ABSTRACT
Introduction
Radiofrequency (RF) ablation of the left atrium of the heart is increasingly used to treat atrial fibrillation (AF). Unfortunately, inadvertent thermal injury to the esophagus can occur during this procedure, potentially creating an atrioesophageal fistula (AEF) which is 80% fatal. The ensoETM (Attune Medical, Chicago, IL), is an esophageal cooling device that has been shown to reduce thermal injury to the esophagus during RF ablation.
Areas covered
This review summarizes growing evidence related to active esophageal cooling during RF ablation for the treatment of AF. The review presents data demonstrating improved outcomes related to patient safety and procedural efficiency and suggests directions for future research.
Expert opinion
The use of active esophageal cooling during RF ablation reduces esophageal injury, reduces or eliminates fluoroscopy requirements, reduces procedure duration and post-operative pain, and increases long-term freedom from arrhythmia. These effects in turn increase patient same-day discharge rates, decrease operator cognitive load, and reduce cost. These findings are likely to further accelerate the adoption of active esophageal cooling.
Plain Language Summary
Atrial fibrillation is a condition in which the heart beats irregularly, causing symptoms such as palpitations, dizziness, shortness of breath, and chest pain. Atrial fibrillation increases the risk of stroke, heart failure, dementia, and death. One treatment for atrial fibrillation is a procedure called a catheter ablation. This procedure is minimally invasive and is performed by a specialized cardiologist, called an electrophysiologist. The electrophysiologist, or operator, uses an energy source, such as radiofrequency energy (radio waves), to stop erratic electrical signals from traveling through the heart. One complication of the catheter ablation is an inadvertent injury to the esophagus, the organ that passes food from the mouth to the stomach. If the injury is severe, it may develop into an atrioesophageal fistula, which often results in death. In this review, a new technology is described that helps prevent this type of injury and can provide additional benefits for the patient, operator, and hospital.
1. Introduction
The incidence of atrial fibrillation (AF) is increasing worldwide, largely because AF occurs more frequently with advancing age, and a growing population of patients in high-income countries are aged >65 years [Citation1,Citation2]. As a method of treatment, catheter ablation is increasingly being used to restore patients to sinus rhythm [Citation3]. One major risk from this procedure is thermal injury to the esophagus, which is a consequence of the proximity of the posterior wall of the left atrium to the anterior wall of the esophagus [Citation4–8]. Traditional methods to protect the esophagus from thermal injury include reducing radiofrequency (RF) power when the catheter is near the esophagus, monitoring luminal esophageal temperature (LET), which depends on the operator halting ablation when the temperature rises, and moving, or deviating, the esophagus using a variety of devices. To date, none of these approaches has demonstrated benefit in rigorous randomized controlled trials [Citation9–12].
In contrast, active esophageal cooling has shown benefit in several studies, including two randomized controlled pilot studies [Citation13,Citation14] and one large randomized controlled trial (RCT) [Citation15]. The largest trial demonstrated an 83% reduction of endoscopically detected esophageal lesions. An esophageal cooling device (ensoETM, Attune Medical, Chicago, IL) developed for patient temperature management became available commercially in Europe in 2014 and in the United States in 2015 [Citation16,Citation17]. The device is a multi-lumen medical-grade silicone tube () with channels to allow the flow of water provided by an external heat exchanger. Adjustment of water temperature on the external heat exchanger allows continuous cooling of the esophagus. During RF ablation, a temperature of 4°C is typically used [Citation18]. The device has been used in laser ablation with a similar setpoint temperature, but analysis of efficacy is pending. To date, no known use has occurred with either hot balloon ablation, where atrioesophageal fistula is known to occur [Citation19], or in pulsed field ablation, where thermal injury may be less common, but is likely dependent on specific settings [Citation20,Citation21]. Active cooling using different configurations has been evaluated as a thermal mitigation strategy with pulsed field ablation primarily in oncology applications [Citation22]. On the other hand, the use of the device has been evaluated for warming against cryothermal energy during cryoablation, with results from a pilot study not showing benefit (presumed due to the device temperature maximum of only 42°C that can safely be used) [Citation23].
Figure 1. Diagram of active esophageal cooling device (external heat exchanger not shown). Placement of the device in the esophagus is done analogously to a standard orogastric tube. Once placed, the temperature of the water flowing through the device can be reduced to a temperature of 4°C, effectively maintaining the esophageal wall opposite the posterior wall of the left atrium at a temperature below the lethal isotherm of tissue while radiofrequency ablation is successfully applied to form the intended lesions in the left atrium.
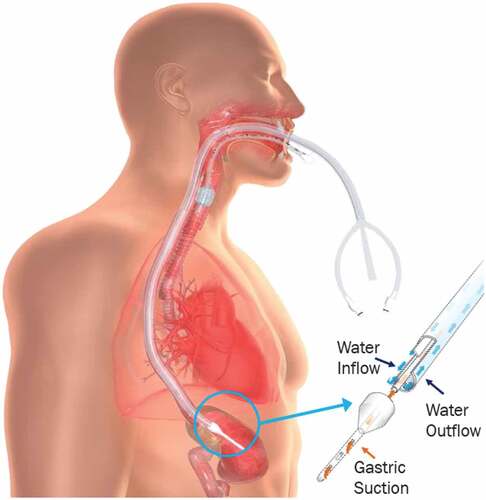
2. Review of reported safety, efficacy, and ancillary benefits
Since the publication of the largest randomized controlled trial of 120 patients in early 2021, adoption of ensoETM has been rapid, resulting in multiple new studies evaluating procedural benefits beyond preventing esophageal injury. These benefits include (1) increased long-term freedom from arrhythmia, (2) reduced procedure duration, (3) reduction or elimination of fluoroscopy requirements, (4) reduced post-operative pain, (5) increased same-day discharge rates, (6) reduced operator cognitive load, and (7) reduced cost. In this review, we present the evidence related to each of these.
