Abstract
Because of their specific properties (mechanical, electrical, etc), carbon nanotubes (CNTs) are being assessed for inclusion in many manufactured products. Due to their massive production and number of potential applications, the impact of CNTs on the environment must be taken into consideration. The present investigation evaluates the ecotoxic potential of CNTs in the amphibian larvae (Ambystoma mexicanum). Acute toxicity and genotoxicity were analysed after 12 days of exposure in laboratory conditions. The genotoxic effects were analysed by scoring the micronucleated erythrocytes in the circulating blood of the larvae according to the French standard micronucleus assay. The results obtained in the present study demonstrated that CNTs are neither acutely toxic nor genotoxic to larvae whatever the CNTs concentration in the water, although black masses of CNTs were observed inside the gut. In the increasing economical context of CNTs, complementary studies must be undertaken, especially including mechanistic and environmental investigations.
Introduction
Carbon nanotubes (CNTs) are hollow-1D objects with an extremely high aspect ratio (the ratio length/diameter ranges between 5.000 and 50.000) and a very high specific surface area (800–1000 m2.g−1). They can behave like metals or semiconductors depending on their structure. Because of their very specific properties (mechanical, electrical), CNTs are envisioned to be included in many manufactured products such as flat-screens, tyres, composite materials in general, special technical clothes, medical and pharmaceutical products. The industrial production of CNTs, currently on the ton-scale, is expected to increase steadily in the next decade. In 2003, Single-Walled Nanotubes (SWNTs) and Multi-Walled (MWNTs) Nanotubes had a worldwide production of over 2900 Kg (Borm et al. Citation2006). Double-walled carbon nanotubes (DWNTs) are at the frontier between SWNTs and MWNTs. The possibility to functionalize the outer wall to ensure the connections with the external environment, while retaining the remarkable mechanical and electronic properties of the inner nanotube, makes them very attractive for their integration into systems and composites (Flahaut et al. Citation2003).
Due to their massive production and utilization, CNTs must receive considerable attention as new, unknown and potentially hazardous materials at each step of their life-cycle. Moreover, potential extensive contamination of the environment must be examined. Indeed, the presence of these products in the environment at the end of their use and the presence of CNT-contaminated waste could lead in the near-future to ecotoxicity problems, which have hardly been evaluated to any extent. In spite of this economical and technological context, it is surprising that only very few studies have been published about their potential toxicity. The vast majority of the studies focusing on the impact of CNTs has been devoted to the study of the toxicity and immunotoxicity in in vitro cellular models on human or rat cells (Shvedova & Castranova Citation2003; Tamura et al. Citation2004, see for review Smart et al. Citation2006; Cui et al. Citation2005; Jia et al. Citation2005; Monteiro-Rivière et al. Citation2005; Muller et al. Citation2005; Salvador-Morales et al. Citation2006). Smart et al. (Citation2006) indicated that in the last five years, disturbing and often conflicting data have emerged concerning the safety of CNTs. To our knowledge no information is currently available about the toxic effects of such materials in in vivo studies, except the following studies on aquatic organisms: (i) Freshwater crustaceans (Daphnia magna and Hyalella azteca), a marine copepod and fish (Pimephales promelas and Oryzias latipes) exposed to C60 (fullerenes) (Oberdörster Citation2004a, Citationb; Oberdörster et al. Citation2006), (ii) on zebrafish embryos (Danio rerio) exposed to SWNTs and DWNTs (Cheng et al. 2007), (iii) on estuarine copepod (Amphiascus tenuiremis) exposed to SWNTs (Templeton et al. Citation2006) and (iv) on juvenile trout (Oncorhynchus mykiss) exposed to SWNTs (Smith et al. Citation2007). None of these four in vivo studies on aquatic organisms are devoted to the genotoxic effects of CNTs, although, among toxic actions, genotoxic effects may durably affect the aquatic ecosystems and the presence of genotoxic compounds in water can also have repercussions on non-aquatic species, via food chains, or simply as a result of drinking the water. One should therefore be aware of the hidden risks of potentially genotoxic substances in the aquatic environment. The interaction of genotoxic compounds with DNA initially causes structural changes in the DNA molecule. Unrepaired