assigns an active or sham coil based on the patient ID during the high frequency (HF) treatment. Importantly, the H7 Coil has shown safety and efficacy beyond OCD, in various neuropsychiatric indications. This narrative review paper aims to provide an overview of the Deep TMS H7 Coil clinical evidence available to date. Additionally, we offer a glimpse into the foreseeable future and speculate on how the evolution of research on this technology might impact the fields of psychiatry and neurology.
2. Clinical evidence in OCD
A pilot single-center randomized controlled trial (RCT) and a follow-up multicenter RCT led to FDA-clearance of the Deep TMS H7 Coil for the treatment of OCD. The pilot investigated two different stimulation protocols (20 Hz and 1 Hz) against sham on 41 treatment-resistant OCD patients [Citation3]. Clinical response to treatment was measured using the Yale-Brown Obsessive Compulsive Scale (Y-BOCS). All treatments were administered following personalized symptom provocation, and electrophysiological (EEG) recordings were obtained during a Stroop behavioral task to gain insight into the mechanism of action of Deep TMS in OCD (will be elaborated on toward the end of this section). Since the interim analysis revealed that only the HF significantly reduced clinical symptoms compared to sham, the LF arm was discontinued. At the end of the study, the HF group showed significantly greater clinical improvement (reduction in Y-BOCS) compared to sham (p < 0.01; ). No significant adverse events were reported.
Figure 2. Clinical outcomes following Deep TMS H7 Coil treatment in the OCD pilot and multicenter trials (adapted from [Citation3] and [Citation4]). (a) outcomes in the pilot study: on the left panel mean % change from baseline in Y-BOCS scores throughout the trial in black circles for the active treatment group and in white circles for the sham group. On the right panel the response rates (defined as ≥30% reduction from baseline Y-BOCS) are presented for the active treatment group on the right bar and for sham on the left bar. (b) outcomes in the multicenter study: on the left panel mean change from baseline in Y-BOCS scores throughout the trial in green circles for the active treatment group and in Orange circles for the sham group. Numbers above line graphs represent number of subjects with available scores for that timepoint. On the right panel the response rates (full and partial response defined as ≥30% and ≥20% reduction from baseline Y-BOCS, respectively) are presented for the active treatment group in green bars and for sham in Orange bars. Asterisks denote statistical significance *-p < 0.05, **-p < 0.01.
![Figure 2. Clinical outcomes following Deep TMS H7 Coil treatment in the OCD pilot and multicenter trials (adapted from [Citation3] and [Citation4]). (a) outcomes in the pilot study: on the left panel mean % change from baseline in Y-BOCS scores throughout the trial in black circles for the active treatment group and in white circles for the sham group. On the right panel the response rates (defined as ≥30% reduction from baseline Y-BOCS) are presented for the active treatment group on the right bar and for sham on the left bar. (b) outcomes in the multicenter study: on the left panel mean change from baseline in Y-BOCS scores throughout the trial in green circles for the active treatment group and in Orange circles for the sham group. Numbers above line graphs represent number of subjects with available scores for that timepoint. On the right panel the response rates (full and partial response defined as ≥30% and ≥20% reduction from baseline Y-BOCS, respectively) are presented for the active treatment group in green bars and for sham in Orange bars. Asterisks denote statistical significance *-p < 0.05, **-p < 0.01.](/cms/asset/e14a1a13-6f4c-4b61-92eb-222b5ef695ac/ierd_a_2013803_f0002_oc.jpg)
Following these positive results, a multicenter RCT was conducted to confirm the safety and efficacy of HF stimulation with the Deep TMS H7 Coil on 100 OCD patients from 11 sites [Citation4]. All patients had moderate-severe OCD and were required to be stable on their OCD medications for ≥10 weeks at the beginning of the study. The study design consisted of a 2–3 weeks screening phase, a 6-week treatment period of 5 sessions per week, and a follow-up 4 weeks after treatment ended. The change in Y-BOCS score from baseline to the end of the treatment phase in the Deep TMS group compared to sham was defined as the primary endpoint. The secondary endpoints of the study included change from baseline compared to 6 weeks and 10 weeks of the Y-BOCS score, Clinical Global Impression scale, Severity (CGI-S) and Improvement (CGI-I) subscales, response rate, partial response rate, and remission rate. At the end of treatment (6-week visit), there was a significantly larger reduction in Y-BOCS scores in the Deep TMS group compared with the sham group (−6.7 vs. −3.6, p = 0.01) for an effect size (Cohen’s d) of 0.69. During the 10-week visit, the adjusted Y-BOCS score decreased by 6.5 points (95% CI: [4.3;8.7]) in the Deep TMS group versus 4.1 points (95% CI: [1.9;6.2]) in the sham group; these decreases were both statistically significant. The difference between the treatment groups showed an effect size (Cohen’s d) of 0.62 and it was statistically significant (p = 0.038) and clinically meaningful. The demonstrated effect sizes in the multicenter study both at 6 weeks and at 10 weeks are considered to be greater-than-medium to larger magnitude. Thus, the treatment effect is maintained for at least 4 weeks after completion of all treatment sessions (i.e., 10 weeks from baseline; ).
At the 6-week visit, the response rate (defined as a reduction from a baseline of at least 30% in Y-BOCS) in the Deep TMS group was 38.1% versus 11.1% in the sham group. The response rate was significantly higher in the Deep TMS group compared to the sham group (p = 0.0033; ). The partial response rate (defined as a reduction from a baseline of at least 20% in Y-BOCS score) at the 6-week visit in the Deep TMS group was 54.8% versus 26.7% in the sham group. This difference is also statistically significant (p = 0.0076; ).
The responder results are not only statistically significant but also clinically meaningful, as demonstrated by the effect size expressed in terms of Number Needed to Treat (NNT). Based on the response rates, the effect size as obtained by the NNT = (1/difference in response rates) is 3.7, which means that for every 4 patients treated with the Deep TMS System, 1 subject will have a response due to the device.
During the 10-week visit, the response rate was 45.2% in the Deep TMS group compared to 17.8% in the sham group, with the difference between the Deep TMS group and the sham group remaining statistically significant (p = 0.0057). There was also a further increase in the response rate at the 10-week visit (45%) in the Deep TMS group compared to the 6-week visit (38%), demonstrating a further positive treatment effect maintained over time. The effect size, as obtained by the NNT, was 3.64, which still means that for every 4 patients treated with the Deep TMS System, 1 subject will have a response due to the device.
In the categorical analysis, 70% of the Deep TMS subjects were evaluated by clinicians as having some global improvement (ranging from minimal to very much improved) and 49% reported an ‘Improved’ (moderate to very much improved) clinical state at 6 weeks because of the Deep TMS treatment. This is compared to only 58% of the subjects in the sham group who reported some improvement, with only 21% experiencing a moderate to very much ‘Improved’ clinical state. There is a statistically significant difference (p = 0.0112) between the percentage of Deep TMS subjects experiencing an ‘Improved’ clinical state compared to the sham group at 6 weeks. CGI-Improvement results are maintained 4 weeks after treatment at the 10-week visit. At week 6, more subjects had an ‘Improved’ CGI Severity (CGI-S) score in the Deep TMS group (61%) than in the sham group (32.6%), and this difference was reported to be statistically significant (p = 0.0221). The CGI-I and CGI-S results support and strengthen the significant clinical effect of Deep TMS treatment.
