ABSTRACT
Introduction
Hyaluronan (HA)-based soft-tissue fillers are injectable crosslinked hydrogels aimed to counteract facial skin aging signs via minimally invasive procedures. The crosslinking step is required to drastically improve HA residence time in vivo and provide the gel with specific viscoelastic properties matching the clinical indications. While HA as a raw material and HA fillers are widely studied, little is reported about crosslinkers themselves used in commercial fillers.
Areas covered
This article introduces the specifications of the ideal crosslinker in HA fillers. The properties of commercially used crosslinkers are reviewed. An up-to-date review of innovative hydrogel fabrication alternatives is conducted, and advantages and drawbacks are discussed.
Expert opinion
HA fillers are predominantly manufactured using 1,4-butanediol diglycidyl ether (BDDE) which is considered as the gold standard crosslinker worldwide due to its proven and unrivaled clinical track record of more than 20 years. Extensive studies have been published covering BDDE-crosslinked HA fillers’ chemistry, gel properties, and clinical effectiveness and safety. However, new hydrogel fabrication strategies have emerged, paving the way for innovative alternatives potentially bringing novel features to HA fillers. Nevertheless, major efforts must still be implemented to assess their safety, efficacy, stability and suitability for industrialization.
1. Introduction
The use of injectable soft-tissue fillers has become increasingly popular in esthetic medicine because it offers an opportunity for facial rejuvenation, as well as plastic and reconstructive improvements via minimally invasive procedures [Citation1–4]. As long-term resorbable implantable gels, they are classified as class III medical devices by most Regulatory Authorities. Fillers are substances that are injected within or beneath the skin layers in order to restore lost volume, smooth lines, soften creases, or enhance facial contours. The earliest forms of fillers included permanent silicone or polymethacrylate implants (the former having been withdrawn in several countries by competent bodies), and resorbable collagen-based fillers [Citation2,Citation5,Citation6]. The use of these products has raised safety concerns due to side effects such as overcorrection, inflammatory responses such as late-onset granulomatous reactions [Citation7], and the need for prior allergy testing. This has therefore led to the search for safer soft-tissue fillers [Citation5,Citation8,Citation9]. More recently, Hyaluronic Acid (HA)-based gels have become the gold standard for soft-tissue filling, thanks to their long safety records, biocompatibility, and low toxicity and immunogenicity profiles, as well as their versatility of applications in biomedicine and hydrogel design [Citation10].
HA is a linear, naturally occurring polysaccharide, belonging to the class of biochemical compounds referred to as glycosaminoglycans. It is one of the primary components of the extracellular matrix and is notably found in synovial fluids and epithelial and connective tissues of vertebrates [Citation11,Citation12]. A 70 kg human body endogenously contains about 15 g of HA and approximately half the quantity is found in skin layers [Citation13,Citation14]. Its primary structure consists of a repeating carbohydrate sequence: [D-glucuronic acid β(1→3) N-acetyl-D-glucosamine β (1→4)]n [Citation15]. Owing to its high molecular weight, it exhibits many unique physicochemical and mechanical properties, such as regulation of inflammation, hygroscopicity, and lubrication [Citation11,Citation16].
Despite its unique biological properties, native HA is known to have poor residence time with a very short half-life in skin of about 2–4 days [Citation17,Citation18], as it degrades rapidly by scission of the glycosidic linkages via the action of endogenous hyaluronidase and reactive oxygen species [Citation19,Citation20]. Moreover, it has poor elastic properties, limiting its direct use as filler because it is not itself able to lift tissues. Therefore, there is a need to overcome these limitations by modifying the structure of HA chains, aiming to obtain HA gels with prolonged residence time and enhanced viscoelastic properties. In fine, an ideal HA gel should be able to lift tissues in a long-term and reproducible way, easily accompany dynamic expressions, exhibit good tissue integration and cohesivity, while remaining easy to inject and biocompatible. One way to achieve these specifications is through chemical modifications and crosslinking [Citation21]. These result in mechanically and chemically stable materials with improved viscoelastic properties when compared to the native polymer, but which maintain the biocompatibility of the raw material and remain resorbable . Among the different strategies to synthesize a HA gel, the use of crosslinkers, which are generally small molecules consisting of a spacer and at least two functional groups designed to bridge HA chains, is the most widely used approach to provide HA with clinically relevant properties.
Through this review, several questions are approached: what are the ideal crosslinker’s specifications? Which are the crosslinkers commonly used in HA-based soft-tissue fillers and what are their properties? And finally, what are the alternatives, their advantages and their drawbacks? This review is primarily based on a comprehensive review of crosslinking agents used for HA-based fillers. In order to provide a focused review, innovative alternatives for the design of HA gels were selected among biomedical applications, but the authors chose to focus on the discussions that are the most relevant to the clinical practitioner.
2. What are the specifications of the ideal crosslinker?
In HA-based fillers, the crosslinker has a prevailing role of linking HA chains together, thus giving rise to necessary elastic properties and projection capacity, as well as the property of being long-lasting by slowing the enzymatic degradation kinetics. In order to be effective in the crosslinking medium, as well as for the crosslink to be stable and safe over time, the crosslinker must meet a drastic list of requirements reducing the list of eligible molecules.
In terms of chemistry, as HA is highly sensitive to pH or temperature for instance [Citation19], the first prerequisite is that the crosslinking reaction should occur rapidly and in high yields in mild aqueous media to limit the degradation of HA and achieve efficient crosslinking. The second requirement is aqueous solubility. Since the resulting gels are intended for injection, the crosslinking reaction – and the manufacturing process – must occur, as much as possible, in aqueous solutions to avoid any traces of organic solvent in the injected gel. Third, the crosslinker must ensure an excellent stability of the gel ex vivo to ensure a long shelf-life, and therefore the formed bonds – mostly covalent – should remain stable over a range of storage temperatures. Finally, the amount of crosslinker used in dedicated manufacturing steps will determine the viscoelastic behavior of the final gel.
In terms of toxicity, the crosslinker, as it must react readily with HA, bears at least two electrophilic functional groups, which could be an inherent source of cell toxicity of the raw material. Therefore, the prerequisite for using a crosslinker in an injectable gel is its complete degradation to safe and inert products under reaction conditions. Any unreacted crosslinker must readily hydrolyze into safe by-products. The purification steps must eliminate any trace of the crosslinker raw material as well as its hydrolysis by-products, even if the latter are expected to be far less troublesome. The crosslinking structure must be metabolized to harmless byproducts during resorption of the gel. Moreover, the crosslinker should be used in limited amounts.
Finally, in terms of safety, the HA recognition sites (carboxyl moieties), and cleavage sites (glycosidic bonds) must nonetheless be preserved as much as possible, so as not to completely prevent HA enzymatic catabolism and thus gel resorption over time, thereby minimizing the risk of delayed adverse events. Rapid enzymatic degradation is also required in case of emergency (e.g. management of vascular occlusion) or remodeling (retouch), using a proper amount of the HA specific degradation enzyme family: hyaluronidases. Moreover, the crosslinker structure may also impact gel properties: a polyfunctional crosslinker may lead to a more difficult degradation [Citation22], whilst a long and hydrophilic crosslinker backbone may result in undesirably large swelling properties [Citation23].
To date, only a limited number of crosslinkers have met this list of specifications conditioning the design of safe HA-based fillers. A review of these commercially used crosslinkers is presented.
3. Commonly available chemical crosslinkers for fillers
HA is a polyfunctional biomolecule, and its chemical modifications therefore target the available functional groups of the disaccharide subunit: one carboxyl group and four hydroxyl groups on the native HA, or a free amino group that can be accessed by deacetylation of the N-acetyl glucosamine or two aldehydes produced by oxidative cleavage of the glucuronic acid ring.