damage can generate other cell lesions and thus lead to tumour formation (Vuillaume Citation1987; Malins et al. Citation1990). A number of tests have been developed to assess the genotoxic potency of water samples, using either plants or aquatic animals (see the reviews of Jaylet et al. Citation1990; Godet et al. Citation1993). The assays can be carried out with intact animals, taking into account uptake and elimination, internal transport and metabolism. An example is the use of amphibians, which have proved to be valuable bioindicators and sensitive models for environmental studies (Jaylet et al. Citation1986; Krauter et al. Citation1987; Fernandez et al. Citation1993; Association Française de Normalisation [AFNOR] Citation2000). Larvae can be reared, not only in containers filled with unconcentrated water samples (laboratory conditions) but also in running water of various sources (factory effluents, surface water, river waters, etc.). In amphibian larvae, as in most eukaryotes, genome mutations may result in the formation of micronuclei, which are a consequence of chromosome fragmentation or malfunction of the mitotic apparatus. Thus, clastogenic compounds and spindle poisons both lead to an increase in the number of micronucleated cells. The micronucleus test has been widely used in many amphibian species in the laboratory (Pleurodeles waltl, Ambystoma mexicanum and Xenopus laevis) (Jaylet et al. Citation1986; Gauthier Citation1996; Ferrier et al. Citation1998; Gauthier et al. Citation2004; Mouchet et al. Citation2006). This method has been documented in French recommendations, using axolotl larvae (AFNOR Citation1987) and standardized in pleurodeles and xenopus larvae (AFNOR Citation2000). In particular, the ecotoxicological relevance of the axolotl larvae (Ambystoma mexicanum) has already been well established in studies focusing on micronucleus formation (Jaylet et al. Citation1986, Citation1990; Zoll et al. Citation1990; Gauthier Citation1996).
The present work constitutes a preliminary study on CNTs and in particular on DWNTs. To date, it is very difficult to evaluate any environmentally realistic CNTs concentrations. Working on water-soluble contaminants, the substance concentrations in water are quite easy to determine using classical analytical methods. In the present work, our strategy was based on a classical risk assessment method, to evaluate the potential risk of this new contaminant on the environment. In this way, a first step consists in determining the potential acute toxic effects of this new material on organisms, i.e. to search biological effects using the amphibian larvae. Because of the lack of preliminary data on the potential biological effects of CNTs on amphibians, amphibian larvae were exposed to a wide range of DWNTs concentrations, including very high concentrations (from 1–1000 ppm), in order to observe potentially toxic effects on our test-organism.
In the present investigation, axolotl larvae were then exposed to CNTs in controlled laboratory conditions to evaluate two different endpoints after 12 days of exposure according to the French standard micronucleus assay (AFNOR Citation2000): (i) Acute toxicity on larvae, and (ii) genotoxicity as the expression of the clastogenic and/or aneugenic effects of CNTs in erythrocytes of the running blood (appearance of micronucleated cells).
Another important aspect of this work lies in the characterization of the DWNTs exposed to amphibian larvae in order to highlight the potential toxic effects of this material.
Materials and methods
CNTs: Synthesis and material used in this study
The formula of the catalyst used in this work could be written as Mg0,99Co0,075Mo0,025O. However, X-ray diffraction (XRD) revealed a mixture of the Mg1-xCoxO solid solution with a very limited amount of an undetermined molybdenum oxide (MoOy). During the CCVD (Catalytic Chemical Vapour Decomposition) synthesis of the CNTs, the catalyst was reduced in a H2-CH4 mixture (18 mol.% CH4, heating and cooling rates 5°C/min, maximum temperature 1000°C, no dwell). This resulted in a dense mat of DWNT composite powder. Catalyst dissolution was achieved by HCl washing. In order to avoid any drying step, which would lead to irreversible agglomeration of the DWNTs, the exact amount of composite powder required to obtain a given final amount of DWNTs was treated with a concentrated aqueous HCl solution to separate the CNTs. The suspension was washed until neutrality with deionised water, on a cellulose nitrate filtration membrane (Flahaut et al. Citation2003). The required amount of reconstituted water was then added to prepare aqueous suspensions at different concentrations of DWNTs in g.l−1: 0.001; 0.01; 0.1; 0.125; 0.25; 0.5; 1.