A post-hoc analysis of the multicenter trial data found that a faster rate of symptom improvement was significantly predicted by the following factors at baseline: older age, lower severity, and lower functional disability. This was regardless of the treatment condition. Greater treatment benefit was found to be moderated by baseline OCD severity, such that for more severe patients (Y-BOCS>28) Deep TMS showed stronger efficacy relative to sham at post-treatment and follow-up () [Citation5].
Figure 3. Characterization of the response to Deep TMS H7 Coil among different OCD patient populations (adapted from [Citation5,Citation6], and [][Citation7]). (a) mean change from baseline in Y-BOCS scores throughout the multicenter trial among 2 subsets of patients: lower baseline severity (Y-BOCS<27, upper panel) and higher baseline severity (Y-BOCS≥28, bottom panel) presented in red line graphs for active treatment group and in blue line graph for sham. (b) schematic timeline of the study (top panel), samples sizes and response rates (middle panel), and the percent of change from baseline in Y-BOCS scores for each individual from the different cohorts at the end of the 6-weeks treatment and at the 10-weeks Follow-up (bottom panel). (c) improvement in clinical symptoms of comorbid OCD-MDD patients from the real-world post-marketing data. Mean ± SEM % change in Y-BOCS and MDD scores from baseline as a function of number of Deep TMS sessions. Scatter plot demonstrating the correlation of improvement in both scores is shown in the top right insert. Asterisks denote a significant response rate difference between the indicated groups, where *p < 0.05, **p < 0.01 (Fisher’s exact test).
![Figure 3. Characterization of the response to Deep TMS H7 Coil among different OCD patient populations (adapted from [Citation5,Citation6], and [][Citation7]). (a) mean change from baseline in Y-BOCS scores throughout the multicenter trial among 2 subsets of patients: lower baseline severity (Y-BOCS<27, upper panel) and higher baseline severity (Y-BOCS≥28, bottom panel) presented in red line graphs for active treatment group and in blue line graph for sham. (b) schematic timeline of the study (top panel), samples sizes and response rates (middle panel), and the percent of change from baseline in Y-BOCS scores for each individual from the different cohorts at the end of the 6-weeks treatment and at the 10-weeks Follow-up (bottom panel). (c) improvement in clinical symptoms of comorbid OCD-MDD patients from the real-world post-marketing data. Mean ± SEM % change in Y-BOCS and MDD scores from baseline as a function of number of Deep TMS sessions. Scatter plot demonstrating the correlation of improvement in both scores is shown in the top right insert. Asterisks denote a significant response rate difference between the indicated groups, where *p < 0.05, **p < 0.01 (Fisher’s exact test).](/cms/asset/69839eb0-1235-4f5f-b0fe-86600ba35bb3/ierd_a_2013803_f0003_oc.jpg)
Another post-hoc analysis of the multicenter trial was performed to examine the effect of treatment resistance, as expressed by a number of failed medication trials and prior CBT, on the efficacy of Deep TMS in OCD [Citation6].
Patients were grouped based on their prior treatment resistance into four cohorts: patients who had insufficient response to 1) 1–2 meds; 2) 3+ meds; 3) prior CBT (>2 months with a therapist); and patients who had 4) no prior CBT. The largest cohorts were the prior CBT (68%, 57/84 completers) and the 3+ meds (63%, 53/84 completers). A significantly higher response rate at post-treatment with Deep TMS compared with sham was found for the larger cohorts of 3+ meds (41.4% vs. 8.3%, p = 0.0109) and prior CBT (33.3% vs. 3.3%, p = 0.0041). Thus, Deep TMS is an effective treatment option, regardless of prior non-response to pharmacotherapy (SRIs, antipsychotics) or psychotherapy (CBT) (). In other words, the MOA of Deep TMS for OCD appears to be different from that of pharmacotherapy/CBT. It is hypothesized that the underlying mechanism of Deep TMS in OCD is a direct modulation of the cortico-striatal-thalamo-cortical (CSTC) circuitry.
Converging evidence from neuroimaging and cognitive-behavioral study points to alterations in several regions of the CSTC circuits as underlying the deficits typical for OCD [Citation8]. The ACC and mPFC are the components within CSTC most commonly implicated in OCD pathology [Citation9].
Feelings of doubt, worry, and repetitive behavior, key symptoms of OCD, have been linked to hyperactive error signals in the brain. The error-related negativity (ERN) [Citation10] is an event-related potential in the theta frequency band (4–8 Hz) that peaks 50–150 ms following errors on many speeded reaction-time (RT) tasks (e.g. the Stroop and flanker). The ERN is recorded with electroencephalography (EEG) over mPFC and consistently attributed to the ACC [Citation11,Citation12]. The ERN is thought to reflect monitoring processes of ongoing task performance necessary for mistake prevention, emotional response to error, and cognitive conflict [Citation10,Citation13–15]. An overactivation of a monitoring system in the ACC in response to self-induced mistakes during tasks like the Stroop, Flanker, or Stop-Signal has been repeatedly demonstrated for OCD patients [Citation13,Citation15–24]. Enhanced ERN amplitudes and post-error slowing (PES) have also been reported for both OCD patients and their unaffected first-degree relatives [Citation10,Citation20,Citation25–34]. Similarly, perceived error-related theta activity (PERTA), that is also recorded over mPFC and attributed to ACC, was found to be enhanced in OCD patients and their unaffected siblings [Citation34–37]. This suggests that the constantly over-activated detection system in OCD evolves in vulnerable individuals with neuronal predisposition.
The most beneficial interventions in OCD have therefore been those that were able to target the mPFC and ACC. For example, increased resting state and task-related activity in the ACC were found in participants that improved after CBT treatment. Saxena and colleagues [Citation38] suggested that enhancement of ACC activity may be a primary mechanism of action (MOA) of CBT for OCD. Deep rTMS 1 Hz treatment over the mPFC with a double-cone coil was shown to improve both OCD symptoms and response time, again suggesting a correlation between error monitoring impairment and OCD pathophysiology. A recent 1 H-MRS study [Citation39] found significant increases in levels of NAA, Choline, and Creatine in the ACC following treatment with Deep TMS H7 Coil in OCD patients, indicating direct neural stimulation of this region. A recent modeling study [Citation41] found the H7 induces significant electric field in various deep structures including the ACC.