2.1. Safety
Esophageal thermal injury resulting in atrioesophageal fistula (AEF) is one of the most feared complications of RF ablation in the left atrium. Estimates of the rates of AEF vary, but because AEF tends to develop weeks after the procedure, and the presenting symptoms mimic the more common presentation of sepsis and/or stroke, the condition is likely under-reported [Citation4,Citation8,Citation24]. Early surveys suggested rates of one out of 1000 or less, but more recent studies, including those from prospectively collected data, suggest a rate ranging from 0.2% to 0.4% [Citation7,Citation25–27].
To date, over 18,000 patients have had ablations with active esophageal cooling using the ensoETM device (with one study evaluating the first 7,000 uses) [Citation28]. With the completion of 18,000 ablations, one would expect an incidence of approximately 36 to 72 AEF cases, assuming no change in the baseline rate of AEF development that occurs with LET monitoring. In fact, no AEF has yet been reported with active esophageal cooling, and only a single case of the less severe pericardial-esophageal fistula is known to have developed. Pericardial-esophageal fistula belongs to a rare subset of fistula formation [Citation29], and analysis of this event identified a number of potentially contributing factors, including higher energy deposition on the esophagus and a stacking of lesions, which is known to pose an increased risk of thermal injury [Citation30].
This difference between expected and reported rates of AEF with the use of active esophageal cooling represents an order-of-magnitude increase in safety over LET monitoring. This effect is believed to stem from two primary factors. The first is the direct thermal impact of cooling, which has been shown to reduce, or eliminate, the potential for esophageal tissue to reach lethal isotherm temperatures [Citation31–33]. Prevention of lethal isotherm temperatures in the esophageal wall is believed to preclude development of the degree of thermal injury that may lead to an AEF weeks after the initial ablation. The second factor impacting safety is the downstream anti-inflammatory effect of cooling. Thermal injury is known to incite a cascade of inflammatory mediators, which result in injury progression, burn conversion, and the development of cellular changes that allow for fistula formation [Citation34–36]. Cooling has mitigating effects on the activity of most of these inflammatory mediators, and abundant clinical data demonstrate marked benefits from cooling burns in preventing worsening injury, reducing grafting requirements, and reducing healing time [Citation37–39]. Combined, these two factors may be synergistic in their effects, providing the degree of safety (a roughly 10-fold reduction in AEF rate) seen to date with active esophageal cooling [Citation28].
2.2. Long-term efficacy
Initial concerns about active esophageal cooling during RF ablation included the potential to cool beyond the esophagus and into the atrial wall, which could reduce the efficacy of the procedure, inhibiting the transmurality of the intentional lesions created to isolate the pulmonary veins and eliminate AF [Citation40]. A number of studies have investigated this outcome in concordance with the availability of more long-term follow-up data. The long-term follow-up data from the IMPACT randomized controlled trial show a 3% increased freedom from atrial arrhythmia in patients treated with esophageal cooling [Citation41]. Although not statistically significant, the absence of a significant worsening of freedom from arrhythmia is notable. Moreover, retrospective data to date support not just an absence of any degradation of long-term efficacy but an increase in freedom from arrhythmia at 1 year. Studies from a wide range of operators and clinical settings have shown improved freedom from arrhythmia at 1 year with active esophageal cooling, with these improvements ranging from 11% to over 24% when compared to LET monitoring [Citation42–45].
The mechanisms underpinning these findings may arise from two factors. First, mathematical models of active esophageal cooling have shown that although the degree of cooling in the esophageal wall is significant, because of the manner in which heat is transferred across tissue boundaries with differing heat transfer coefficients, the influence on the atrial wall is minimal [Citation32,Citation46,Citation47]. This is well illustrated in a recent computational modeling study showing the effects of high-power short-duration ablation on both the atrial and esophageal walls with and without cooling in place (). As shown in , the transmurality of the atrial wall lesions remains at 100% with or without cooling, which is a result of the lower heat transfer coefficients of the different tissues across which heat must transfer, and the limited perfusion of tissues such as the fibrous pericardium and the pericardial fat [Citation47].
Figure 2. Lesion shapes (computed with the Arrhenius model after 90s of equilibration) when using 50 W for 10s or 90 W for 4s, with (protection) and without (control) proactive esophageal cooling. Left sided images for each power setting show the case immediately after the RF pulse, while right sided images for each power setting show the case after 90s to account for lesion growth due to thermal latency. (From Mercado Montoya et al., under the terms of the Creative Commons CC BY license, which permits unrestricted use, distribution, reproduction in any medium, provided the original work is properly cited) [Citation47].
![Figure 2. Lesion shapes (computed with the Arrhenius model after 90s of equilibration) when using 50 W for 10s or 90 W for 4s, with (protection) and without (control) proactive esophageal cooling. Left sided images for each power setting show the case immediately after the RF pulse, while right sided images for each power setting show the case after 90s to account for lesion growth due to thermal latency. (From Mercado Montoya et al., under the terms of the Creative Commons CC BY license, which permits unrestricted use, distribution, reproduction in any medium, provided the original work is properly cited) [Citation47].](/cms/asset/5893efb7-67b4-47e9-b345-9f6220a2674b/ierd_a_2150930_f0002_oc.jpg)
Second, clinical data have shown that lesions placed sequentially without delay (as opposed to lesions placed after a delay due to temperature alarms forcing cessation of RF power) have a higher likelihood of achieving transmurality [Citation48]. Moreover, ensuring continuity of lesion placement without pauses or relocations of the RF catheter during ablation improves pulmonary vein isolation success and long-term freedom from arrhythmia [Citation49]. This aspect of lesion placement has been quantified by a measure referred to as the Continuity Index (CI; see ), which refers to the number of positions in the left atrium that the ablation catheter tip is moved over after prematurely stopping RF power application at a site due to overheating and moving elsewhere in the atrium to perform subsequent ablations in non-adjacent positions. In the EFFICAS-II study, ablations performed with a low CI (CI < 6) had a 98% chance of remaining isolated, while ablations performed with a high CI (CI ≥ 6) had only a 62% chance of remaining isolated [Citation49].