Elemental analysis (flash combustion) of a dry sample of DWNTs gave a carbon content of ca. 90 wt%. This corresponds to more than 97.7 mol.% of carbon, assuming that the sample contains mainly Co and C. The remaining Co was assumed to be present only as carbon-encapsulated nanoparticles (Flahaut et al. Citation2000, Citation2002). The BET (Brunauer Emmett Teller) specific surface area was 985 m2.g−1. Raman analysis (λ = 488 nm, not shown) revealed that the ratio between the intensity of the D and G bands was close to 10%, corresponding to a good structural quality of the sample. Analysis of the radial breathing modes (which frequency can be easily associated to the diameter of the CNTs) indicated the presence of CNTs with diameters ranging from 0.7–2.13 nm. shows a representative FEG-SEM (Field Emission Gun-Scanning Electron Microscopy) image of the raw CNT sample (before removal of the catalyst), showing a very high density of CNT bundles, with extensive branching. Their diameter typically ranged between 10 and 20 nm but numerous individual CNTs were also present. No carbon nanofiber (a typical by-product of CVVD methods) was observed in the sample. HRTEM (High Resolution Transmission Electron Microscopy) observation was performed on the CNTs after elimination of the catalyst () and revealed clean CNT surfaces; as suggested by SEM, the CNTs are mainly isolated, or gathered into small bundles, mainly composed of DWNTs (not all with the same diameter). Electron diffraction data have confirmed these observations (Colomer et al. Citation2003). A few bundles are also made of CNTs with a different number of walls. Analysis of HRTEM images allowed the determination of the number of walls and inner and outer diameters. Data show that the main species (ca. 80%) is DWNTs, much more abundant than either SWNTs (15%) or triple-walled CNTs (5%). The inner and outer diameters range from 0.6–2.6 nm and from 1.2–3.2 nm, respectively.
Axolotl rearing and breeding
Sexually mature males and females were placed together in normal tap water at 18±2°C. A few hours later, the eggs were collected and placed in tanks containing 5–6 l of filtered tap water (100 eggs per tank). Viable eggs were maintained at 18°C, until they reached the development stage appropriate for experimentation (onset of formation of the two first digits). After hatching, the animals were fed on freshly hatched artemia (Artemia salina). One third of the water was renewed daily before feeding. When the young axolotl reached around 30 mm, the food was switched to frozen chironomid larvae.
Exposure conditions
The experimental exposure procedure was performed as indicated in the AFNOR recommendations (AFNOR Citation1987), according to the French Standard AFNOR NF T90-325 (AFNOR Citation2000) and the international standard ISO 21427-1 (ISO Citation2006) and subsequently adapted for more environmentally realistic exposure condition, i.e., without daily water renewal. The amphibian larvae were exposed in reconstituted water (distilled tap water to which nutritive salts were added (294 mg/l CaCl2.2H2O, 123.25 mg/l MgSO4.7H2O, 64.75 mg/L NaHCO3, 5.75 mg/l KCl). Ambystoma exposure began when the hind limb buds of the larvae exhibited slight indentations (onset of the formation of the two first digits). Two independent experiments were conducted but the larvae were taken from the same hatch to reduce inter-animal genetic variability within each experiment and between both experiments. In Experiment I, larvae were exposed in groups of 5 animals (100 ml/larva) in 2 l glass crystallizing dishes containing either the control medium (negative control) or the test medium (CNTs to 1, 10, 100, 125, 250, 500 and 1000 mg.l−1 in water). In Experiment II, larvae were exposed in groups of 13 animals (100 ml/larvae) in the same kind of flasks containing either the control medium (negative and positive controls) or the test medium (CNTs to 0.5 and 1 g.l−1 in water). The negative control was the reconstituted water alone. The positive control was cyclophosphamide (CP, [6055-19-2], Sigma France) in reconstituted water at 2 mg.l−1 (AFNOR Citation2000; ISO Citation2006). CP is a standard indirect mutagen requiring metabolic activation in liver prior to becoming effective. For each CNT condition, the mixtures were simply prepared by addition of an aqueous suspension of CNTs in the required amount of reconstituted water. The larvae were submitted to a 12 h light: 12 h dark cycle at 20°C±0.5°C. They were fed every day with frozen chironomid larvae.