Further elucidation of the MOA was provided by analyses of a behavioral task administered in the pilot study and its recorded electrophysiological correlate. Both the Stroop task and the ERN were analyzed as objective measures of treatment-induced modifications. Significant enhancement in the ERN was found following active treatment but not sham (p < 0.01; ). Across patients, this enhancement was correlated with their symptom improvement (p < 0.01; ) [Citation3]. The analysis of the Stroop data demonstrated a significant reduction in post-error slowing (PES) following Deep TMS but not sham (F1,19 = 5.39 p < 0.05 vs F1,19 = 1.06 p < 0.32; ). Comparable analysis of post-error accuracy (PEA) revealed robust reduction (F1, 19 = 16.4, p < 0.001) but without an interaction effect with treatment or time (F1, 19 = 0.04, p = 0.83), indicating that PEA was not influenced by treatment and that accuracy levels were probably reduced following errors due to the contradiction between PES and task requirements (i.e. time limits). Moreover, the treatment-induced changes in PES and PEA were significantly correlated with each other in the active but not in the sham group (r = 0.63 p < 0.05 vs r = 0.03 p = 0.93; ). That is, shorter PES following active treatment was associated with reduced PEA, as seen in healthy population, suggesting treatment-induced normalization of the speed-accuracy trade-off [Citation42].
Figure 4. Effect of treatment with Deep TMS H7 Coil on theta power and task performance (adapted from [Citation3,Citation42]). (a) average theta power in the active treatment group in black bars and sham treatment group in white bars following correct and mistake responses, pre-treatment (left) and post-treatment (right). (b) correlation between changes in Y-BOCS scores and ERN amplitudes (Pre-minus post-treatment) for the active treatment group (left; r = 0.63, p < 0.01) and sham treatment group (right; r = −0.42, p < 0.26). (c) average RTs (left) following correct and erroneous responses, and accuracy (right) following correct and erroneous responses. (d) correlation between changes in PEA and PES following active treatment (left) and following sham treatment (right). Data are presented as Mean ± SEM. *p < 0.05, **p < 0.01, ***p < 0.001.
![Figure 4. Effect of treatment with Deep TMS H7 Coil on theta power and task performance (adapted from [Citation3,Citation42]). (a) average theta power in the active treatment group in black bars and sham treatment group in white bars following correct and mistake responses, pre-treatment (left) and post-treatment (right). (b) correlation between changes in Y-BOCS scores and ERN amplitudes (Pre-minus post-treatment) for the active treatment group (left; r = 0.63, p < 0.01) and sham treatment group (right; r = −0.42, p < 0.26). (c) average RTs (left) following correct and erroneous responses, and accuracy (right) following correct and erroneous responses. (d) correlation between changes in PEA and PES following active treatment (left) and following sham treatment (right). Data are presented as Mean ± SEM. *p < 0.05, **p < 0.01, ***p < 0.001.](/cms/asset/c71e79a6-f2a1-4af8-8753-2b2a1c70e3ec/ierd_a_2013803_f0004_b.gif)
Real-world data on treatment details and outcome measures were obtained from 22 clinical sites with the H7 Coil. The primary outcome measure was response (30% reduction in Y-BOCS score from baseline to endpoint), and the secondary outcome measures were first response (the first time the Y-BOCS score has met response criteria) and sustained response (when two consecutive Y-BOCS scores met response criteria). Response rate after 29 Deep TMS treatments was 58% – higher than the 38.1% reported in the multicenter trial. Overall, the first and sustained response rates were 72.6% and 52.4%, respectively. The average number of sessions required to reach the first response was 18(±9.4) or 28(±22.4) days. The average number of sessions required to reach a sustained response was 19(±9.6) or 30(±24.5) days. The onset of the effect is quick compared to psychotherapy and pharmacotherapy where it is usually between 10 and 13 weeks [Citation43]. Additionally, a continuous gradual reduction in YBOCS was evident up to 40 Deep TMS sessions () [Citation43].
Figure 5. Change in clinical response as a function of number of treatment sessions with Deep TMS H7 Coil (modified from Citation43,Citation43). Total Y-BOCS score (green line) and % change from baseline in Y-BOCS score (blue line). Shown as means ± SEMs. Numbers above the x axis indicate numbers of subjects who had Y-BOCS scores at the respective number of sessions.
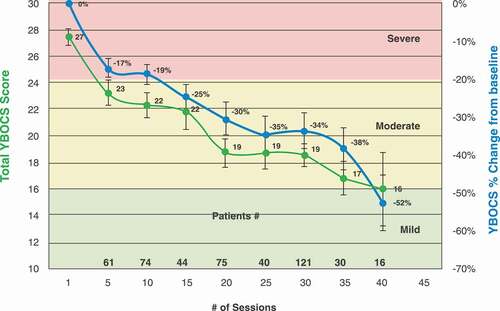
To answer the question of durability of the effect for Deep TMS H7 Coil in OCD, data on responders from a single site (multicenter and open label) was analyzed. Durability was defined as days of loss of efficacy by patient report and clinician survey. Inclusion criteria, other than response, included ≥3 months follow-up (unless durability was lost earlier). This resulted in 38 responders with available durability data. Using a survival analysis, it was found that the Deep TMS effect was durable in 72% (26/36) patients after 3 months, and in 50% (18/36) patients after 6 months. A significant negative correlation was found between durability and the number of failed medications (p = 0.04), such that patients with only one prior medication had significant longer durability (p = 0.007; 711 vs. 264 days). A significant negative correlation with age was found in patients with durability longer than 3 months (p = 0.012). Baseline Y-BOCS score, prior CBT failure, and gender did not correlate with durability. Overall, Deep TMS for OCD demonstrated an average durability of 426 days and median durability of 201 days [Citation40].
3. Comorbid OCD-MDD
OCD is often presented with psychiatric comorbidities, the most common (>50%) being depression that can complicate treatment and increase the risk of suicide. Although the conventional FDA-cleared Deep TMS protocol for MDD stimulates the DLPFC (with H1 Coil), there is been accumulating evidence for the efficacy of mPFC stimulation in MDD. Therefore, a post-hoc analysis of the OCD multicenter RCT set out to investigate whether treatment with the H7 Coil alone was sufficient to also improve comorbid MDD symptoms [Citation44]. The analysis included 19 patients with comorbid OCD-MDD (HDRS-21 ≥ 16) and found a significant decrease in MDD symptoms (HDRS score) from baseline starting at week 2 of treatment (p < 0.05) and up to follow-up (1-month post-treatment, p < 0.005)) in the Deep TMS group but not in the sham group.
A second analysis further addressed this question in a larger sample of comorbid OCD-MDD patients from the OCD post-marketing dataset that were treated with the H7 Coil only [Citation7]. Fifty-nine patients with baseline OCD (Y-BOCS≥20) and at least moderate MDD were included in the analysis that found a significant decrease in both Y-BOCS and MDD scores following 5 Deep TMS sessions and at any point beyond that. Most patients (71.4%) demonstrated benefit from treatment in both disorders after 30 sessions, with an average decrease of 30% in Y-BOCS score and an average 38% decrease in MDD scores (). This improvement continues with further (up to 40) Deep TMS sessions. The treatment-induced changes in Y-BOCS and MDD scores are significantly correlated with one another (384 pairs, Spearman r = 0.3923, p < 0.0001; insert).