Figure 3. Example of a Continuity Index calculation for two examples of ablation patterns. On the left sided image, ablation proceeds uninterrupted by thermal overheating and subsequent relocations of the ablation catheter. On the right sided image, lesion placement is interrupted at Ablation point #1 due to thermal heating triggering a temperature sensor elevation, prompting the operator to move to Ablation site #2 before returning to continue the lesion set at Ablation site #3 adjacent to the first Ablation site #1. The resulting Continuity Index is determined by the number of non-adjacent segments of the left atrium that are passed over, or crossed, which in this example is equal to 3. Reprinted with permission [Citation49].
![Figure 3. Example of a Continuity Index calculation for two examples of ablation patterns. On the left sided image, ablation proceeds uninterrupted by thermal overheating and subsequent relocations of the ablation catheter. On the right sided image, lesion placement is interrupted at Ablation point #1 due to thermal heating triggering a temperature sensor elevation, prompting the operator to move to Ablation site #2 before returning to continue the lesion set at Ablation site #3 adjacent to the first Ablation site #1. The resulting Continuity Index is determined by the number of non-adjacent segments of the left atrium that are passed over, or crossed, which in this example is equal to 3. Reprinted with permission [Citation49].](/cms/asset/0d0557d2-2eee-4f2b-b508-157436675441/ierd_a_2150930_f0003_oc.jpg)
Active esophageal cooling allows uninterrupted lesion placement without the need to pause for temperature alarms, which may result in a lower Continuity Index (as low as zero in most cases). This may provide a mechanistic rationale for improved freedom from arrhythmia at 1 year with active esophageal cooling.
2.3. Procedure duration
Because the use of active esophageal cooling avoids the need to stop for temperature alarms from local tissue overheating in the esophagus, early operators noted substantial procedure time reductions in left atrial ablation cases using active esophageal cooling when compared to LET monitoring. Several formal analyses into this phenomenon have been completed recently, with findings noting reductions in procedure time of up to 29% [Citation50–55]. The most impressive results came from a two-hospital system that analyzed a total of 373 patients. LET monitoring using a multi-sensor probe was performed in 198 patients, and active esophageal cooling using a dedicated cooling device was performed in 175 patients (patient characteristics did not significantly differ between groups, with a mean age of 67 years, and gender 37.4% female). The mean procedure time was 146 ± 51 min in the LET-monitored patients and 110 ± 39 min in the actively cooled patients, representing a reduction of 36 min, or 24.7% of the total procedure time (p < .001). The median procedure time was 141 [IQR 104 to 174] min in the LET-monitored patients and 100 [IQR 84 to 122] min in the actively cooled patients, for a reduction of 41 min, or 29.1% of the total procedure time (p < .001) [Citation50].
An additional reason for a reduction in procedure time beyond the elimination of the need to pause RF ablation due to high-temperature alarms is avoiding the need to reposition a temperature sensor opposite the RF catheter tip to ensure adequate identification of temperature elevations. Repositioning of a multi-sensor temperature probe is less frequently required than with a single-sensor probe; however, most operators deploy single-sensor probes when using LET monitoring [Citation56].
Reductions in procedure duration offer several benefits beyond hospital cost savings. Increased procedure duration increases the risk of complications [Citation57]. The likelihood of developing a complication (such as surgical or catheter insertion site infection, venous thromboembolism, bleeding, pneumonia, urinary tract infection, renal failure, and hematoma formation) increases with increasing operative time increments (i.e. 1% for every 1-min, 4% for every 10-min, 14% for every 30-min, and 21% for every 60-min increase in operative time) [Citation57,Citation58]. In addition, dwell time in the left atrium is the most significant procedural risk factor for post-operative cognitive dysfunction stemming from ablation procedures [Citation59].
2.4. Fluoroscopy requirements
The ensoETM used for active esophageal cooling is visible on intracardiac echocardiography (ICE), and as such, fluoroscopy is not required to confirm proper placement. Placement and use of the ensoETM has been described [Citation18] and accompanied by a video available online. The device is first connected to an external heat exchanger, which is then turned on to initiate water flow and confirm the absence of leaks. A generous amount of lubrication is then applied to the distal end of the device, after which it is placed in a fashion similar to a standard orogastric tube. Once placement is confirmed with either fluoroscopy or ICE, no additional movement or repositioning is required, and the need for fluoroscopy during the procedure is eliminated [Citation53]. Studies investigating and quantifying this effect have found reductions of 35% in fluoroscopy use when compared to LET monitoring, even in settings where low-fluoroscopy techniques are utilized [Citation60].
More recently, the use of active cooling without fluoroscopy or ICE has been shown to be safe [Citation61]. Data were reviewed for a total of 417 patients undergoing ablation without the use of fluoroscopy or ICE (the zero-fluoroscopy approach was facilitated by the use of transesophageal echocardiography to perform the transseptal puncture). Visualization of the active esophageal cooling device on the 3D mapping system was obtained with a guidewire placed through the central lumen of the cooling device, which was then pinned via a pin block to the 3D mapping system (EnSite Precision™ Cardiac Mapping System, Abbott, St. Paul, MN). Among the 417 patients evaluated, 156 received LET monitoring, and 261 underwent active esophageal cooling. A total of 5 complications were recorded in the active cooling group (three pericardial effusions, one pseudoaneurysm, and one air embolism), for an overall complication rate of 1.9%, in line with typical reported complication rates [Citation62–64].