3.1. Brief chronology
1,4-butanediol diglycidyl ether (BDDE, the first linker from the bisepoxide family) was the first crosslinker to be used in a commercially available HA-based filler. This filler, Restylane, was introduced in Europe in 1996 and FDA-approved in 2003 and is still sold worldwide [Citation24–26]. Divinylsulfone (DVS)-crosslinked HA fillers Hylaform (HA from rooster comb) and Captique were introduced on the US market in 2004, while a p-phenylene biscarbodiimide (BCDI)-crosslinked HA filler Hydrelle (formerly named Elevess) was FDA-approved in 2006; none of these remain on the market. Diepoxyoctane (DEO, the second linker in the bisepoxide family)-crosslinked HA fillers were introduced in Europe in 2005 under the name Puragen [Citation27] but are also no longer commercialized. Finally, polyethyleneglycol diglycidyl ether (PEGDE, the third linker from the bisepoxide family)-crosslinked HA fillers were recently introduced in Europe in 2012 within the Neauvia product line.
Only BDDE-crosslinked HA fillers are represented today among the approximately 20 HA-based fillers currently marketed in the US. The same trend is observed among fillers approved on the Chinese market as of 2019 [Citation28]. Currently in Europe, about 30 international manufacturers commercialize filler product lines with BDDE as a crosslinker. To the best of our knowledge, only two product lines are DVS-crosslinked, and one product line is PEGDE-crosslinked [Citation29].
3.2. Chemistry
The chemistry of commercially used crosslinkers’ functional groups is detailed in this section, as summarized in .
Figure 1. Synthetic pathways of the crosslinking reaction used for the fabrication of commercial soft-tissue fillers.
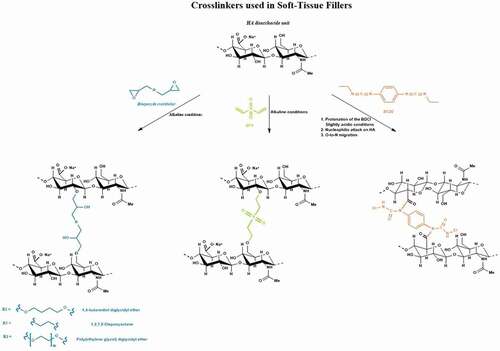
3.2.1. Bisepoxides
Bisepoxides are a class of chemical crosslinkers for HA in which the epoxide functionalities serve as electrophiles for the Williamson etherification via ring opening [Citation12]. As mentioned earlier, the different bisepoxides used as crosslinkers for HA include BDDE, PEGDE, and DEO. Under strong alkaline conditions (usually at pH 13), bisepoxides react with the hydroxyl groups on the HA chains to form ether linkages as shown in [Citation30]. Generally, temperatures higher than 40°C over a few hours of reaction are reported [Citation31,Citation32]. This leads to the modification of hydroxyl groups at different positions, not necessarily on the primary alcohol [Citation33,Citation34]. The resulting bridge between both HA chains creates an elastic crosslinked HA hydrogel network. Typically, degrees of modification (MoD), commonly measured by 1H NMR, are reported between 1 and 10% for BDDE-crosslinked HA fillers [Citation35,Citation36], the MoD being defined as the molar ratio of crosslinker to HA disaccharide units. This parameter represents the total HA modification, although the crosslinker may be either linked to a single HA strand, or may effectively crosslink two HA chains [Citation36].
3.2.2. Divinyl sulfone
DVS is another crosslinker of interest used to chemically modify the structure of HA into stable three-dimensional hydrogel networks for various biomedical applications, including soft tissue augmentation [Citation37]. DVS is a bifunctional electrophile that crosslinks HA in alkaline medium via ether bond formation between the hydroxyl groups of the HA and the double bonds of DVS via a Michael-type addition (). Under strongly alkaline conditions, the alkaline medium induces the deprotonation of hydroxyl groups on the HA chains to form alkoxy ions, which react with the electron deficient vinyl groups of DVS to form ether bonds, resulting in a five-atom sulfonyl-bis-ethyl bridge between two HA chains [Citation38].
3.2.3. Biscarbodiimide
BCDI can also be used as a bifunctional crosslinker to directly crosslink adjacent HA chains. In this technique, the carboxyl functionality on the HA main chain is utilized under mildly acidic medium to form a stable HA acylurea with the biscarbodiimide on both ends in the absence of a nucleophile (such as amines) or any other polyanionic polysaccharide other than HA itself [Citation39]. The reaction proceeds by protonation of the carbodiimide, followed by attack of the carboxylate anion on the carbon atom of the BCDI to form an O-acylisourea intermediate. In acidic medium, the intermediate then undergoes O-to-N migration to form the more stable N-acylurea depicted in . This reaction is carried out in the presence of a pH buffer usually between pH 4.5 and 5.5. One of the specifications of this method is keeping the pH of the medium within the required range as carbodiimides are unstable at lower pH values while the reaction rate is diminished [Citation40]. Since the rearrangements at both ends of the biscarbodiimide are non-concerted, the O-to-N migration can be incomplete which would result in a mixture of N-acylurea at both ends, a mixture one N-acylurea and one O-acylisourea at either end, and O-acylisourea at both ends.
3.3. Manufacturing process related to crosslinkers
3.3.1. Properties of crosslinkers used for marketed gels
Between its functional groups, the crosslinker bears a spacing backbone that has a prevailing role during the gel manufacturing. Indeed, the solubility of the crosslinker in aqueous media, mainly due to the spacer physico-chemical properties, must be sufficient to avoid precipitation during manufacturing and promote efficient chemical bonding with HA. To meet this criterion, molecules with polar functionality are preferred. For instance, BDDE and DVS both have a LogP of 0.4 conferring sufficient miscibility in water despite the presence of central butanediol or divinyl chains, respectively (). PEGDE is a hydrophilic polymer which has a size distribution (dispersity) centered around a mean value of several hundreds of daltons, in other words, it is a mixture of oligomers, which differentiate PEGDE from all the other commercially used crosslinkers. PEGDE has a negative LogP value that indicates high-water solubility and also an increased capacity to adsorb water. On the other hand, the less soluble crosslinker is BCDI has a LogP of 2.4. The poor water solubility of this crosslinker due to the presence of a phenyl ring generally leads to the use of organic co-solvents during the crosslinking step that requires extensive purification and characterization of the resulting gel.
Table 1. Summary of the commercially used crosslinkers physico-chemical properties
3.3.2. Purification process
Once the chemical modification of HA chains has occurred, the next step involves the purification of the resulting 3D network. Information about this step is often confidential and specific to each manufacturer. It is nevertheless of paramount importance to guarantee product safety. Dialysis remains the gold standard technique to purify HA gels with the use of a semipermeable membrane to selectively separate unwanted molecules such as crosslinker traces [Citation41]. Another benefit of the dialysis is regulation of the pH of the hydrogel matrix and the opportunity for ion exchange to homogenize the ionic strength to obtain isotonic formulations suitable for injection. The disadvantages of dialysis are its long duration and the large volume of aqueous solution required for the bath where the gel contained in the membrane is immersed due to the slow diffusion of solutes within the gel network. Precipitation of the materials with organic solvents may be carried out and followed by repetitive washing, generally on laboratory scale, to remove unreacted crosslinking reagents. For instance, PEGDE-crosslinked HA gels might be washed with acetonitrile instead of water to limit excessive gel swelling as PEGDE-crosslinked gels tend to swell more than BDDE-crosslinked gels [Citation23].
3.3.3. Shelf life or expiration dating
After the sterilization step, final packaging, and quality controls, release specifications of HA gels must be stable over time to ensure they can be safely used throughout their shelf-life, i.e. gel’s properties must remain suitable for their intended use. As medical devices, HA soft-tissue fillers are required to be labeled with an expiration date that is supported by stability studies determining product’s shelf-life. It is noteworthy that stability of the final gels depends on the formulation itself i.e. the stability of the covalently linked crosslinker and on the packaging. Most crosslinked HA fillers on the market claim a shelf-life of 2 years upon storage at temperatures ranging between 2 and 25°C, without remarkable differences introduced by the industrially used crosslinkers.