4. BDD
Body dysmorphic disorder (BDD) is an OCD-related disorder in which the patient’s obsessions and/or compulsions focus on physical appearance. Since the safety and efficacy of the Deep TMS H7 Coil have been demonstrated for OCD, it might also be beneficial to patients with BDD.
A recent case report [Citation45] presented a 25-year-old-woman with history of performance anxiety and attention deficit disorder who developed significant anxiety after her first job offer. Antidepressants and antipsychotics demonstrated no benefit, and she was hospitalized due to her BDD and suicidal depression. Treatment with Deep TMS H1 Coil over the left PFC failed to reduce her obsessions or depression, but she was no longer actively suicidal. Then, in addition to the H1 Coil, the H7 Coil over the mPFC-ACC was added with personalized provocation immediately prior to each session. Outcome measures included CGI-S, Beck Depression Inventory (BDI) and Y-BOCS. The patient remitted (CGI-S 7/1, BDI 39/11, Y-BOCS 17/9) after 32 daily H7-coil session. Treatments continued twice per week for 12 weeks, and she remained gainfully employed in remission for close to 2 years without further Deep TMS. This initial evidence points to the potential benefit of Deep TMS over the mPFC-ACC for BDD patients who fail to respond to pharmacotherapy.
5. Autism
Autism spectrum disorder (ASD) is a complex developmental condition that involves persistent challenges in social interaction, speech, and nonverbal communication, and restricted/repetitive behaviors. The mPFC is an important node within functional networks involved in mentalizing abilities crucial to social interactions that were found to be impaired in adults with ASD. Two recent studies of Deep TMS in ASD investigated its effect on different common ASD deficits. The first study examined the changes in emotional and cognitive processing following daily HF (5 Hz) Deep TMS H7 Coil sessions over 5 weeks in two high-functioning ASD patients [Citation46]. Assessments included a computerized cognitive battery, tasks for testing emotional recognition, and clinical questionnaires. Both patients improved in a variety of cognitive functions, with a global improvement of 20% (for P1) and 30% (for P2) in the neuropsychological battery. Emotional recognition tasks also revealed that recognizing emotions in others became easier. Patients reported being more aware of emotional and social cues already during the first 2 weeks of treatment, as well as experiencing increased attention and decisiveness throughout the course of treatment. The self-reported questionnaires showed slight improvement in autistic symptoms (AQ) and empathy (IRI). The most noticeable effect, however, was the decrease in OCD-like symptoms at the end of the treatment as measured by the Y-BOCS scale. This decrease was already reported by the patients during the first 2 weeks of treatment and follow-up assessment indicated that it is still significant even 2 months after the end of treatment.
The second study was a prospective RCT that investigated social relating [Citation1,Citation47]. Twenty-eight adults with high-functioning ASD or Asperger’s disorder completed 2 weeks of weekday treatment sessions (5 Hz, 10 s train, 20 s inter-train interval, 1500 pulses/session). Assessments were conducted at baseline, post-treatment, and at one-month follow-up. Participants in the Deep TMS group, compared with the sham group, demonstrated a significant reduction in social related symptoms at post-treatment and follow-up. Additionally, a reduction in self-oriented (socially related) anxiety during difficult and emotional social situations was found only for the Deep TMS group from baseline to follow-up. The results of these studies suggest that Deep TMS over mPFC might be beneficial in high-functioning ASD patients with OCD/anxiety symptoms.
6. Addiction
The ACC, mPFC, and their interactions with cortical and subcortical areas subserve cravings, reward-related decision-making, and top-down control of drug seeking [Citation48–53].
6.1. CUD
A recent pilot study investigated the effect of Deep TMS H7 Coil on cocaine self-administration [Citation54]. The study admitted 18 cocaine use disorder (CUD) volunteers to an inpatient unit for the duration of the study and assigned each to one of the 3 study arms: HF (10 Hz), LF (1 Hz), and sham. Deep TMS was delivered on weekdays across 3 weeks. In order to directly measure cocaine-seeking behavior, CUD participants chose between cocaine and an alternative reinforcer (money) during three self-administration sessions at baseline (session 1), after 4 days of Deep TMS (session 2), and after 13 days of Deep TMS (sessions 3). The number of choices for cocaine was the outcome measure, and it was found that there was a significant decrease in the number of choices for cocaine between sessions 2 and 3 only in the HF group (p = 0.02). These results are in line with existing evidence of the efficacy of HF rTMS over PFC in treating CUD.
6.2. AUD
Fifty-one recent abstinent, treatment-seeking patients with moderate-severe alcohol use disorder (AUD) were randomized to sham or active Deep TMS H7 Coil [Citation55,Citation56]. Treatment included 15 sessions over 3 weeks, followed by 5 sessions over 3 months of follow-up. Each session delivered 100 trains of 30 pulses at 10 Hz. The primary predefined outcome was a reduction in the percentage of heavy drinking days (pHDD), obtained using timeline follow-back (TLFB) interviews. Secondary analyses included self-reports of craving, ethylglucuronide in urine, and brain imaging measures. Results showed that both craving following treatment and pHDD during follow-up were significantly lower in the active vs. sham group (pHDD = 2.9 ± 0.8% vs 10.6 ± 1.9%, p = 0.037). Active Deep TMS was associated with decreased resting state functional connectivity of the dorsal ACC with the caudate nucleus, decreased connectivity of the mPFC to subgenual ACC (sgACC) and lower ACC activation during risky decision-making. This study provides an initial proof-of-concept for Deep TMS targeting midline frontal cortical structures as a treatment for alcohol addiction. Therapeutic benefits of Deep TMS appear to be associated with persistent changes in brain network activity.
7. MDD case series in people who failed H1-coil Deep TMS
Current FDA-cleared TMS protocols for TRD target primarily the left DLPFC. These protocols fail to benefit a large population of TRD patients, probably due to the heterogeneity of the disorder that affects multiple cortical and subcortical networks. As such, novel approaches and targets are required for the treatment of MDD in general and TRD in particular. One such approach is to stimulate the mPFC, including the ACC, using Deep TMS H7 Coil. A recent case series examined the efficacy of Deep TMS over the mPFC-ACC in patients who failed Deep TMS over the left DLPFC [Citation57]. Nine severely depressed patients without current OCD or PTSD, who failed to respond to a course of Deep TMS H1 Coil in the current episode were treated with the H7 Coil over the mPFC-ACC for 5 days a week (20 Hz, 50 trains, 20 sec ITI, 2000 total pulses). Once patients responded, treatments were decreased to twice/week. Evaluations administered to patients weekly included Beck depression and anxiety inventories (BDI, BAI), patient health questionnaire (PHQ-9), and CGI. Remission was defined as a CGI-S score ≤2 or BDI score <12; response to treatment was defined as a 2-point drop in CGI or 50% drop in BDI score. Five out of 9 (56%) severely treatment-resistant patients are remitted after receiving the H7 Coil treatment. One patient responded with CGI criteria but not BDI criteria. Three patients did not benefit from the H7 Coil. The only adverse events were local scalp discomfort and temporary headaches. This is the first report of Deep TMS H7 Coil over the mPFC-ACC for MDD. The demonstration that this coil led to remission in patients that failed to benefit from lateral PFC stimulation supports basic evidence that the mPFC-ACC regions are implicated in the pathophysiology of MDD, and for a portion of individuals such treatment may be a first-line intervention.