2.5. Post-operative pain
Cooling of tissues exerts an anti-inflammatory effect, which impacts a range of outcomes. For example, cooling is used to reduce neurological insult from ischemia, prevent thermal injury progression, and reduce post-operative ophthalmologic pain [Citation37,Citation65,Citation66]. The IMPACT trial noted trends toward reduced gastroparesis with active esophageal cooling [Citation15], and extremes of temperature in the esophagus have been shown to correlate with higher rates of gastroparesis (presumed due to vagal plexus or periesophageal plexus injury) [Citation67,Citation68]. Early reports from operators using active esophageal cooling were notable for finding a reduction in patient complaints of chest pain. A survey of operators found an average reduction in patient notifications for chest pain after RF ablation of 58% after adopting active esophageal cooling [Citation69]. A study of 164 patients from a large academic medical center found that chest pain was reported by 11 patients (18%) in the LET monitored group and 7 patients (7%) in the actively cooled group, representing a 60% relative reduction and an 11% absolute reduction in chest pain complaints (p = 0.03) [Citation70].
2.6. Same-day discharge
Same-day discharge is desirable for both patients and hospital systems but requires patients to have undergone an uncomplicated procedure, without complaints such as chest pain that can require overnight observation before safe discharge [Citation71]. Evidence suggests the reason behind the increased same-day discharge is the previously discussed reduction in post-procedural pain complaints (chest pain, gastroparesis, pericarditis, etc.) that historically led to overnight observation. The reductions in post-operative chest pain reported by operators using active esophageal cooling were associated with increases in same-day discharge rate. A study of one academic hospital comparing discharge rates before and after the implementation of active esophageal cooling analyzed a total of 164 patients who underwent RF ablation between January 2020 and January 2022; 63 (38%) patients were treated with LET monitoring and 101 (62%) were treated with active esophageal cooling [Citation70]. Of the patients treated with esophageal cooling, 39 (38.6%) were discharged within 12 hours of admission compared to 13 (20.6%) of the patients treated with LET monitoring (p = 0.016).
2.7. Cognitive load
Increased cognitive load (information overload) on the operator during medical procedures has many adverse consequences [Citation72,Citation73]. The overall cognitive load and intrinsic load are negatively associated with the level of performance, and distractions contribute to extraneous load [Citation73]. Increased cognitive load results in increased response times [Citation74]. Implementing active esophageal cooling removes the alarm component of LET monitoring. The elimination of these alarms during RF ablation has been reported by operators to contribute substantially to reductions in cognitive load during ablation procedures. Further work investigating the implications of these findings is ongoing.
2.8. Cost savings
Procedural time directly impacts costs in the hospital system. Moreover, targeting same-day discharge avoids the additional costs incurred by overnight hospital stays. When factoring in both the shorter procedure time and an improved same-day discharge rate associated with active esophageal cooling, a quantifiable dollar savings can be calculated. The cost of time in the electrophysiology lab has been reported to be $29 per minute [Citation75]. Translating a typical time savings seen with active esophageal cooling into financial costs results in a savings of $1073 per case. The financial impact associated with post-ablation same-day discharge has been estimated at $7116, factoring in the reimbursement available from the additional bed made available by the same-day discharge [Citation76]. After including the increased cost of the active esophageal cooling device over the standard single-sensor LET probe, the net savings to the hospital are $1,554 per patient treated [Citation77].
One factor related to cost is the use of general anesthesia versus conscious sedation. In the U.S., most left atrial ablations are performed with general anesthesia, and as such, no formal comparison has been made between procedures performed under general anesthesia and those performed under conscious sedation. A higher proportion of ablations in Europe are performed under conscious sedation, and in some of these, the esophageal cooling device has been used successfully. The sedation required for use is similar to that used for common procedures such as transesophageal echocardiography (TEE) or esophagoduodenoscopy (EGD), but the total case volume to date limits detailed comparisons. Recent data suggest that general anesthesia adds a small cost (approximately 7% more than conscious sedation) to an ablation procedure [Citation78]. Additionally, no comparisons have been made with ablation procedures in which no LET monitoring is used. The 2017 Expert Consensus offers only a Class IIa recommendation, with a Level of Evidence of C-EO (expert opinion), stating that ‘It is reasonable to use an esophageal temperature probe during AF ablation procedures to monitor esophageal temperature and help guide energy delivery.’ [Citation56]. The update to the Expert Consensus document is currently being written, and as such, these recommendations may change.
3. Conclusion
Since first being described in 2005 as a possible strategy for esophageal protection during cardiac ablation, active esophageal cooling has received increasing interest from the electrophysiology community. The FDA clearance in 2015 of a commercially available esophageal cooling device has prompted a rapid growth in adoption of this technique, which in turn has resulted in a substantial amount of new data describing the safety and efficacy of active esophageal cooling. This review highlights the latest data showing reductions in esophageal injury as well as increased long-term freedom from arrhythmia, reduced procedure duration, a reduction or elimination of fluoroscopy requirements, and reduced post-operative pain. These effects in turn enhance same-day discharge rates, reduce cognitive load, and increase cost savings. These favorable facets are likely to further accelerate the adoption of active esophageal cooling.
4. Expert opinion
Active esophageal cooling has emerged from a concept first published almost 2 decades ago to an increasingly utilized technique to reduce esophageal injury during radiofrequency ablation. The introduction in 2015 of a commercially available esophageal cooling device, combined with growing data supporting the safety and other ancillary benefits of this approach, has resulted in further widespread adoption of this technology. The evidence suggests that esophageal cooling results in more effective ablation via improvement in adjacent lesion placement, reduces procedure time resulting in improved patient safety and hospital savings, and reduces post-ablation pain. These factors in turn may result in decreased antiarrhythmic drug utilization and decreased stroke incidence in the treated population, which continues to grow as the population continues to age. At present, one remaining barrier to wider adoption in clinical practice may be the lack of a specific indication statement for esophageal protection with active cooling, limiting the ability to market this technology. To that end, a large multi-center randomized controlled trial (NCT04577859) is underway that will provide data required for the expansion of the current FDA labeling to include reduction of esophageal injury.