Acute toxicity
Acute toxicity (death or abnormal behaviour) of larvae exposed to CNTs in both experiments was examined for 12 days (AFNOR Citation2000) by visual inspection, compared to the negative control group. Abnormal behaviour corresponds to reduced size and/or growth stop of larvae, reduced food uptake and abnormal motility.
Micronucleus test, genotoxicity assay
Genotoxicity was only evaluated in Experiment II. At the end of the 12 days of exposure, a blood sample was obtained from each anaesthetized larva by cardiac puncture with heparinized micropipettes (20% solution at 5000 IU/ml). After fixing in methanol and staining with hematoxylin, the smears were screened under the microscope (oil immersion lens, ×1000). The number of erythrocytes that contained one micronucleus or more (MNE) was determined in a total sample of 1000 erythrocytes per larva. All slides were scored by only one individual. Since micronucleus frequency was not normally distributed, median values and quartiles were calculated instead of means (McGill et al. Citation1978). For each group of animals, the results (number of micronucleated erythrocytes per thousand, MNE°/oo) obtained for each larva were arranged in increasing order of magnitude. The medians and quartiles were then calculated. The statistical method used to compare the medians consists in determining the theoretical medians of samples of size n (where n≥7) and their 95% confidence limits expressed by M±1.57×IQR/√n, where M is the median and IQR is the Inter-Quartile Range (upper quartile – lower quartile) (McGill et al. Citation1978). The difference between the theoretical medians of the test groups and the theoretical median of the negative control group is significant to within 95% certainty if there is no overlap.
Results
Acute toxicity of CNTs (Experiments I and II)
CNTs appeared to be not acutely toxic to Ambystoma larvae exposed in both experiments in all the investigated concentration range (from 1–1000 mg.l−1) ().
Figure 3. Results of mortality in larvae exposed to CNTs (mg.l−1) for 12 days in Experiments I and II. NC, negative control; PC, positive control (cyclophosphamide, CP 2 mg.l−1); n.e., non explored concentration; CNTs, carbon nanotubes. Mortality is expressed as the percentage of survival larvae (n=5 in Experiment I; n=13 in Experiment II). No mortality was observed in Ambystoma larvae exposed to CNTs, whatever the concentration and the experiment.
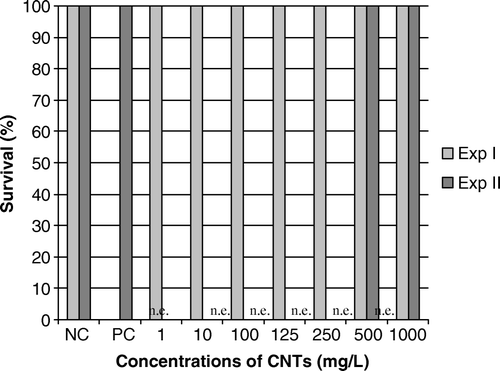
Genotoxicity of CNTs (Experiment II)
The median value of MNE‰ for the negative control was 7±1.31 (). The positive control showed significantly higher MNE‰ (32±4.35) compared to the negative control group. Results indicated that CNTs did not induce any genotoxicity (no micronucleus induction in erythrocytes of Ambystoma larvae exposed to the two CNTs concentrations tested). In both groups, the levels of MNE induced were 8 and 9‰ for 0.5 and 1 g. l−1, respectively. Moreover, MNE frequencies did not vary significantly between the two CNTs concentrations tested.
Figure 4. Results of the micronucleus assay in larvae exposed to CNTs (g.l−1) for 12 days in Experiment II. NC, negative control; PC, positive control (cyclophosphamide, CP 2 mg.l−1); CNTs, carbon nanotubes; Genotoxicity is expressed as the values of the medians (number of micronucleated erythrocytes per thousand, MNE°/oo) and their 95% confidence limits. No genotoxic effects were observed in Ambystoma larvae exposed to 0.5 and 1 g.l−1 of CNTs.