8. PTSD
Post-traumatic stress disorder (PTSD) is a debilitating anxiety disorder caused by traumatic experiences. Currently, the most efficacious treatment for PTSD is exposure therapy. However, it is still limited. Since Deep TMS of the mPFC has shown preliminary efficacy in PTSD [Citation58] and mPFC is known to be involved in extinction learning, it stands to reason that combining exposure therapy with mPFC stimulation might enhance its effectiveness. One hundred and twenty-five patients participated in an RCT investigating the safety and efficacy of Deep TMS H7 Coil following a brief exposure procedure in PTSD [Citation59]. Deep TMS treatment included 12 sessions across 4 weeks with each session immediately following exposure to the patient’s trauma narrative using script-driven imagery (SDI). The primary endpoint was a change in the Clinician Administered PTSD Scale (CAPS-5) score from baseline to week 5. While both groups demonstrated a significant improvement in CAPS-5 score at week 5, the improvement in the Deep TMS group was smaller than in the sham group (16.32 vs. 20.52, p = 0.027). This improvement was enhanced at follow-up (week 9) and remained smaller in the Deep TMS group compared to sham (19 vs. 24.4, p = 0.024). While it is likely that the significant improvement in PTSD symptoms in both groups was due to the SDI exposure, it is surprising that the Deep TMS stimulation had a detrimental effect relative to sham rather than a beneficial one. It could be that repeated HF stimulation of the mPFC with the Deep TMS H7 Coil interferes with the trauma-mediated extinction. Future studies should explore whether memory reactivation with inhibitory rather than excitatory stimulation of mPFC is advantageous when coupled with SDI. Indeed, a recent study [Citation60] found that low-frequency Deep TMS H7 Coil led to decreased connectivity of the mesial PFC with the cingulate cortex and with the ventral striatum, and to increased connectivity between the cingulate cortex and the ventral striatum. These findings may hint that low-frequency Deep TMS over medial PFC may increase response inhibition following exposure to trauma. Future studies should investigate these possibilities.
9. Conclusions
The reviewed studies demonstrate the efficacy of the Deep TMS H7 Coil in several neuropsychiatric disorders. Specifically, the evidence in OCD, points to the effectiveness of this treatment in treatment-resistant patients who have failed to achieve sufficient benefit from pharmacotherapy and CBT, irrespective of the degree of resistance (i.e. number of failed past treatments). Interestingly, signs of clinical improvement (response) are achieved with Deep TMS in a shorter timeframe (6 weeks) than typical for pharmacotherapy (10–13 weeks [Citation61]). The above suggests a MOA for Deep TMS in OCD that is distinct from that of pharmacotherapy and CBT. Notably, more severe patients (higher baseline Y-BOCS), despite showing a slower timeline of improvement, demonstrate greater benefit from Deep TMS treatment and a longer treatment course results in higher response rates, including remission. Additionally, OCD patients with MDD comorbidity not only achieve improvement in their OCD symptoms with the H7 Coil but experience relief in their MDD symptoms as well. This observation, in conjunction with evidence of symptom improvement in MDD patients who have failed to achieve response with the H1 Coil, provides a compelling argument for the involvement of mPFC and ACC in the pathophysiology of both OCD and MDD. While the underlying neural MOA of the H7 Coil in OCD warrants further investigation using imaging techniques, the neuromodulatory effect of the H7 Coil in AUD has been delineated. Thus, if HF stimulation with the H7 Coil following symptom provocation in OCD reduced functional connectivity (FC) between the mPFC and ACC in the same manner it reduced FC between mPFC and sgACC following provocation with alcohol-related cues in AUD, it would explain the clinical improvement through a mechanism of reduction in connectivity. This would be in line with the well-known increases in activity and connectivity of CSTC in OCD that have been found to be correlated with symptom severity and reduced following successful treatment.
One might speculate that this mechanism could explain the interference of the H7 Coil HF stimulation with the SDI in the PTSD study (i.e. reduction in FCin this case, between the mPFC and amygdala). In PTSD, where the deficit appears to be reduced by inhibitory control of the mPFC over amygdala-mediated fear response, an inhibitory Deep TMS protocol (i.e., LF or continuous theta burst stimulation (cTBS)) might be more appropriate, strengthening the mPFC-amygdala connectivity, and reinforcing the SDI effect.
Further evidence from smaller studies has also been provided to showcase the potential of the Deep TMS H7 Coil in a wider array of neuropsychiatric disorders from addiction (both CUD and AUD) to BDD to Autism.
10. Expert opinion
While much work has been done with the Deep TMS H7 Coil in the neuropsychiatric space, many important questions remain unanswered and require further investigation. Below we discuss our view of the coming years and the types of work that might provide information pertaining to these open issues.
10.1. Mechanism
Initial imaging evidence hinting to a potential MOA of the Deep TMS H7 Coil has been provided in alcohol dependence. Behavioral and electrophysiological mechanistic evidence have been provided for OCD. Several clinical trials that are underway or planned for the immediate future will further address the critical topic that is the MOA of Deep TMS. Among those is an H7 Coil vs. H1 Coil non-inferiority in the MDD trial that has just finished recruiting. The results will reveal whether medial prefrontal stimulation is as effective in alleviating MDD symptoms as the lateral prefrontal stimulation, which is the current standard (FDA-approved) protocol. Importantly, this trial also aims to contribute to treatment personalization efforts by identifying symptom clusters and electrophysiological biomarkers that will define patients that will benefit from medial prefrontal stimulation and those that will benefit from lateral prefrontal stimulation.
Another important area of investigation is a more extended OCD treatment protocol with a long-term follow-up, as well as a structural and functional imaging component. This would confirm the efficacy of Deep TMS for OCD, further elucidate its MOA, and answer the following questions: Will severe patients regain the ability to function? Will a patient with severe OCD reach mild severity or even remission with continued Deep TMS? How durable is the effect of Deep TMS for OCD? Will OCD patients need maintenance or retreatment?
10.2. Combining treatments
Due to the burden of frequent treatments, TMS is generally reserved for patients who have failed prior treatment with pharmacotherapy and/or CBT. We suspect that the MOA for TMS in OCD is different from medication and CBT, as prior failure has no impact on TMS outcome. There is evidence of an increased period of plasticity several minutes after TMS. Can this enhanced period of plasticity be utilized to administer brief computerized cognitive therapy?