Key areas for improvement in the treatment of atrial fibrillation include identifying patients earlier to prevent progression, reducing the need for repeat ablations due to recurrence of arrhythmia, appropriately managing the balance between destroying arrhythmogenic myocytes and inadvertently harming adjacent structures such as the esophagus, and monitoring patients after treatment to ensure efficacy of ablation procedures. Active esophageal cooling may address some of these areas directly by the reduction in recurrence of arrhythmia and the reduction in injury to the esophagus.
Newer monitoring devices have come to market, with one having just received FDA clearance for continuous monitoring of atrial fibrillation. The use of personal monitors such as this may reduce reliance on office and hospital visits (where traditional ECGs and Holter monitors are typically used). The consequence of this may be to allow earlier detection of patients with atrial fibrillation, or more sensitive identification of arrhythmias, while also better quantifying atrial fibrillation burden, rather than relying on a binary yes/no determination of arrhythmia at static time points.
Further research holds the potential to restore normal sinus rhythm more effectively and with greater safety than currently possible. Pulsed field energy to induce irreversible electroporation in cardiac tissue was first used in the 1980s but was superseded by radiofrequency ablation due to complications from early iterations of the technology (primarily barotrauma and microbubble formation). Newer approaches to utilizing pulsed field energy have recently been developed, with the anticipation that tissue selectivity might eliminate collateral damage during ablation of cardiac tissue. At present, there has been some progress in this area, with the caveat that demonstration of both safety and long-term efficacy has not been completed. A recent large survey of the first 1,758 cases treated with one of the first successfully commercialized systems for pulsed field ablation found significantly more complications than expected, prompting the authors to note that the high frequency of complications underscores the need for improvement.
Novel energy sources may allow for noninvasive ablation in the future. Although most laboratories are currently focusing on investigating its utility in treating ventricular arrhythmias, stereotactic body radiotherapy (SBRT) has been reported to be successful in the first five patients treated for AF [Citation79]. This noninvasive treatment shows promise but concerns for long-term safety remain for treating a non-lethal arrhythmia such as AF.
One new approach being investigated by a few laboratories and at least one start-up is via gene therapy. A grant has been awarded to one company that has (i) identified major molecular mechanisms contributing to atrial fibrillation, (ii) identified trans-genes to selectively target these mechanisms, and (ii) developed a novel method of facilitating gene delivery via electroporation. If a complete elimination of atrial fibrillation is achieved in this manner, a clear endpoint in the quest to cure atrial fibrillation will have been attained.
Over the next 5 years, evolution in the field of cardiac ablation is likely to include further enhancements and improvements to the mapping technology, allowing a more refined determination of specific areas of the heart to treat (using either radiofrequency, cryothermal, or pulsed field energy). Improvements in safety and effectiveness will allow the use of ablation as a treatment modality even for asymptomatic atrial fibrillation patients and lessen the utilization of rate- and rhythm-controlling drugs while also reducing the need for anti-embolic therapies.
Article highlights
The ensoETM is an esophageal temperature management device that became available in Europe in 2014 and in the United States in 2015.
Recent data have shown the device can decrease esophageal thermal injury during cardiac ablation procedures in the left atrium for the treatment of atrial fibrillation.
Adoption of this technology by electrophysiologists has consequently been rapid, resulting in a proliferation of data demonstrating beneficial effects in outcomes beyond safety.
Studies show that this technology can reduce or eliminate fluoroscopy requirements, reduce procedure duration, decrease post-operative pain, and increase long-term freedom from arrhythmia.
These effects in turn can increase patient same-day discharge rates, decrease operator cognitive load, and reduce cost.
Declaration of interest
Julie Cooper has received funding for data collection from Attune Medical. Erik Kulstad declares equity and employment in Attune Medical. Christopher Joseph declares an internship with Attune Medical. Jason Zagrodzky declares consulting for Biosense Webster and Attune Medical. Christopher Woods declares consulting for Abbott, Farapulse; equity in inHEART Medical; and research funding from Biosense Webster. Mark Metzl declares consulting for Abbott, Biosense Webster, Attune Medical, Medtronic, Sanofi Aventis, and Philips. The authors have no other relevant affiliations or financial involvement with any organization or entity with a financial interest in or financial conflict with the subject matter or materials discussed in the manuscript apart from those disclosed.
Reviewers disclosure
Peer reviewers on this manuscript have no relevant financial relationships or otherwise to disclose.
Supplemental Material
Download MP4 Video (141.4 MB)Supplemental Material
Download MS Word (24.4 KB)Supplementary material
Supplemental data for this article can be accessed online at https://doi.org/10.1080/17434440.2022.2150930
Additional information
Funding
References
- Lippi G, Sanchis-Gomar F, Cervellin G. Global epidemiology of atrial fibrillation: an increasing epidemic and public health challenge. Int J Stroke. 2021;16(2):217–221. PMID: 31955707.
- Rahman F, Kwan GF, Benjamin EJ. Global epidemiology of atrial fibrillation. Nat Rev Cardiol. 2014;11(11):639–654. PMID: 25113750.
- McCarthy PM, Cox JL, Kislitsina ON, et al. Surgery and catheter ablation for atrial fibrillation: history, current practice, and future directions. J Clin Med. 2021;11(1):210. PMID: 35011953.
- Della Rocca DG, Magnocavallo M, Natale VN, et al. Clinical presentation, diagnosis, and treatment of atrioesophageal fistula resulting from atrial fibrillation ablation. J Cardiovasc Electrophysiol. 2021;32(9):2441–2450. PMID: 34260115.
- Nair KK, Shurrab M, Skanes A, et al. The prevalence and risk factors for atrioesophageal fistula after percutaneous radiofrequency catheter ablation for atrial fibrillation: the Canadian experience. J Interv Card Electrophysiol. 2014;39(2):139–144. PMID: 24317916.
- Muller P, Dietrich JW, Halbfass P, et al. Higher incidence of esophageal lesions after ablation of atrial fibrillation related to the use of esophageal temperature probes. Heart Rhythm. 2015;12(7):1464–1469. PMID: 25847474.