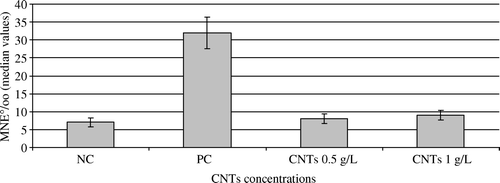
Discussion
During the last few years, research into the synthesis of CNTs has increased tremendously. A number of international research projects and activities are ongoing in the EU such as the Marie Curie Research Training Networks RTN (FP6, 2005) NANOTOX, IMPART. In the US, several research programmes are financed by the National Science Foundation and Environmental Protection Agency. In comparison with their increasing production and use, only few relevant data have been published these last five years concerning their potential toxicological effects (for review, see Borm et al. Citation2006). To date, the results have shown that their harmlessness is not demonstrated. For instance, effects of CNTs in rat were expressed as mortality (Warheit et al. Citation2004), pulmonary lesions (Lam et al. Citation2004), inflammatory responses (Muller et al. Citation2005) in relation with their distribution in organs (Wang et al. Citation2004) and their biopersistence (Muller et al. Citation2005). Considering the tons of engineered CNTs planned for production, and their integration in everyday products, it is likely that some of them will enter the environment during their product's Life Cycle (manufacture, use, and disposal). Their widespread use thus constitutes a potential risk of exposure for all living organisms in the environment. The findings of toxicology studies stress the need for work to characterize the potential impact of CNTs on the environment. Curiously, one of the most important areas of interest in environmental risk assessment of such new materials, i.e., the ecotoxicological field of research, remains totally uninvestigated and very little data on nanoparticles (including NTCs) have been published. Using for fullerenes (C60), Oberdörster (Citation2004a) showed that the 48-h LC50 was approximately 800 ppb for Daphnia magna, making them moderately toxic. This author also demonstrated that Micropterus salmoides exposed to up to 1 ppm (C60) showed no signs of mortality or morbidity after 48 h, although glutathione, a component of the antioxidant defence system, was depleted in the gill, and significant lipid peroxidation (LPO) was found in the brain (Oberdörster Citation2004b). Both the liver and gills presented decreased LPO, possibly due to up-regulation of repair enzymes (Oberdörster Citation2004b). Oberdörster et al. (Citation2006) highlighted the ingestion of fullerenes and their toxicity in Daphnia magna (alteration of mobility and reproduction) and in Pimphales promelas (reduction in the synthesis of proteins involved in lipid metabolism). Templeton et al. (Citation2006) demonstrated that copepods ingesting purified SWNTs showed no significant effects on mortality, development and reproduction across exposure whereas exposure to the more complex AP-SWNT mixture (partially purified and oxidatively processed to remove metallic and carbonaceous impurities in nitric acid, i.e., dispersible in water) significantly increased life-cycle mortality, reduced fertilization rates and reduced molting success in case of high exposure (10 mg/l). Cheng et al. (Citation2006) showed that exposure to raw SWCNTs induced a significant hatching delay in zebrafish embryos between 52 and 72 h post-fertilization (hpf) at concentrations of greater than 120 mg/l, but 99% of the exposed embryos hatched by 75 hpf. Raw DWNTs also induced a hatching delay at concentrations greater than 240 mg/l. These authors suggested that the hatching delay was likely induced by the Co and Ni catalysts used in the production of SWNTs. Smith et al. (Citation2007) showed that exposure of juvenile trout (Oncorhynchus mykiss) for up to 10 days to dispersed SWNTs (prepared in sodium dodecyl sulphate supported by a sonication step) caused respiratory toxicity (a dose-dependent rise in ventilation rate) and gill pathologies (oedema, altered mucocytes, hyperplasia). Other physiological effects (mucus secretion, changes of tissue metal level in brain and gill for Zn or Cu but attributed in part to the solvent, a significant increase in Na+K+-ATPase activity in the gills and intestine, a decrease of thiobarbituric acid reactive substances in the gill, brain and liver, an increase of the total glutathione levels in the gills and livers, etc.), some cellular disturbances (apoptotic bodies in liver cells, abnormal cellular division) were also observed. On the other hand, these authors observed no major haematological or blood disturbances (red and white blood cell counts, haematocrits, whole blood haemoglobin, and plasma Na+ or K+).