3.4. Degradation/hydrolysis, metabolism, and toxicity
HA itself has a rapid turnover of 2–4 days in skin [Citation18] due to the activity of a specific family of enzymes named hyaluronidases [Citation42] and the local production of reactive oxygen species after gel injection. Crosslinking HA was found to be an effective way to significantly reduce the rate of degradation of HA and thus to develop long-lasting dermal fillers [Citation43,Citation44]. As a result, crosslinked HA fillers can last from a few weeks to several months, while remaining nonetheless resorbable. The preservation of HA natural recognition or cleavage sites during the crosslinking guarantees resorption of the HA gel over time as well as quick dissolution through hyaluronidase injection in case of emergency (e.g. vascular occlusion) or of need for retouching.
3.4.1. BDDE/bisepoxides
The use of BDDE to crosslink HA chains and create an elastic network slows its enzymatic degradation, presumably by obstructing hyaluronidase access to cleavage sites, but does not prevent it altogether, since glycosidic bonds remain available for enzymatic cleavage, as they are unaffected by the covalent ether bond formed between BDDE and HA hydroxyl groups [Citation45].
During the manufacturing process, the epoxide groups, which need to be highly reactive in order to effectively crosslink HA, bring inherent toxicity to native BDDE as a raw material. According to safety data sheets provided for BDDE as a raw material, exposure to BDDE may induce irritation and allergic reactions at greater concentrations than the safety threshold of 2 ppm. As regulated medical device, fillers are assessed in terms of biocompatibility according to ISO 10993 standard, which requires, among others, a full biological evaluation including genotoxicity, carcinogenicity, and reproductive toxicity testing (ISO 10993–3) and evaluation of long-term local tissue effects after implantation (ISO 10993–6). Residual BDDE is expected to be nearly absent and in any case well below the toxicity threshold of 2 ppm in the final gel. BDDE readily reacts either with HA hydroxyl groups or with water of the reaction medium during the crosslinking step. Thus, BDDE which does not react with HA undergoes fast degradation through hydrolysis under alkaline reaction conditions [Citation46,Citation47]. Eventual trace amounts of residual crosslinker present after crosslinking will thus continue to hydrolyze later in the manufacturing process until complete deactivation in the form of hydrolyzed BDDE (a diol-ether form) which has been shown to be nontoxic and nongenotoxic at the low concentrations met in fillers [Citation45]. An extensive purification process will nonetheless serve to remove trace amounts of unreacted BDDE and all other unbound hydrolysis by-products, so that the common acceptable limit for residual BDDE below 2 ppm is achieved in the finished products. Unless the manufacturer has poor control over the manufacturing process of a crosslinked-HA gel, there should be no BDDE left beyond this limit in the finished product. The limit of 2 ppm was originally set as part of Restylane toxicological assessment [Citation45], which deemed the cancer risk to be minimal for patients injected as per the product intended use. The rationale was subsequently accepted by the Food and Drug Administration and the BDDE specification of 2 ppm was extensively re-used for other marketed HA fillers for minimal cancer risk [Citation48]. As an illustration, open access FDA Summary of Safety and Effectiveness Data sheets confirm that batch release specifications set by all manufacturers of FDA-approved HA fillers require a BDDE residual content inferior to 2 ppm. Using novel analytical techniques, HA filler manufacturers progressively lowered their detection limit below this historical limit. For instance, a systematic control on every batch of Teosyal RHA® products is performed to ensure that BDDE, as a raw material, is well below the specification of 1 ppm. In general practice, Teosyal RHA® products present BDDE residual traces that are below the limit of detection of the chromatographic method set at 0.2 ppm.
Once implanted in vivo, BDDE derivatives are either crosslinked to 2 HA chains or monolinked to 1 HA chain, leaving a hydrolyzed and inert pendant form. The ether bonds which are rather stable bonds, thus providing long-lasting properties to the fillers, can nevertheless be cleaved by a family of enzymes of the cytochromes P450 family over time [Citation45]. On this subject, an in-depth review of BDDE-crosslinked HA filler metabolism presents the enzymatic degradation cascade [Citation45]. The sites of the crosslinker susceptible to degradation are the ether bonds between HA and the reacted BDDE as well as the ether bonds on the reacted BDDE backbone, resulting in 1,4 butanediol and glycerol, both of which do not pose any safety risk at observed concentrations and are further naturally eliminated via the Krebs cycle or by glycolysis, respectively [Citation45].
The other bisepoxides employed in fillers, 1,2,7,8- Diepoxyoctane (DEO) and polyethylene glycol diglycidyl ether (PEGDE), have similar properties and reactivity to BDDE since the functional epoxide groups are identical. PEG is used extensively in pharmaceutical sciences to improve drug bioavailability via nanocarriers or prodrugs. This polymer is known to be eliminated primarily in urine as is, due to its low Mw, once it detaches from HA.
The toxicity of crosslinkers essentially arise from the reactive epoxide groups that are required to effectively crosslink HA. As a result, these reactive sites should be effective during the crosslinking step but then be degraded and eliminated after the purification step, independently of the spacer linking the epoxide moieties. As an illustration, the DL50 reported on the safety data sheets provided for current commercially available bis-epoxide crosslinkers, except for PEGDE that was not available, exhibit very similar toxicities after oral administration to rats, around 1 g/kg. Moreover, a recent article assessed the toxicity of BDDE and PEGDE directly as raw materials on keratinocytes and fibroblasts. The authors showed no toxic cellular responses for either crosslinkers at concentrations below 25 ppm, which represents a concentration 12 times higher than the release specification usually reported in the literature for dermal fillers [Citation49]. Moreover, manufacturing processes are increasingly reviewed by authorities and, as analytical methods are getting more efficient, potential by-products, even obtained under harsh conditions, are systematically monitored [Citation50]. Manufacturers document and evaluate any possible and unwanted molecules through the development phase of the medical devices design verification and implement release specifications in line with the regulatory authority if necessary to guarantee a safe final product.
When considering HA fillers in medical practice, the toxicity of BDDE (or other bisepoxides) as native crosslinker is thus a much less relevant consideration than that of reacted/hydrolyzed forms effectively present in the final gel, along with their released metabolites.
3.4.2. Divinyl sulfone
DVS, as a raw material, was proven to be toxic with a IC50 on human colorectal carcinoma cells of 34 μM due to protein alkylation and protein crosslinking [Citation51]. The safety data sheet of this crosslinker shows a lower DL50 than bisepoxides, around 30 mg/kg after oral administration in rats. No minimum acceptable limit for residual DVS has been reported in the literature, but one can expect a lower threshold than for BDDE. Once reacted, DVS forms a diethyl sulfone group that did not cause any toxicity up to 10 mM in human colorectal carcinoma cells [Citation51].
3.4.3. Biscarbodiimide
The BCDI crosslinker has received limited attention in the literature [Citation52], and no toxicological data are available to the best of our knowledge. The main specificity of BCDI compared to bisepoxides or DVS is the location of its reaction site on HA carboxyl moieties forming an amide bond. Since the anionic carboxyl groups play a significant role in the charge/hydrophilicity of the HA chains and their biological recognition by hyaluronidase [Citation53], modifying COO-moieties by ester or amide functions reduces the enzymatic degradation of HA chains proportionally to the degree of modification [Citation11,Citation53,Citation54]. As a result, a limited amount of this crosslinker should be employed in the manufacturing of HA gels in order not to prevent their enzymatic degradability in case of an adverse event. Moreover, acylurea bonds are known to be less stable than ether bonds, thus the shelf-life stability of BCDI-crosslinked gels should be weaker than those met with bisepoxides and DVS-crosslinked gels.