- De Vasconcelos JT M, Filho S, Atié J, et al. Atrial-oesophageal fistula following percutaneous radiofrequency catheter ablation of atrial fibrillation: the risk still persists. Europace. 2017;19(2):250–258. PMID: 28175286.
- Shen KR. Commentary: the rising storm of atrioesophageal fistulae after catheter ablation for atrial fibrillation. JTCVS Tech 2021, 6:169 PMID: 34318186
- Leung LWM, Akhtar Z, Sheppard MN, et al. Preventing esophageal complications from atrial fibrillation ablation: a review. Heart Rhythm O2 2021, 2(6Part A):651–664 PMID: 34988511.
- Schoene K, Arya A, Grashoff F, et al. Oesophageal Probe Evaluation in Radiofrequency Ablation of Atrial Fibrillation (OPERA): results from a prospective randomized trial. Europace. 2020;22(10):1487–1494. PMID: 32820324.
- Chen S, Schmidt B, Seeger A, et al. Catheter ablation of atrial fibrillation using ablation index-guided high power (50 W) for pulmonary vein isolation with or without esophageal temperature probe (the AI-HP ESO II). Heart Rhythm. 2020;17(11):1833–1840. PMID: 32470628.
- Meininghaus DG, Blembel K, Waniek C, et al. Temperature monitoring and temperature-driven irrigated radiofrequency energy titration do not prevent thermally-induced esophageal lesions in pulmonary vein isolation a randomized study controlled by esophagoscopy before and after catheter ablation. Heart Rhythm. 2021; PMID: 33561587. doi:10.1016/j.hrthm.2021.02.003
- Tschabrunn CM, Attalla S, Salas J, et al. Active esophageal cooling for the prevention of thermal injury during atrial fibrillation ablation: a randomized controlled pilot study. J Interv Card Electrophysiol. 2022;63(1):197–205. PMID: 33620619.
- Clark B, Alvi N, Hanks J, et al. An esophageal cooling device during radiofrequency ablation for atrial fibrillation – a comparison between reactive and proactive esophageal cooling. J Innov Card Rhythm Manag 2022, November, 2022 In Press PMID
- Leung LWM, Bajpai A, Zuberi Z, et al. Randomized comparison of oesophageal protection with a temperature control device: results of the IMPACT study. Europace. 2021;23(2):205–215. PMID: 33205201.
- Naiman M, Shanley P, Garrett F, et al. Evaluation of advanced cooling therapy’s esophageal cooling device for core temperature control. Expert Rev Med Devices. 2016;13(5):423–433. PMID: 27043177.
- Goury A, Poirson F, Chaput U, et al. Targeted temperature management using the “esophageal cooling device” after cardiac arrest (The COOL Study): a feasibility and safety study. Resuscitation. 2017;121:54–61. PMID: 28951293.
- Zagrodzky J, Gallagher MM, Leung LWM, et al. Cooling or warming the esophagus to reduce esophageal injury during left atrial ablation in the treatment of atrial fibrillation. J Vis Exp. 2020(157): PMID: 32225140 DOI:10.3791/60733.
- Yamasaki H, Nakahara S, Sohara H, et al. HotBalloon pulmonary vein isolation registry study – real-world efficacy and safety of hotballoon ablation. Circ J. 2022. PMID: 36130909. DOI:10.1253/circj.CJ-21-0994.
- Faroja M, Ahmed M, Appelbaum L, et al. Irreversible electroporation ablation: is all the damage nonthermal? Radiology. 2013;266(2):462–470. PMID: 23169795.
- van Gemert MJ, Wagstaff PG, de Bruin DM, et al. Irreversible electroporation: just another form of thermal therapy? Prostate. 2015;75(3):332–335. PMID: 25327875.
- Aycock KN, Campelo SN, Davalos RV. A comparative modeling study of thermal mitigation strategies in irreversible electroporation treatments. J Heat Transfer. 2022;144(3):031206. PMID: 35833151.
- Sink J, Nimmagadda K, Zhao M, et al. Esophageal temperature management during cryoballoon ablation for atrial fibrillation. J Cardiovasc Electrophysiol. 2022;(): doi:10.1111/jce.15724. Online ahead of print. PMID: 36317453.
- Challa B, Yao K, Allenby P, et al. Autopsy techniques in patients with history of radiofrequency ablation for atrial fibrillation. Arch Pathol Lab Med. 2021; PMID: 34619753. doi:10.5858/arpa.2021-0161-OA
- Zima LA, Fornoff LE, Surdell DL. Atrioesophageal fistula: considerations for the neurological clinician. Clin Neurol Neurosurg. 2018;170:58–60. PMID: 29730269.
- Schuring CA, Mountjoy LJ, Priaulx AB, et al. Atrio-esophageal fistula: a case series and literature review. Am J Case Rep. 2017;18:847–854. PMID: 28761039.
- Dagres N, Hindricks G, Kottkamp H, et al. Complications of atrial fibrillation ablation in a high-volume center in 1,000 procedures: still cause for concern? J Cardiovasc Electrophysiol. 2009;20(9):1014–1019. PMID: 19490383.
- Leung LWM, Akhtar Z, Bajpai A, et al. A registry review update of 7120 catheter ablations for atrial fibrillation using a dedicated esophageal temperature control device for protection - CA-528-03. Heart Rhythm. 2022;19(5):S8. DOI: 10.1016/j.hrthm.2022.03.101.
- Back Sternick E, Soares Correa F, Ferber Drumond L, et al. Esophago-pericardial fistula after catheter ablation of atrial fibrillation: a review. J Cardiovasc Electrophysiol. 2020;31(10):2600–2606. PMID: 32829527.
- Sánchez-Muñoz EJ, Berjano E, González-Suárez A. Computer simulations of consecutive radiofrequency pulses applied at the same point during cardiac catheter ablation: implications for lesion size and risk of overheating. Comput Methods Programs Biomed. 2022;220:106817. PMID: 35468542.