10.3. Role of provocation
Deep TMS can be delivered concurrently with provocations in order to enhance the effect of stimulation [Citation62]. Proof of concept has been provided by studies in PTSD, smoking cessation, and depression [Citation58,Citation63,Citation64], all of which compared stimulation immediately following provocation to stimulation without provocation and found better response to stimulation when provocation preceded it. In OCD, provocation continues to be used clinically as part of the Deep TMS treatment protocol, although its effects have not been isolated. The theoretical explanation for the synergistic effect of Deep TMS paired with provocation is that the provocation engages the target circuitry for stimulation by retrieving items stored in long-term memory, thereby making them labile to modulation by stimulation [Citation3,Citation65,Citation66]. This process of reconsolidation of fear/distress-inducing memories as a result of activating obsessive-compulsive triggers might allow an opportunity to facilitate fear extinction with stimulation of the relevant circuitry during the 6 h window of memory reactivation (when the memory trace is labile [Citation67,Citation68]). Converging evidence from pharmacological and neuroimaging studies provides support for the update of fear memories during the reconsolidation window [Citation68,Citation69]. Thus, it appears that the role of symptom provocation in OCD is to activate the specific dysfunctional circuitry, consequently allowing the H7 Coil that stimulates deep and broadly to modulate the circuit more selectively. In other words, Hebbian plasticity is induced by Deep TMS [Citation70] with the specificity of the circuit being induced by the provocation [Citation71,Citation72].
10.4. Potential improvements – Rotational field coil
The goal of rotational field TMS (rfTMS), a newly developed method, is to reduce TMS’s sensitivity to coil orientation with respect to neurons. The principle of the method is to superimpose the field of two orthogonally positioned TMS coils and stagger the two pulses with a 90° (one-fourth cycle) phase delay, thereby producing a circular polarization (i.e. rotating electric field vector). This potentially results in stimulation of a larger portion of the neuronal population in a specific brain region. A recent study compared the effects of rfTMS and unidirectional (conventional) TMS (udTMS) in the hand and foot motor cortices and found that, for comparable induced electric field amplitude, rfTMS induced significantly lower resting motor threshold and higher motor evoked potentials than those induced by udTMS [Citation73]. In other words, rfTMS induces stronger physiological effects in targeted brain regions at significantly lower intensities. With repeated applications, this has the potential for more effective changes in neuronal excitability and synaptic plasticity, and hence enhanced clinical impact in various brain disorders.
Expanding the concept of rfTMS for three or more coils with synchronized operation of one-fourth cycle intervals might induce field rotation in three dimensions in brain regions exposed to the field of the three coils, recruiting neuronal structures with different orientations. Another advantage might hypothetically be increased focality at depth. This can be achieved by integrating the rfTMS with the previously demonstrated multi-channel method of combined operation of coils with various depth profiles [Citation74]. For instance, a coil array with the H7-coil to apply rfTMS and coils with faster attenuating depth profiles (e.g., Figure-8)might decrease the effects in superficial brain regions and increase stimulation selectivity at depth. Since one of the main limitations of current TMS technology is the ‘depth-focality tradeoff,’ this potential improvement might open novel research and clinical opportunities. The rfTMS is currently being investigated in trials for stroke rehabilitation, depression, and multiple sclerosis. Basic scientific research includes studies on full 360° coverage (2D), 3-axis rotational field (3D), and depth focusing.
10.5. Future directions
Building on the findings shared here, potential future investigations will explore the efficacy of the Deep TMS H7 Coil in cue-induced cravings in addictions other than AUD and CUD (e.g. opioid use disorder). Provided positive results in large FDA-regulated double-blinded randomized controlled trials, the labeling of the H7 Coil would expand to the treatment of addictions in general. This might imply a shared MOA in different addictions, and additional imaging and behavioral investigations could further shed light on it.
Future studies of PTSD are also warranted for a better understanding of the role of Deep TMS (targeting the DLPFC vs the mPFC/ACC, with the H1 Coil vs the H7 Coil, respectively) combined with SDI in treatment response. Given the unexpected results of the H7-coil study described above, a few potential directions would be investigating an inhibitory protocol (i.e., LF or cTBS), applying Deep TMS prior to SDI, as well as exploring the neural correlates of the clinical response.
Future sham-controlled studies may investigate the therapeutic potential of the H7 Coil, possibly applying sessions over several brain regions, in neurological disorders, such as stroke rehabilitation, autism, Parkinson’s, and Alzheimer’s diseases.
Deep TMS H7 Coil was tested and FDA-cleared in OCD patients who failed previous medication trials. Yet, it was found to be effective in patients with various degrees of resistivity, and the onset of the effect is much earlier than with medications, while its side effects are much milder. Hence, the efficacy of the H7 Coil should be tested also in drug-naïve patients.
Article Highlights
The H7 Coil stimulates in the medial prefrontal cortex and the anterior cingulate cortex.
Treatment with H7 Coil for OCD was cleared by the FDA following a large multicenter RCT.
Efficacy of H7 Coil was demonstrated in OCD patients with various degrees of severity and comorbidities.
Efficacy of H7 Coil was demonstrated in various kinds of addictions.
Results from various indications imply that a provocation that activates the relevant brain circuits prior to Deep TMS is important for clinical efficacy.
Declaration of Interest
T. Harmelech is a BrainsWay employee. A. Tendler is the Chief Medical Officer at BrainsWay and has financial interest in BrainsWay and ownership interest in Advanced Mental Health Care Inc. Y. Roth is the key inventor of Deep TMS and has financial interest in BrainsWay. The authors have no other relevant affiliations or financial involvement with any organization or entity with a financial interest in or financial conflict with the subject matter or materials discussed in the manuscript apart from those disclosed.
Reviewer Disclosures
Peer reviewers in this manuscript have no relevant financial or other relationships to disclose.
Additional information
Funding
References
- Tendler A, Barnea-Yigael N, Roth Y, et al. Deep transcranial magnetic stimulation (dTMS) – beyond depression. Expert Rev Med Devices. 2016;13(10):987–1000.
- Tzirini M, Roth Y, Harmelech T, et al. Electrical field measurements and simulations of the H7 and D-B80 Coils: Non-equivalence for obsessive compulsive disorder. .Brain Stimul. 2021;14(6):1525-1527. doi: https://doi.org/10.1016/j.brs.2021.10.382.
- Carmi L, Alyagon U, Barnea-Ygael N, et al., Clinical and electrophysiological outcomes of deep TMS over the medial prefrontal and anterior cingulate cortices in OCD patients. Brain Stimul. 2018;11(1): 158–165.
- Carmi L, Tendler A, Bystritsky A, et al., Efficacy and safety of deep transcranial magnetic stimulation for obsessive-compulsive disorder: a prospective multicenter randomized double-blind placebo-controlled trial. Am J Psychiatry. 2019;176(11): 931–938.
- Storch E, Tendler A, Schneider SC, et al. Moderators and predictors of response to deep transcranial magnetic stimulation for obsessive-compulsive disorder. J Psychiatr Res. 2021;136:508–514.