3.5. Clinical and post-market surveillance data
In the US only, in 2019, minimally invasive cosmetic procedures involving hyaluronic acid soft-tissue fillers reached more than 2.6 million injections as reported by the American Society of Plastic Surgeons [Citation55]. Based on data extracted from clinicaltrials.gov on September 2020, more than 130 fully completed clinical trials evaluating the efficacy and safety of BDDE-crosslinked HA fillers have been reported, covering a large variety of indications. Overall, this represents about 12,000 patients worldwide enrolled in these trials, covering the whole panel of Fitzpatrick skin phenotypes and a broad range of ethnicities (The trials were selected using product names of HA-based soft-tissue fillers as keywords. All conditions that did not imply esthetic purposes were excluded). Given that clinical studies, particularly investigator-initiated trials or post-market follow-up studies, are not systematically recorded on databases, the actual number of trials and population sizes remain largely underestimated.
Wang et al. performed a systematic review and meta-analysis evaluating effectiveness and safety of HA fillers with lidocaine for the treatment of nasolabial folds (NLF), which has been one of the first treatment target of HA fillers and continue to be a ‘market-entry’ indication [Citation56]. They selected and analyzed 10 randomized clinical trials, gathering 723 patients injected with BDDE-crosslinked HA fillers. Beyond the evaluation of filler effectiveness, safety records were systematically reported. Regardless of the gel formulation, the investigators mainly reported common and mild adverse events such as swelling, erythema, bruising, itching, and induration [Citation56]. In another indication, Stojanovic et al. conducted a similar systematic review of the effectiveness and safety of BDDE-crosslinked HA fillers for lip augmentation [Citation57]. Based on data from 3965 patients enrolled in 22 clinical studies, HA turned out to be both effective and safe for lip fullness treatments, eliciting mostly mild and transient reactions at injection sites (swelling, contusion, bruising, pain, redness and itching).
While these minor and local reactions represent the majority of side effects emerging upon HA filler treatment, more serious adverse events (SAE) have been reported such as hypersensitivity reactions, late-onset inflammatory reactions (including granulomas), infections, or vascular compromises [Citation58]. None of these AE were ever proven to be related to residual traces of the HA-crosslinking agent. Hypothetical causes rather include immune or inflammatory responses directed against anesthetics included in the formula (cases of immediate reactions), or against HA degradation products released over time (case of delayed reactions), and – for vascular compromise – improper injection techniques causing a vessel occlusion [Citation59–61].
To the best of our knowledge, only one clinical trial involving 74 patients injected into their nasolabial folds with a DEO-crosslinked HA filler on the one side, and with a DVS-crosslinked HA filler on the contralateral side, has been reported [Citation62]. In spite of discrepancies in product effectiveness, both fillers induced comparable side effects in approximately 90% of subjects, which consisted in mild to moderate and short-lived reactions such as swelling, redness, itching, pain and hardening. Despite extensive clinical and scientific databases search, no clinical trial pertaining to PEGDE-crosslinked HA fillers could be retrieved.
As for other categories of crosslinkers, only one clinical trial evaluating a biscarbodiimide-crosslinked HA filler in 100 patients was retrieved on clinical trial.gov (NCT01147172), which reported mostly expected treatment reactions (e.g. injection site discoloration, induration, nodule, erythema, etc.) and no serious adverse event. Finally, 6 clinical trials evaluating esthetic treatments performed with DVS-crosslinked HA fillers in 370 patients were identified, including the aforementioned split-face trial with a DEO-crosslinked filler on the contralateral side. One other study (NCT00444626) had posted results on clinicaltrials.gov, which suggested that the biscarbodiimide-crosslinked filler had a similar safety profile as the control BDDE-crosslinked filler, as evaluated following spit-face injection in the NLF of 140 patients.
Though this is not an exhaustive selection as not all clinical trials are duly reported or published, it provides a large quantity and high-level of evidence of the clinical safety of BDDE-crosslinked HA gels, based on data gathered throughout their 25-year period of use. Albeit more scarcely evaluated, commercially available HA fillers manufactured with alternative crosslinkers seem to present comparable safety profiles.
4. Alternatives
Finding innovative approaches to preparing HA-based hydrogel networks that respect regulatory requirements to manufacture safe and clinically relevant products is a significant challenge for the soft-tissue filler industry. Many reviews have been recently published about different strategies to crosslink HA, proving that a broad range of techniques already exist to fabricate HA networks. Nonetheless, the spectrum of conditions and linkers used in the industry of soft-tissue fillers remains very narrow, in part because of the lack of long-term safety record of alternatives [Citation10,Citation16,Citation63]: Any novelty must be submitted to extensive time and cost-consuming biocompatibility and stability studies as well as toxicological assessments.
The strategies can be divided into three categories (): 1) direct crosslinking of native HA is the most straightforward approach, which is the currently employed method in the soft-tissue filler field. 2) A second approach is to modify the HA chain first, either by deacetylation to liberate the free amine, by oxidation to free up a dialdehyde functionality, or by modification with a linker bearing new functional groups. It is still necessary to remove all residual traces of the crosslinker plus the modifying linkers but there are considerable process advantages to this approach such as crosslinking in milder conditions or bringing new functionalities to the hydrogel network. 3) Finally, a crosslinker-free approach using the conjugation technique consists in crosslinking two samples of HA that are previously derivatized with different groups and can then react with each other under mild conditions.
Figure 2. Summary of the HA hydrogel fabrication strategies (A) starting from native HA and a crosslinker, (B) starting from a modified version of HA and a crosslinker, and (C) using the conjugation technique.
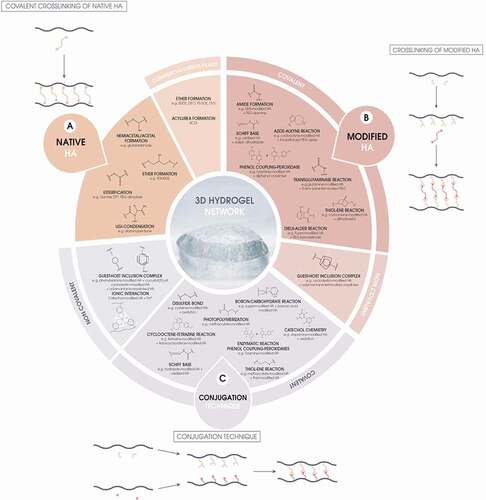
also represents the different chemical routes which may be employed for the fabrication of HA hydrogels highlighting the limited area of crosslinkers used in commercial fillers. The main differences between the different approaches reside in process issues and delivery methods rather than in toxicity since the functional groups are often identical. In all cases, the active functionalities have to be completely depleted during the reaction, and any traces of unbound reagents can be removed completely from the final gel thanks to extensive purifications. However, the metabolic fate of the crosslinked structure during resorption of the filler will have to be considered and thoroughly studied.
4.1. Covalent crosslinking of native HA
In order to design new crosslinkers for native HA, based on what is currently marketed by manufacturers, two directions are possible: changing the crosslinker functional groups to use new chemical routes () or changing the crosslinker spacer to modify the physico-chemical properties of the molecule as well as its functionality. As observed in , a limited number of condensation reactions have been reported in the literature as an alternative to etherification by epoxide opening. Beyond the very classical crosslinking with glutaraldehyde, which toxicity and non-specificity make it inappropriate for skin filler applications, crosslinking of the alcohol groups with a maleic anhydride copolymer was reported by Larrañeta et al. [Citation64]. Alternatively, Huin-Amargier et al. reported the nucleophilic esterification of the HA carboxylate groups with tetraethyleneglycol bis toluenesulfonate [Citation65]. Finally, de Nooy et al. reported an elegant crosslinking process by in situ Ugi multicomponent reaction [Citation66]. Nonetheless, there is still considerable room for innovation, even though innovative crosslinkers are rarely commercially available.