- Kulstad E, Mercado-Montoya M, Gomez-Bustamante T, et al. Influence of tissue thickness on thermal latency during high-power short-duration radiofrequency ablation with proactive esophageal cooling. EP Europace. 2022;24(Supplement_1):. DOI:10.1093/europace/euac053.085.
- Mercado M, Leung L, Gallagher M, et al. Modeling esophageal protection from radiofrequency ablation via a cooling device: an analysis of the effects of ablation power and heart wall dimensions. Biomed Eng Online. 2020;19(1):77. PMID: 33046057.
- Montoya MM, Berjano E, Mickelsen SR, et al. Abstract 10771: avoidance of lethal isotherm formation in esophageal tissue with an active cooling device under high-power, short-duration ablation. Circulation. 2021;144(Suppl_1):A10771–A10771. DOI: 10.1161/circ.144.suppl_1.10771.
- Wright EH, Harris AL, Furniss D. Cooling of burns: mechanisms and models. Burns. 2015;41(5):882–889. PMID: 25820085.
- Scharl M, Rogler G. Pathophysiology of fistula formation in Crohn’s disease. World J Gastrointest Pathophysiol. 2014;5(3):205–212. PMID: 25133023.
- Kalantarian S, Clark A, Tamirisa K, et al.: Mechanisms of action behind proactive esophageal cooling during left atrial ablation. AF Symposium 2022, New York, NY; 2022. doi: 10.1111/jce.15392
- Griffin BR, Frear CC, Babl F, et al. Cool running water first aid decreases skin grafting requirements in pediatric burns: a cohort study of two thousand four hundred ninety-five children. Ann Emerg Med. 2020;75(1):75–85. PMID: 31474480.
- Wright EH, Tyler M, Vojnovic B, et al. Human model of burn injury that quantifies the benefit of cooling as a first aid measure. Br J Surg. 2019;106(11):1472–1479. PMID: 31441049.
- Harish V, Tiwari N, Fisher OM, et al. First aid improves clinical outcomes in burn injuries: evidence from a cohort study of 4918 patients. Burns. 2019;45(2):433–439. PMID: 30337155.
- Berjano EJ, Hornero F. A cooled intraesophageal balloon to prevent thermal injury during endocardial surgical radiofrequency ablation of the left atrium: a finite element study. Phys Med Biol. 2005;50(20):N269–279. PMID: 16204868.
- Leung LWM, Akhtar Z, Elbatran A, et al. Effect of esophageal cooling on ablation lesion formation in the left atrium: insights from ablation Index data in the IMPACT trial and clinical outcomes. J Cardiovasc Electrophysiol. 2022 . Online ahead of print. PMID: 36284450 Online ahead of print. PMID: 36284450.
- Joseph C, Francisco G, Ruppert A, et al.: Active esophageal cooling is associated with improved freedom from arrhythmias at one-year: a large hospital registry review. AF Symposium 2022, New York, NY; 2022. doi: 10.1111/jce.15392.
- Joseph C, Francisco G, Ruppert A, et al. Arrhythmia recurrence reduction with an active esophageal cooling device during radiofrequency ablation. EP Europace. 2022;24(Supplement_1). DOI:10.1093/europace/euac053.096.
- Joseph C, Zagrodzky W, Sherman J, et al. One-year outcomes after active cooling during left atrial radiofrequency ablation. J Am Coll Cardiol. 2022;79(9, Supplement):114. doi:10.1016/S0735-1097(22)01105-6.
- Zagrodzky J, Zagrodzky W, Joseph C, et al.: Proactive esophageal cooling during radiofrequency ablation is associated with improved one-year freedom from atrial fibrillation. AF Symposium 2022, New York, NY; 2022. doi: 10.1111/jce.15392.
- Montoya MM, Mickelsen S, Clark B, et al. Protecting the esophagus from thermal injury during radiofrequency ablation with an esophageal cooling device. J Atr Fibrillation. 2019;11(5):2110. PMID: 31139296.
- Mercado Montoya M, Gomez Bustamante T, Berjano E, et al. Proactive esophageal cooling protects against thermal insults during high-power short-duration radiofrequency cardiac ablation. Int J Hyperthermia J Eur Soc Hypertens Oncol 2022, 39(1):1202–1212 PMID: 36104029.
- Jankelson L, Dai M, Aizer A, et al. Lesion sequence and catheter spatial stability affect lesion quality markers in atrial fibrillation ablation. JACC Clin Electrophysiol. 2021;7(3):367–377. PMID: 33516716.
- Kautzner J, Neuzil P, Lambert H, et al.EFFICAS II: optimization of catheter contact force improves outcome of pulmonary vein isolation for paroxysmal atrial fibrillation. Europace. 2015;17(8):1229–1235. PMID: 26041872.
- Joseph C, Sherman J, Ro A, et al. Procedural time reduction associated with active esophageal cooling during pulmonary vein isolation. J Interv Card Electrophysiol. 2022; Online ahead of print. PMID: 35416632.
- Joseph C, Francisco G, Ruppert A, et al. Effect of a proactive esophageal cooling device on procedure length – a multicenter comparison of persistent and paroxysmal atrial fibrillation. EP Europace. 2022;24(Supplement_1). . DOI:10.1093/europace/euac053.086.
- Joseph C, Henry C, Kawasaki R. The impact of active esophageal cooling on pulmonary vein isolation procedure duration. AF Symposium, New York, NY; 2022. DOI:10.1111/jce.15392.
- Joseph C, Sherman J, Ro A, et al. Reduction of procedure time with active esophageal cooling during left atrial ablation in zero-fluoroscopy cases. J Am Coll Cardiol. 2022;79(9_Supplement):161. DOI: 10.1016/S0735-109701152-4.
- Joseph C, Zagrodzky JD, Zagrodzky W, et al. Reduced procedural duration with proactive esophageal cooling during left atrial ablation: a multicenter analysis - PO-681-08. Heart Rhythm. 2022;19(5):S364. DOI: 10.1016/j.hrthm.2022.03.510.