- Roth Y, Barnea-Ygael N, Carmi L, et al. Deep TMS for OCD is efficacious even in patients who failed multiple medications and CBT. Psychiatry Res. 2020;290:113179.
- Tendler A, Roth Y, Harmelech T. Deep repetitive TMS with the H7 coil is sufficient to treat comorbid MDD and OCD. Brain Stimul. 2021;14(3):658–661.
- Stein DJ, Costa DLC, Lochner C, et al. Obsessive–compulsive disorder. Nat Rev Dis Primers. 2019;5(1):52.
- Radua J, van Den Heuvel Oa, Surguladze S, et al. Meta-analytical comparison of voxel-based morphometry studies in obsessive-compulsive disorder vs. other anxiety disorders. Arch Gen Psychiatry. 2010;67:701–711.
- Gehring WJ, Goss B, Coles MG, et al. A neural system for error detection and compensation. Psychol Sci. 1993;4:385–390.
- Dehaene S. The error-related negativity, self-monitoring, and consciousness. Perspectives Psychol Sci. 2018;13:161–165.
- Holroyd CB, Dien J, Coles MG. Error-related scalp potentials elicited by hand and foot movements: evidence for an output-independent error-processing system in humans. Neurosci Lett. 1998;242:65–68.
- Falkenstein M, Hoormann J, Christ S, et al. ERP components on reaction errors and their functional significance: a tutorial. Biol Psychol. 2000;51:87–107.
- Holroyd CB, Coles MGH. The neural basis of human error processing: reinforcement learning, dopamine, and the error-related negativity. Psychol Rev. 2002;109:679–709.
- Botvinick M, Braver T. Motivation and cognitive control: from behavior to neural mechanism. Annu Rev Psychol. 2015;66:83–113.
- Brem S, Hauser TU, Iannaccone R, et al. Neuroimaging of cognitive brain function in pediatric obsessive–compulsive disorder: a review of literature and preliminary meta-analysis. J Neural Transm. 2012;119:1425–1448.
- Endrass T, Schuermann B, Kaufmann C, et al. Performance monitoring and error significance in patients with obsessive–compulsive disorder. Biol Psychol. 2010;84:257–263.
- Chamberlain SR, Blackwell AD, Fineberg NA, et al. The neuropsychology of obsessive–compulsive disorder: the importance of failures in cognitive and behavioral inhibition as candidate endophenotypic markers. Neurosci Biobehav Rev. 2005;29:399–419.
- Hajcak G, Moser JS, Yeung N, et al. On the ERN and the significance of errors. Psychophysiology. 2005;42:151–160.
- Hajcak G, Simons RF. Error-related brain activity in obsessive–compulsive undergraduates. Psychiatry Res. 2002;110:63–72.
- Saxena S, Brody AL, Ho ML, et al. Cerebral metabolism in major depression and obsessive—compulsive disorder occurring separately and concurrently. Biol Psychiatry. 2001;50:159–170.
- Tzur G, Berger A. When things look wrong: theta activity in rule violation. Neuropsychologia. 2007;45:3122–3126.
- Yeung N, Botvinick MM, Cohen JD. The neural basis of error detection: conflict monitoring and the error-related negativity. Psychol Rev. 2004;111:931–959.
- Kerns JG. Anterior cingulate and prefrontal cortex activity in an FMRI study of trial-to-trial adjustments on the Simon task. Neuroimage. 2006;33:399–405.
- Veale DM, Sahakian BJ, Owen AM, et al. Specific cognitive deficits in tests sensitive to frontal lobe dysfunction in obsessive-compulsive disorder. Psychol Med. 1996;26:1261–1269.
- Cavanagh JF, Zambrano-Vazquez L, Allen JJ. Theta lingua franca: a common mid-frontal substrate for action monitoring processes. Psychophysiology. 2012;49(2):220e38.
- Modirrousta M, Meek BP, Sareen J, et al. Impaired trial-by-trial adjustment of cognitive control in obsessive compulsive disorder improves after deep repetitive transcranial magnetic stimulation. BMC Neurosci. 2015;16:63.
- Gehring WJ, Himle J, Nisenson LG. Action-monitoring dysfunction in obsessive-compulsive disorder. Psychol Sci. 2000;11:1–6.
- Luu P, Tucker DM, Derryberry D, et al. Electrophysiological responses to errors and feedback in the process of action regulation. Psychol Sci. 2003;14:47–53.
- Fitzgerald KD, Welsh RC, Gehring WJ, et al. Error-related hyperactivity of the anterior cingulate cortex in obsessive compulsive disorder. Biol Psychiatry. 2005;57:287–294.
- Fitzgerald KD, Stern ER, Angstadt M, et al. Altered function and connectivity of the medial frontal cortex in pediatric obsessive-compulsive disorder. Biol Psychiatry. 2010;68:1039–1047.
- Carrasco M, Harbin SM, Nienhuis JK, et al. Increased error-related brain activity in youth with obsessive–compulsive disorder and unaffected siblings. Depress Anxiety. 2013;30:39–46.
- Riesel A, Endrass T, Kaufmann C, et al. Overactive error-related brain activity as a candidate endophenotype for obsessive–compulsive disorder: evidence from unaffected first-degree relatives. Am J Psych. 2011;168:317–324.
- Luu P, Tucker DM, Makeig S. Frontal midline theta and the error-related negativity: neurophysiological mechanisms of action regulation. Clin Neurophysiol. 2004;115:1821–1835.
- Cohen MX, Elger CE, Ranganath C. Reward expectation modulates feedback-related negativity and EEG spectra. Neuroimage. 2007;35:968–978.
- Mesika D, Tzur G, Berger A. Electrophysiological evidence for automatic processing of erroneous stimuli. Neuropsychologia. 2014;59:85–92.
- Carmi L, Alyagon U, Barnea-Ygael N, et al. From self-induced to perceived errors - A generalized over-monitoring activity in obsessive-compulsive disorder. Eur Neuropsychopharmacol. 2019;29:1083–1091.
- Saxena S, Gorbis E, O’Neill J, et al. Rapid effects of brief intensive cognitive-behavioral therapy on brain glucose metabolism in obsessive-compulsive disorder. Mol Psychiatry. 2009;14:197–205.
- Reddy S, Goyal N, Shreekantiah U. Adjunctive deep transcranial magnetic stimulation (dTMS) in obsessive compulsive disorder: findings from 1 H magnetic resonance spectroscopy. Asian J Psychiatr. 2021;62:102721.
- Tendler A, Sisko E, Garrison S, et al. Initial report on long-term durability of deep TMS for obsessive compulsive disorder. Brain Stimul. 2020;13:1844.
- Gomez-Tames J, Hamasaka A, Hirata A, et al. Group-level analysis of induced electric field in deep brain regions by different TMS coils. Phys Med Biol. 2020;65:025007.