Table 2. Review of different covalent crosslinking strategies to fabricate HA gels. Conjugation techniques as well as physical crosslinking are not reported
The second approach is to modify the structure of the spacer group. Optimizing the length and polarity of the spacer can impact the properties of the gel, although no systematic study of the effect of crosslinker structure on gel properties seems to have been reported. The various efforts using the oligomeric and hydrophilic PEG chain have been mentioned above and a PEGDE-crosslinked HA filler product line has recently been marketed, exhibiting high hygroscopicity compared to BDDE-crosslinked gels [Citation23]. In addition, Khaleghi et al. have reported the use of polydimethylsiloxane-diglycidyl ether, a hydrophobic crosslinker, which should be preliminary dissolved in organic solvent (DMSO) before crosslinking [Citation76].
Each of these crosslinkers raise interesting advantages and challenges from the process point of view, yet in all cases, the principal issue to be considered in medical practice remains the metabolic fate of the crosslinker during resorption. The three key rationales for developing new crosslinkers for native HA are thus: (1) improving the physical properties of the hydrogel filler; (2) improving process issues, including reaction conditions, crosslinker solubility, and purification conditions, the latter being a necessarily time-consuming and costly step of the process; (3) improving the metabolic fate of the crosslinker and crosslinking residues. The perfect crosslinker would add useful properties to the gel, be perfectly water soluble, react under mild conditions, be easy to extract from the gel, and be metabolized though well-known and nontoxic pathways.
4.2. Crosslinking of modified HA
Alternatively, it is possible to modify HA prior to crosslinking in order to reveal new reactive functional groups, with advantages such as high yields, high selectivity of the complementary functional groups, the rapidness of crosslinking, or the use of milder crosslinking conditions to better preserve HA chains ().
For example, oxidative cleavage of the glucuronic acid ring with periodate reveals two aldehyde groups, and Schiff-base formation was successfully used to fabricate HA gel networks using adipic dihydrazide () [Citation77]. Another possibility is to covalently derivatize the HA chains with functionalities that react under mild conditions with various crosslinkers. Amines, phenols, maleimides, alkenes, dienes, and strained alkynes have been covalently bound to HA, and these functionalities have been crosslinked by specific enzymatic, traditional, and click-chemistry reactions (). Moreover, the crosslinking step can be utilized to incorporate covalently attached functional molecules such as peptides in the gel structure, thereby bringing new functionalities to the implant such as cell adhesion through RGD (Arginylglycylaspartic acid) peptide [Citation78].
Physical, non-covalent crosslinks are also widely used in hydrogel fabrication, the main drawback being the long-term stability of the gel compared to covalent crosslinks. Several studies took advantage of strong inclusion complexes formed by cyclodextrin macrocycles and adamantane via guest (adamantane)-host (cyclodextrin) interactions, giving rise to strong supramolecular (non-covalent) bonds [Citation79,Citation80]. Cyclodextrin, a sugar cycle similar to a barrel, has a hydrophobic cavity able to form strong interactions with hydrophobic guests such as adamantane [Citation10]. For instance, Kovacevic et al. synthesized a cyclodextrin-modified HA gel thanks to the inclusion of a di- or trifunctional adamantane-terminated crosslinkers [Citation81].
Although the crosslinking step can occur under milder conditions, the main challenges of these approaches remain the preliminary modifications of HA chains required to permit reaction of these new crosslinkers, which can be difficult to implement industrially without using toxic reagents, solvents, or catalysts that will demand drastic purification steps and meticulous analytical methods to monitor their elimination in finished goods. Crosslinkers are also often non-commercially available and may be difficult to produce industrially. Modified HA and new crosslinkers would also constitute new raw materials for Regulatory Authorities thereby implying safety evaluation. Moreover, little is known about reaction by-products, degradation products or metabolites of such structures, or on the long-term stability and toxicity thereof, thus limiting their immediate use in commercial products.
4.3. Conjugation technique of derivatized HA
Many different studies highlighted the gelation of HA without the need for crosslinkers, using the conjugation technique [Citation10]. The reacting functional groups are similar to the ones used in HA/covalent crosslinker systems, but this time, both functional groups are directly borne on HA chains (). A prerequisite of this technique involves the derivatization of native HA chains with reactive functional groups. The HA chain derivatives may self-react under specific conditions to form a gel, such as methacrylate-derivatized HA chains which photopolymerize using UV light and a photoinitiator [Citation82], or may react thanks to the simultaneous mixing of two modified HA chains bearing the reactive and complementary functional groups. The advantages of such reactions are mainly: 1) the ability to crosslink the HA chains in milder conditions 2) the absence of a crosslinker that must be washed from the gel and 3) the use of a new variety of functionalities on HA potentially bringing new viscoelastic or biological behavior to the final gel network. The same drawbacks than covalent crosslinking are foreseen with respect to the preparation of the derivitized HA.
Interestingly, this gelation process may occur directly in situ [Citation83,Citation84]. For instance, the practitioner would mix the pre-gel mixture before injection or simultaneously inject the solutions using a dual-barrel syringe injection with modified HA solutions forming a gel once implanted. The main advantages are the ease of injection of HA derivatives solutions instead of gels which may lower the extrusion force and the faster purification process of modified HA solutions compared to gel networks, thanks to a higher diffusion of undesirable molecules. However, main drawbacks might be the lack of control over the gelation process and resulting viscoelastic properties, inducing potential safety issues and/or loss of effectiveness for a given clinical application, the toxicity of the unreacted reactive moieties in vivo since the crosslinking may not be fully achieved with no possible purifications, and the future of the potentially released reactive moieties. This technique may also require high amount of HA modifications to improve the reactivity of the compatible functional groups in complex in vivo environments to achieve relevant viscoelastic properties.
These innovative routes for HA crosslinking pave the way to brand new HA filler materials with tunable viscoelasticity, self-healing properties, and enhanced adhesion to surrounding tissues, potentially manufactured using greener and safer raw materials. The duration of in vivo clinical effect could be fine-tuned by modifying enzymatic recognition sites [Citation53] or by the addition of hyaluronidase inhibitors embedded in gels [Citation85]. A special attention is nevertheless the capacity to still allow a prompt and complete filler dissolution, if needed to resolve safety issues or correct esthetically displeasing outcomes.
Extensive preclinical studies are nevertheless required to ensure the long-term stability, biocompatibility and efficacy of such systems, especially regarding the ultimate fate of the reactive sites and the potential metabolites released in vivo after resorption. Our team is currently working on another review of literature dealing with conjugation methods to create HA-based gels.
5. Conclusion
Crosslinkers currently used in commercial HA fillers represent a small part of the whole spectrum of available options for chemically bonding HA chains. This is partly attributable to the challenging regulatory journey required for bringing new fillers on the market, encouraging manufacturers to rely on materials and processes that are well-known to the evaluators. The main reasons for using DVS or bisepoxide crosslinkers, more specifically BDDE, are the associated one-step crosslinking reaction on native HA that does not require additional chemicals and more than one purification step, the vast range of product indications that are already covered by fillers manufactured with such processes, and their proven safety track record. Implementing a new HA hydrogel fabrication strategy is one of the new challenge filler manufacturers are now facing to bring novelty to the market. New viscoelastic properties, milder crosslinking conditions to preserve HA chains, new biomechanical features are among the novel opportunities. However, innovative products require extensive stability, biocompatibility and clinical studies lasting several years to meet the highest standards. Indeed, the existing innovative alternatives to traditional crosslinkers are nowadays mostly confined to laboratory research thus little is known about the long-term stability of resulting hydrogels, or on their toxicity profile. However, we can expect some of these innovations to reach the market in due course.