- Joseph C, Cooper J, Turer RW, et al. Reduced procedure time and variability with active esophageal cooling during radiofrequency ablation for atrial fibrillation. J Vis Exp. 2022(186). PMID: 36094261. DOI:10.3791/64417.
- Calkins H, Hindricks G, Cappato R, et al. 2017 HRS/EHRA/ECAS/APHRS/SOLAECE expert consensus statement on catheter and surgical ablation of atrial fibrillation. Heart Rhythm. 2017;14(10):e275–e444. PMID: 28506916.
- Cheng H, Clymer JW, Po-Han Chen B, et al. Prolonged operative duration is associated with complications: a systematic review and meta-analysis. J Surg Res. 2018;229:134–144. PMID: 29936980.
- Cheng H, Chen BP, Soleas IM, et al. Prolonged operative duration increases risk of surgical site infections: a systematic review. Surg Infect (Larchmt). 2017;18(6):722–735. PMID: 28832271.
- Medi C, Evered L, Silbert B, et al. Subtle post-procedural cognitive dysfunction after atrial fibrillation ablation. J Am Coll Cardiol. 2013;62(6):531–539. PMID: 23684686.
- Zagrodzky J, Bailey S, Shah S, et al. Impact of active esophageal cooling on fluoroscopy usage during left atrial ablation. J Innov Card Rhythm Manag. 2021;12(11):4749–4755. PMID: 34676132.
- Oseph C, Cooper J, Schricker A, et al. Use of an active esophageal cooling device in zero-fluoroscopy settings without intracardiac echocardiography. Eur Heart J. 2022;43(Supplement_2). doi:10.1093/eurheartj/ehac544.600.
- Steinbeck G, Sinner MF, Lutz M, et al. Incidence of complications related to catheter ablation of atrial fibrillation and atrial flutter: a nationwide in-hospital analysis of administrative data for Germany in 2014. Eur Heart J. 2018;39(45):4020–4029. PMID: 30085086.
- Ngo L, Ali A, Ganesan A, et al. Institutional variation in 30-day complications following catheter ablation of atrial fibrillation. J Am Heart Assoc. 2022;11(4):e022009. PMID: 35156395.
- Cheng EP, Liu CF, Yeo I, et al. Risk of mortality following catheter ablation of atrial fibrillation. J Am Coll Cardiol. 2019;74(18):2254–2264. PMID: 31672181.
- Meduri A, Bergandi L, Oliverio GW, et al. The cold eye irrigation BSS solution used during phacoemulsification reduces post-surgery patients discomfort preventing the inflammation. Eur J Ophthalmol. 2021:11206721211018377. PMID: 34011203
- Ma H, Sinha B, Pandya RS, et al. Therapeutic hypothermia as a neuroprotective strategy in neonatal hypoxic-ischemic brain injury and traumatic brain injury. Curr Mol Med. 2012;12(10):1282–1296. CMM-EPUB-20120723-6 [pii] PMID: 22834830.
- Aksu T, Golcuk S, Guler TE, et al. Gastroparesis as a complication of atrial fibrillation ablation. Am J Cardiol. 2015;116(1):92–97. PMID: 25933733.
- Akhtar T, Calkins H, Bulat R, et al. Atrial fibrillation ablation–induced gastroparesis: a case report and literature review. HeartRhythm Case Rep. 2020;6(5):249–252. PMID: 32461887.
- Joseph C, Daniels J, Kawasaki R, et al.: The effect of active esophageal cooling during left atrial ablation on postoperative chest pain – an updated survey of operators. AF Symposium 2022, New York, NY; 2022. doi: 10.1111/jce.15392
- Cooper J, Joseph C, Turer R, et al. Procedural impacts of active esophageal cooling during radiofrequency ablation in an academic medical center. In: AHA scientific sessions. Chicago IL; 2022.
- Creta A, Ventrella N, Providência R, et al. Same-day discharge following catheter ablation of atrial fibrillation: a safe and cost-effective approach. J Cardiovasc Electrophysiol. 2020;31(12):3097–3103. PMID: 33107171.
- Greer SK, Jeffe DB, Manga A, et al. 2022. Cognitive load assessment scales in simulation: validity evidence for a novel measure of cognitive load types. Simulation In Healthc J Simul Healthc. Publish Ahead of Print. PMID: 35470346. doi: 10.1097/sih.0000000000000665
- Park SH, Goldberg SA, Al-Ballaa A, et al. Objective measurement of learners’ cognitive load during simulation-based trauma team training: a pilot study. J Surg Res. 2022;279:361–367. PMID: 35816846.
- van Winsum W. The effects of cognitive and visual workload on peripheral detection in the detection response task. Hum Factors. 2018;60(6):855–869. PMID: 29791188.
- Sundaram S, Humer J, Alyesh D, et al. Creating a 5-step process to improve EP lab efficiency. EP Lab Digest. 2021; 1535–2226.
- Chu E, Zhang C, Musikantow DR, et al. Barriers and financial impact of same-day discharge after atrial fibrillation ablation. Pacing Clin Electrophysiol. 2021;44(4):711–719. PMID: 33686695.
- Cooper J, Joseph C, Turer R, et al.: Improved hospital discharge and cost savings with esophageal cooling during left atrial ablation. In: 5th Annual Architecture of High Value Health Care National Conference. Baltimore, MD; 2022 Oct 7-8.
- Yokokawa M, Chugh A, Dubovoy A, et al. A comparison of clinical outcomes and cost of radiofrequency catheter ablation for atrial fibrillation with monitored anesthesia care versus general anesthesia. J Cardiovasc Electrophysiol. 2022;33(8):1714–1722. PMID: 35652836.
- Di Monaco A, Gregucci F, Bonaparte I, et al. Paroxysmal atrial fibrillation in elderly: worldwide preliminary data of LINAC-based stereotactic arrhythmia radioablation prospective phase ii trial. Front Cardiovasc Med. 2022;9:832446. PMID: 35310997.