- Alyagon U, Barnea-Ygael CL, Zangen A. Modifications of cognitive performance in the Stroop task following deep rTMS treatment course in OCD patients. Brain Stimul. 2021;14:48–50.
- Roth Y, Tendler A, Arikan MK, et al. Real-world efficacy of deep TMS for obsessive-compulsive disorder: post-marketing data collected from twenty-two clinical sites. J Psychiatr Res. 2021;137:667–672.
- Harmelech T, Tendler A, Roth Y, et al. Do Comorbid OCD-MDD patients need two separate dTMS protocols. Brain Stimul. 2020;13(4):1000–1001.
- Tendler A, Sisko E, Rodriguez N. DMPFC-ACC DTMS for refractory body dysmorphic disorder: case report. Brain Stimul. 2017;10:e21–e45.
- Avirame K, Stehberg J, Todder D. Enhanced cognition and emotional recognition, and reduced obsessive compulsive symptoms in two adults with high-functioning autism as a result of deep Transcranial Magnetic Stimulation (dTMS): a case report. Neurocase. 2017;23(3–4):187–192.
- Enticott PG, Fitzgibbon BM, Kennedy HA, et al. A double-blind, randomized trial of deep repetitive transcranial magnetic stimulation (rTMS) for autism spectrum disorder. Brain Stimul. 2014;7:206–211.
- Camchong J, Stenger A, Fein G. Resting-state synchrony during early alcohol abstinence can predict subsequent relapse. Cereb Cortex. 2013;23:2086–2099.
- Fede SJ, Grodin EN, Dean SF, et al. Resting state connectivity best predicts alcohol use severity in moderate to heavy alcohol users. NeuroImage Clin. 2019;22:101782.
- Durazzo TC, Meyerhoff DJ. Changes of frontal cortical subregion volumes in alcohol dependent individuals during early abstinence: associations with treatment outcome. Brain Imaging Behav. 2020;14(5):1588–1599.
- Peters J, Kalivas PW, Quirk GJ. Extinction circuits for fear and addiction overlap in prefrontal cortex. Learn Mem. 2009;16:279–288.
- Schacht JP, Anton RF, Myrick H. Functional neuroimaging studies of alcohol cue reactivity: a quantitative meta-analysis and systematic review. Addict Biol. 2013;18(1):121–133.
- Wandres M, Pfarr S, Molnár B, et al. Alcohol and sweet reward are encoded by distinct meta-ensembles. Neuropharmacology. 2021;195:108496.
- Martinez D, Urban N, Grassetti A, et al. Transcranial magnetic stimulation of medial prefrontal and cingulate cortices reduces cocaine self-administration: a pilot study. Front Psychiatry. 2018;9:80.
- Harel M, Barnea-Ygael N, Shalev H, et al. Deep transcranial magnetic stimulation over the medial prefrontal and anterior cingulate cortex alters brain connectivity and reduces relapses to alcohol use. Biol Psychiatry. 2019;85(10):S108.
- Harel M, Perini I, Kämpe R et al, et al. Repetitive transcranial magnetic stimulation in alcohol dependence: a randomized, double-blind, sham-controlled proof-of-concept trial targeting medial prefrontal and anterior cingulate cortex Biological Psychiatry 2022 doi:https://doi.org/10.1016/j.biopsych.2021.11.020 . . *RCT in 51 alcoholic patients demonstrating high efficacy of H7 Deep TMS and clinical outcomes were associated with brain connectivity alterations reflected in fMRI.
- Tendler A, Sisko E, Barnea-Ygael N, et al. Antidepressant remission to dTMS of the dmPFC and ACC in lateral PFC dTMS non-responders: case series. Brain Stimul. 2017;10:714–715.
- Isserles M, Shalev AY, Roth Y, et al. Effectiveness of deep transcranial magnetic stimulation combined with a brief exposure procedure in post-traumatic stress disorder–a pilot study. Brain Stimul. 2013;6:377–383.
- Isserles M, Tendler A, Roth Y, et al.Deep transcranial magnetic stimulation combined with brief exposure for post-traumatic stress disorder – a prospective multisite randomized trial. Biol Psychiatry. 2021;S0006-3223(21): 01264–6. https://doi.org/10.1016/j.biopsych.2021.04.019. Online ahead of print
- Popa T, Morris LS, Hunt R, et al. Modulation of resting connectivity between the mesial frontal cortex and Basal Ganglia. Front Neurol. 2019 Jun 5;10:587.
- Soomro GM, Altman D, Rajagopal S, et al. Selective serotonin re-uptake inhibitors (SSRIs) versus placebo for obsessive compulsive disorder (OCD). Cochrane Database Syst Rev. 2008;1:CD001765.
- Luber BM, Davis S, Bernhardt E, et al. Using neuroimaging to individualize TMS treatment for depression: toward a new paradigm for imaging-guided intervention. Neuroimage. 2017;148:1–7.
- Isserles M, Rosenberg O, Dannon P, et al. Cognitive-emotional reactivation during deep transcranial magnetic stimulation over the prefrontal cortex of depressive patients affects antidepressant outcome. J Affect Disord. 2011;128:235–242.
- Dinur-Klein L, Dannon P, Hadar A, et al. Smoking cessation induced by deep repetitive transcranial magnetic stimulation of the prefrontal and insular cortices: a prospective, randomized controlled trial. Biol Psychiatry. 2014;76:742–749.
- Dudai Y. The neurobiology of consolidations, or, how stable is the engram? Ann Rev Psychol. 2004;55:51–86.
- Dudai Y. Reconsolidation: the advantage of being refocused. Curr Opin Neurobiol. 2006;16:174–178.
- Ledoux JE, Muller J. Emotional memory and psychopathology. Philos Trans R Soc Lond B Biol Sci. 1997;352:1719–1726.
- Olatunji B, Sarawgi S, Viar-Paxton M. An initial test of reconsolidation in disgust-related learning and extinction. J Cognit Psychotherapy. 2016;30:190–202.
- Greco JA, Liberzon I. Neuroimaging of fear-associated learning. Neuropsychopharmacology. 2016;41(1):320–334.
- Zibman S, Daniel E, Alyagon U, et al. Interhemispheric cortico-cortical paired associative stimulation of the prefrontal cortex jointly modulates frontal asymmetry and emotional reactivity. Brain Stimul. 2019;12:139–147.
- Chiappini E, Silvanto J, Hibbard PB, et al. Strengthening functionally specific neural pathways with transcranial brain stimulation. Curr Biol. 2018;28:R735–6.
- Meyer V. Modification of expectations in cases with obsessional rituals. Behav Res Ther. 1966;4:273–280.
- Roth Y, Pell GS, Barnea-Ygael N, et al. Rotational field TMS: comparison with conventional TMS based on motor evoked potentials and thresholds in the hand and leg motor cortices. Brain Stimul. 2020;13:900–907.
- Roth Y, Levkovitz Y, Pell GS, et al. Safety and characterization of a novel multi-channel TMS stimulator. Brain Stimul. 2014;7:194–205.