6. Expert opinion
These expert commentary and 5-year-view sections were written by Teoxane SA’s R&D Department, Teoxane being a leading company in HA-based soft-tissue fillers.
BDDE is nowadays the standard crosslinker of HA for its use in fillers in esthetic medicine. This crosslinker brings significant advantages. First, the biocompatibility of crosslinked HA gels needs to be extensively demonstrated by manufacturers through biocompatibility and safety studies conditioning regulatory approvals. BDDE-crosslinked HA was proven to be safe based on more than 25 years of clinical experience, with a rising number of injections carried out each year worldwide. Besides, BDDE readily reacts with HA to form a gel network by forming inactive and nontoxic ether covalent bonds with HA. As a result, HA chains are ether-linked by an inert diol molecule which is no longer BDDE. During the crosslinking step, unreacted BDDE promptly hydrolyzes into an inactive diol form which is further eliminated by purification steps. BDDE residual traces are either undetectable in the final gel formulation or at least below the reported safety limit. It is important to note that the crosslinker as a raw material must be reactive to effectively react with HA and may present toxicity if tested as a reactive raw material. Nevertheless, this form remains active on a very limited period, the time needed to manufacture the gel, and is subsequently eliminated through purification steps. It is therefore of limited relevance to the medical practitioner and the patient.
In addition, the use of BDDE does not have significant drawbacks. Treatment-related side effects following a HA filler injection are rather linked to the procedure than to the presence of BDDE. Indeed, adverse events are relatively rare regarding the invasiveness of the procedure and the growing number of procedures carried out every year [Citation55]. Crosslinking HA with BDDE guarantees long-lasting effectiveness without jeopardizing filler resorption. Clinical studies evaluating BDDE-crosslinked HA fillers demonstrated their safety and efficacy at improving various facial esthetic features with natural-looking results. In the domain of HA fillers, BDDE features an unrivaled number of clinical studies, historical safety records, and number of products approved on the market.
Through fine-tuning of the HA crosslinking reaction parameters, BDDE, among other process parameters, provides manufacturers with numerous opportunities to obtain and adjust fillers properties, in particular their viscoelasticity, to meet very specific clinical needs and indications. Moreover, BDDE-crosslinked HA gels may be injected without a massive gel swelling due to their rather low propensity to absorb water.
Some efforts have been made to use other crosslinkers. However, in the worst case these attempts have resulted in preclinical, clinical, or commercial failures. In the best case, they could not outperform BDDE and its safety and efficacy records. Nevertheless, this does not preclude the prospection for alternative crosslinking solutions. This could even represent an opportunity for manufacturers to extend the scope of HA hydrogels to innovative applications, new indications, and improved gel properties.
We, Teoxane, as a market reference in the domain of HA-based soft-tissue fillers, believe in the following changes regarding crosslinkers employed in the manufacturing of fillers in the future:
a better similarity with natural unmodified HA by lowering crosslinking rates (or HA degrees of modification) with the goal to achieve clinically relevant viscoelastic properties by adapting manufacturing processes, as initiated with Teosyal RHA® products manufactured with a low degree of HA modification while being resilient, cohesive, long-lasting, and more malleable gels [Citation35].
the use of new effective linkers or conjugation techniques usable in mild crosslinking conditions to preserve HA chains even better. The unreacted residues, by-products, metabolites, as well as the stability will have to be meticulously monitored. As the development timeline is very long, we may expect several patents on this subject coming in the next 5 years.
the use of crosslinkers or conjugated moieties that can bring an ancillary action to the filler, such as interesting features to the gel in terms of mechanical properties, and/or biological accessory activities.
Besides the scientific challenge to bring innovation on the market, substantial efforts must be done both evaluating the safety and the performance of new fillers.
Article highlights
To manufacture HA fillers, crosslinkers should meet a drastic list of chemistry, stability and safety requirements due to the highly regulated domain of implantable medical devices. As a result, only a limited range of candidates are currently used industrially.
1,4-butanediol diglycidyl ether is by far the gold standard crosslinker with unrivaled published scientific and clinical data proving the efficacy and safety of BDDE-crosslinked HA fillers.
New HA hydrogel fabrication routes are emerging, mostly at the laboratory scale, anticipating milder (preservation of HA chains) and more efficient ways to manufacture HA fillers, while potentially bringing innovative features to the gel network such as new viscoelastic properties, and thus new product indications.
These innovative routes of HA hydrogel fabrication require extensive, time-consuming (several years) and costly toxicological, biocompatibility, and stability assessments of final products prior to human use.
While HA filler manufacturers currently provide practitioners with ready-to-use HA gels, new alternatives foresee the use of easy-to-inject pre-gel solutions that form a gel directly in situ.
Declaration of Interest
Teoxane SA is an HA-based filler manufacturer. J Faivre, J Iehl, P Maffert, and F Bourdon are employees at Teoxane SA. P Goekjian’s lab has received a grant from Teoxane SA. PI Amos is an intern in P Goekjian’s lab. PIA gratefully acknowledges an MSc scholarship from the Petroleum Technology Development Fund, Nigeria. PGG wishes to thank the Auvergne-Rhone-Alpes Region (SCUSI 2017 009361 02). The authors have no other relevant affiliations or financial involvement with any organization or entity with a financial interest in or financial conflict with the subject matter or materials discussed in the manuscript apart from those disclosed.
Reviewer disclosures
One peer reviewer is a consultant and speaker for Allergan-Abbvie. Another peer reviewer is a trainer and speaker for Allergan. Peer reviewers on this manuscript have no other relevant financial relationships or otherwise to disclose.
References
- Funt D, Pavicic T. Dermal fillers in aesthetics: an overview of adverse events and treatment approaches. Clin Cosmet Investig Dermatol. 2013;6:295–316.
- Bogdan Allemann I, Baumann L. Hyaluronic acid gel (Juvéderm) preparations in the treatment of facial wrinkles and folds. Clin Interv Aging. 2008;3(4):629–634.
- Kim ZH, Lee Y, Kim SM, et al. A composite dermal filler comprising cross-linked hyaluronic acid and human collagen for tissue reconstruction. J Microbiol Biotechnol. 2015;25(3):399–406.
- Mansouri Y, Goldenberg G. Update on hyaluronic acid fillers for facial rejuvenation. Cutis. 2015;96(2):85–88.
- Tezel A, Fredrickson GH. The science of hyaluronic acid dermal fillers. J cosmet laser ther: off publ Eur Soc Laser Dermatol. 2008;10(1):35–42
- Akkary E, Shumway R, Barnett J. Purified Polydimethylsiloxane (Silicone) as Dermal Filler Resolving the Controversy? Am j cosmet surg. 2019;36(2):71–77
- Friedmann DP, Kurian A, Fitzpatrick RE. Delayed granulomatous reactions to facial cosmetic injections of polymethylmethacrylate microspheres and liquid injectable silicone: a case series. J cosmet laser Ther: Off Publ Eur Soc Laser Dermatol. 2016;18(3):170–173
- Sadick N, Sorhaindo L. The utility of soft tissue fillers in clinical dermatology: treatment of fine wrinkles and skin defects. Expert Rev Med Devices. 2007;4(4):559–565.
- Falcone SJ, Berg RA. Crosslinked hyaluronic acid dermal fillers: a comparison of rheological properties. J Biomed Mater Res A. 2008;87(1):264–271.
- Trombino S, Servidio C, Curcio F, et al. Strategies for Hyaluronic Acid-Based Hydrogel Design in Drug Delivery. Pharmaceutics. 2019;11(8):407.
- Fallacara A, Baldini E, Manfredini S, et al. Hyaluronic acid in the third millennium. Polymers (Basel). 2018;10(7):701.
- Yeom J, Bhang SH, Kim BS, et al. Effect of cross-linking reagents for hyaluronic acid hydrogel dermal fillers on tissue augmentation and regeneration. Bioconjug Chem. 2010;21(2):240–247.
- Tokudome Y, Komi T, Omata A, et al. A new strategy for the passive skin delivery of nanoparticulate, high molecular weight hyaluronic acid prepared by a polyion complex method. Sci Rep. 2018;8(1):2336.
- Juncan AM, Moisă DG, Santini A, et al. Advantages of hyaluronic acid and its combination with other bioactive ingredients in cosmeceuticals. Molecules. 2021;26(15):4429.
- Laurent TC, Fraser JR. Hyaluronan. Faseb J. 1992;6(7):2397–2404.
- Khunmanee S, Jeong Y, Park H. Crosslinking method of hyaluronic-based hydrogel for biomedical applications. J Tissue Eng. 2017;8:1–16.
- Burdick JA, Prestwich GD. Hyaluronic acid hydrogels for biomedical applications. Adv Mater. 2011;23(12):H41–56.
- Laurent UBG, Reed RK. Turnover of hyaluronan in the tissues. Adv Drug Deliv Rev. 1991;7(2):237–256.
- Stern R, Kogan G, Jedrzejas MJ, et al. The many ways to cleave hyaluronan. Biotechnol Adv. 2007;25(6):537–557.
- Soltés L, Mendichi R, Kogan G, et al. Degradative action of reactive oxygen species on hyaluronan. Biomacromolecules. 2006;7(3):659–668.
- Kablik J, Monheit GD, Yu L, et al. Comparative physical properties of hyaluronic acid dermal fillers. Dermatologic Surg. 2009;35(Sup 1):302–312.
- Shmidov Y, Zhu Y, Matson JB, et al. Effect of Crosslinker Topology on Enzymatic Degradation of Hydrogels. Biomacromolecules. 2020;21(8):3279–3286.
- Monticelli D, Martina V, Mocchi R, et al. Chemical Characterization of Hydrogels crosslinked with polyethylene glycol for soft tissue augmentation. Open Access Maced J Med Sci. 2019;7(7):1077–1081.
- Fagien S, Klein AW. A brief overview and history of temporary fillers: evolution, advantages, and limitations. Plast Reconstr Surg. 2007;120(Supplement):8S–16S.
- Kim JE, Sykes JM. Hyaluronic acid fillers: history and overview. Facial plastic surgery: FPS. 2011;27(6):523–528
- Kontis TC, Rivkin A. The history of injectable facial fillers. Facial plastic surgery: FPS. 2009;25(2):67–72
- Kinney BM. Injecting Puragen Plus into the nasolabial folds: preliminary observations of FDA trial. Aesthet Surg J. 2006;26(6):741–748.
- Zhao P, Zhao W, Zhang K, et al. Polymeric injectable fillers for cosmetology: current status, future trends, and regulatory perspectives. J Appl Polym Sci. 2019;n/a(n/a):48515.
- Zerbinati N, Esposito C, Cipolla G, et al. Chemical And Mechanical Characterization Of Hyaluronic Acid Hydrogel Cross-Linked With Polyethylen Glycol And Its Use In Dermatology. Dermatol Ther. 2020;n/a(n/a):e13747.
- Yang B, Guo X, Zang H, et al. Determination of modification degree in BDDE-modified hyaluronic acid hydrogel by SEC/MS. Carbohydr Polym. 2015;131:233–239.
- Lebreton P. US7741476B2. Cross-linking of low and high molecular weight polysaccharides preparation of injectable monophase hydrogels and polysaccharides and hydrogels thus obtained. (June 22, 2010). (Ed.^(Eds).
- Gerup B US5827937A. Polysaccharide gel composition. (Oct 27 1998).
- Wende FJ, Gohil S, Nord LI, et al. 1D NMR methods for determination of degree of cross-linking and BDDE substitution positions in HA hydrogels. Carbohydr Polym. 2017;157:1525–1530.
- Wende FJ, Gohil S, Mojarradi H, et al. Determination of substitution positions in hyaluronic acid hydrogels using NMR and MS based methods. Carbohydr Polym. 2016;136:1348–1357.
- Faivre J, Gallet M, Tremblais E, et al. Advanced Concepts in Rheology for the Evaluation of Hyaluronic Acid-Based Soft Tissue Fillers. Dermatologic Surg. 2021;47(5):e159–e167.
- Edsman K, Nord LI, Ohrlund A, et al. Gel properties of hyaluronic acid dermal fillers. Dermatologic Surg. 2012;38(7):1170–1179.
- Lai JY. Relationship between structure and cytocompatibility of divinyl sulfone cross-linked hyaluronic acid. Carbohydr Polym. 2014;101:203–212.
- Shimojo AAM, Pires AMB, Lichy R, et al. The Performance of Crosslinking with Divinyl Sulfone as Controlled by the Interplay Between the Chemical Modification and Conformation of Hyaluronic Acid. J Braz Chem Soc. 2015;26:506–512.
- Kuo JW, Swann DA, Prestwich GD. Chemical modification of hyaluronic acid by carbodiimides. Bioconjug Chem. 1991;2(4):232–241.
- Sadozai KK, Gooding TB, Bui K, et al. US8124120. Crosslinked Hyaluronic acid compositions for tissue augmentation. (2003 Dec 22). (Ed.^(Eds)
- Alonso JM, Andrade Del Olmo J, Perez Gonzalez R, et al. Injectable Hydrogels: from Laboratory to Industrialization. Polymers. 2021;13(4):650.
- Stern R. Hyaluronan catabolism: a new metabolic pathway. Eur J Cell Biol. 2004;83(7):317–325.
- Sall I, Férard G. Comparison of the sensitivity of 11 crosslinked hyaluronic acid gels to bovine testis hyaluronidase. Polym Degrad Stab. 2007;92(5):915–919.
- Jones D, Tezel A, Borrell M. In vitro resistance to degradation of hyaluronic acid dermal fillers by ovine testicular hyaluronidase. Dermatol Surg. 2010;36(1):804–809.
- De Boulle K, Glogau R, Kono T, et al. A review of the metabolism of 1,4-butanediol diglycidyl ether-crosslinked hyaluronic acid dermal fillers. Dermatologic Surg. 2013;39(12):1758–1766.
- Stropoli SJ, Elrod MJ. Assessing the potential for the reactions of epoxides with amines on secondary organic aerosol particles. J Phys Chem A. 2015;119(40):10181–10189.
- Ahmed EM. Hydrogel: preparation, characterization, and applications: a review. J Adv Res. 2015;6(2):105–121.
- Summary of Safety and Effectiveness Data [Internet]. Medicis Aesthetics Holdings, Inc; 2005. Available from: https://www.accessdata.fda.gov/cdrh_docs/pdf4/P040024B.pdf*OriginaldocumentdealingwiththelimitofBDDEat2ppmwhichisconsideredtobesafeforafiller
- Jeong CH, Kim DH, Yune JH, et al. In vitro toxicity assessment of crosslinking agents used in hyaluronic acid dermal filler. Toxicol in Vitro. 2021;70:105034.
- Fidalgo J, Deglesne PA, Arroyo R, et al. Detection of a new reaction by-product in BDDE cross-linked autoclaved hyaluronic acid hydrogels by LC-MS analysis. Med Devices (Auckl). 2018;11:367–376.
- West JD, Stamm CE, Brown HA, et al. Enhanced Toxicity of the Protein Cross-Linkers divinyl sulfone and diethyl acetylenedicarboxylate in comparison to related monofunctional electrophiles. Chem Res Toxicol. 2011;24(9):1457–1459.
- Gold MH. Soft tissue augmentation in dermatology - 2009 update. J Cutan Aesthet Surg. 2010;3(1):2–10.
- Zhong SP, Campoccia D, Doherty PJ, et al. Biodegradation of hyaluronic acid derivatives by hyaluronidase. Biomaterials. 1994;15(5):359–365.
- Knopf-Marques H, Pravda M, Wolfova L, et al. Hyaluronic acid and its derivatives in coating and delivery systems: applications in tissue engineering, regenerative medicine and immunomodulation. Adv Healthc Mater. 2016;5(22):2841–2855.
- 2020 national Plastic Surgery Statistics [Internet]. American Society of Plastic Surgeons; 2021. Available from: https://wwwplasticsurgeryorg/documents/News/Statistics/2020/plastic-surgery-statistics-report-2020pdf*Overviewofstatisticsaboutthecosmeticsurgical,minimally-invasive,andreconstructiveprocedures
- Wang C, Luan S, Panayi AC, et al. Effectiveness and Safety of Hyaluronic Acid Gel with Lidocaine for the Treatment of Nasolabial Folds: a Systematic Review and Meta-analysis. Aesthetic Plast Surg. 2018;42(4):1104–1110.
- Stojanovič L, Majdič N. Effectiveness and safety of hyaluronic acid fillers used to enhance overall lip fullness: a systematic review of clinical studies. J Cosmet Dermatol. 2019;18(2):436–443.
- Abduljabbar MH, Basendwh MA. Complications of hyaluronic acid fillers and their managements. J Dermatol Dermatologic Surg. 2016;20(2):100–106
- Humphrey S, Jones DH, Carruthers JD, et al. Retrospective review of delayed adverse events secondary to treatment with a smooth, cohesive 20-mg/mL hyaluronic acid filler in 4500 patients. J Am Acad Dermatol. 2020;83(1):86–95.
- Beleznay K, Carruthers JD, Carruthers A, et al. Delayed-onset nodules secondary to a smooth cohesive 20 mg/mL hyaluronic acid filler: cause and management. Dermatologic Surg. 2015;41(8):929–939.
- Smith L, Cockerham K. Hyaluronic acid dermal fillers: can adjunctive lidocaine improve patient satisfaction without decreasing efficacy or duration? Patient Prefer Adherence. 2011;5:133–139.
- Onesti M, Toscani M, Curinga G, et al. Assessment of a new hyaluronic acid filler. double-blind, randomized, comparative study between Puragen and Captique in the treatment of nasolabial folds. In Vivo. 2009;23(3):479–486.
- Schanté CE, Zuber G, Herlin C, et al. Chemical modifications of hyaluronic acid for the synthesis of derivatives for a broad range of biomedical applications. Carbohydr Polym. 2011;85(3):469–489.
- Larrañeta E, Henry M, Irwin NJ, et al. Synthesis and characterization of hyaluronic acid hydrogels crosslinked using a solvent-free process for potential biomedical applications. Carbohydr Polym. 2018;181:1194–1205.
- Huin-Amargier C, Marchal P, Payan E, et al. New physically and chemically crosslinked hyaluronate (HA)-based hydrogels for cartilage repair. J Biomed Mater Res A. 2006;76(2):416–424.
- de Nooy Aej, Capitani D, Masci G, et al. Ionic Polysaccharide Hydrogels via the Passerini and Ugi Multicomponent Condensations: synthesis, Behavior and Solid-State NMR Characterization. Biomacromolecules. 2000;1(2):259–267.
- Tomihata K, Ikada Y. Crosslinking of hyaluronic acid with glutaraldehyde. J Polym Sci A Polym Chem. 1997;35(16):3553–3559.
- Maiz-Fernández S, Pérez-Álvarez L, Ruiz-Rubio L, et al. Synthesis and Characterization of Covalently Crosslinked pH-Responsive Hyaluronic Acid Nanogels: effect of Synthesis Parameters. Polymers. 2019;11(4):742.
- Magnani A, Rappuoli R, Lamponi S, et al. Novel polysaccharide hydrogels: characterization and properties. ?Polym Adv Technol. 2000;11(8‐12):488–495.
- Prestwich GD, Marecak DM, Marecek JF, et al. Controlled chemical modification of hyaluronic acid: synthesis, applications, and biodegradation of hydrazide derivatives. J Control Release. 1998;53(1–3):93–103.
- Ranga A, Lutolf MP, Hilborn J, et al. Hyaluronic Acid Hydrogels Formed in Situ by Transglutaminase-Catalyzed Reaction. Biomacromolecules. 2016;17(5):1553–1560.
- Gramlich WM, Kim IL, Burdick JA. Synthesis and orthogonal photopatterning of hyaluronic acid hydrogels with thiol-norbornene chemistry. Biomaterials. 2013;34(38):9803–9811.
- Hui E, Gimeno KI, Guan G, et al. Spatiotemporal Control of Viscoelasticity in Phototunable Hyaluronic Acid Hydrogels. Biomacromolecules. 2019;20(11):4126–4134.
- Yu F, Cao X, Li Y, et al. Diels–Alder Click-Based Hydrogels for Direct Spatiotemporal Postpatterning via Photoclick Chemistry. ACS Macro Lett. 2015;4(3):289–292.
- Fu S, Dong H, Deng X, et al. Injectable hyaluronic acid/poly(ethylene glycol) hydrogels crosslinked via strain-promoted azide-alkyne cycloaddition click reaction. Carbohydr Polym. 2017;169:332–340.
- Khaleghi M, Ahmadi E, Khodabandeh Shahraki M, et al. Temperature-dependent formulation of a hydrogel based on Hyaluronic acid-polydimethylsiloxane for biomedical applications. Heliyon. 2020;6(3):e03494.
- Su W-Y, Chen K-H, Chen Y-C, et al. An Injectable Oxidated Hyaluronic Acid/Adipic Acid Dihydrazide Hydrogel as a vitreous substitute. J Biomater Sci Polym Ed. 2011;22(13):1777–1797.
- Wang L-S, Lee F, Lim J, et al. Enzymatic conjugation of a bioactive peptide into an injectable hyaluronic acid–tyramine hydrogel system to promote the formation of functional vasculature. Acta Biomater. 2014;10(6):2539–2550.
- Moghaddam S, Yang C, Rekharsky M, et al. New Ultrahigh Affinity Host−Guest Complexes of Cucurbit[7]uril with Bicyclo[2.2.2]octane and Adamantane Guests: thermodynamic Analysis and Evaluation of M2 Affinity Calculations. J Am Chem Soc. 2011;133(10):3570–3581.
- Liu G, Yuan Q, Hollett G, et al. Cyclodextrin-based host–guest supramolecular hydrogel and its application in biomedical fields. Polym Chem. 2018;9(25):3436–3449.
- Kovačević J, Prucková Z, Pospíšil T, et al. A New Hyaluronan Modified with β-Cyclodextrin on Hydroxymethyl groups forms a dynamic supramolecular network. Molecules. 2019;24(21):3849.
- Baier Leach J, Bivens KA, Patrick Jr. CW Jr, et al. Photocrosslinked hyaluronic acid hydrogels: natural, biodegradable tissue engineering scaffolds. Biotechnol Bioeng. 2003;82(5):578–589.
- Heo JY, Noh JH, Park SH, et al. An injectable click-crosslinked hydrogel that prolongs dexamethasone release from dexamethasone-loaded microspheres. Pharmaceutics. 2019;11(9):438.
- Park SH, Seo JY, Park JY, et al. An injectable, click-crosslinked, cytomodulin-modified hyaluronic acid hydrogel for cartilage tissue engineering. Npg Asia Mater. 2019;11(1):30.
- Kim M-H, Park J-H, Nguyen D-T, et al. Hyaluronidase inhibitor-incorporated cross-linked hyaluronic acid hydrogels for subcutaneous injection. Pharmaceutics. 2021;13(2):170.