The present work demonstrated that this kind of CNTs deposited in water did not lead to any acute toxicity or to any induction of micronuclei in erythrocytes of axolotl larvae, whatever their concentration in the water. Further studies are needed using dispersed and functionalized CNTs on amphibian larvae since their behaviour and effects can be different. Nevertheless, visual observation showed that inside the gut there were large black masses of ingested CNTs even at the lowest concentration tested. In Ambystoma larvae, xenobiotics enter the body from the exposure medium in two principal ways: The dermal route and the digestive route leading to potentially high ingestion rates of particles, especially particles of food susceptible to vehicle agglutinated CNTs and water with suspended particles. Further studies are also needed on other amphibian species. Both hypotheses can be proposed to discuss these negative results: (i) DWNTs used in this study are neither toxic, nor genotoxic on Ambystoma larvae, (ii) lethality and abnormal behaviour are not adequate parameters for the evaluation of the acute toxicity of DWNTs and micronucleus is not a relevant biomarker to evaluate their genotoxicity. When looking at possible exposure routes for CNTs, other mechanisms of toxicity and genotoxicity must be explored. Indeed, results of in vitro studies on human cells showed that CNTs lead to reduced cellular viability (Shvedova et al. Citation2003; Cui et al. Citation2005; Monteiro-Rivière et al. Citation2005), various processes involving oxidative stress (Manna et al. Citation2005) and structural alterations in relation with apoptotic and necrotic processes (Shvedova et al. Citation2003; Cui et al. Citation2005; Bottini et al. Citation2006; Manna et al. Citation2005). Nevertheless, direct comparison of the present results with other results available in the literature must be carried out with precaution because: (i) Toxic and genotoxic results are likely to be dependent on the administration mode (peritoneal injection for rodents and water exposure for aquatic organisms) and on the possible route exposure for aquatic species in relation with their way of life and breeding (grazing, drinking, etc.) and with their biology (gill size, lung, etc.) or exposure stage (embryos, larvae, juvenile); (ii) the nature of CNTs is dependent on the nature of their synthesis (for a given kind of NCTs, i.e., SWNTs, DWNTs or MWNTs) and on the physical-chemical state (e.g., dispersion) when they are not used as pristine or raw; most of the published studies are poorly informative concerning the nature of the CNTs used since no or few physical-chemical characterization is carried out.
Conclusion
This preliminary work shows that DWNTs did not lead to acute toxicity or to the induction of micronuclei in erythrocytes of axolotl larvae, whatever their concentration in water. Nevertheless, visual observation showed that inside the gut there were large black masses of ingested CNTs even at low concentration and one of the further investigation would be to examine the persistence and bioaccumulation potential of DWNTs in the organisms.
Finally, this paper underlines that only few studies on the potential ecotoxic impact of CNTs have been investigated at this time, and further studies and extrapolation of the present data are needed to be able to clearly state that CNTs are safe for living organisms in the environment. In this context, more mechanistic and environmental investigations are needed to understand the potential risk of any given type of CNTs on the ecosystem and finally to help policy-makers with recommendations to develop methods for an appropriate regulation.
Acknowledgements
We are very grateful to Dr S. Jean-Dupuy for her help in fieldwork. We acknowledge the Service Commun de Microscopie à Transmission of the University Paul Sabatier for help with HRTEM characterization and Pr A. Peigney for SEM observation. We are very indebted to Anne Alibert for reviewing the English version of the manuscript.
References
- Association Française de Normalisation (AFNOR). 1987. Association Française de Normalisation (the French National Organization for quality regulations). Norme NFT 90-325. Essais des eaux. Détection en milieu aquatique de la génotoxicité d'une substance vis-à-vis de larves de batraciens (Pleurodeles waltl et Ambystoma mexicanum). Essais des micronoyaux. AFNOR Monograph – Paris, pp 12.
- AFNOR. 2000. Norme NFT 90-325. Qualité de l'Eau. Evaluation de la génotoxicité au moyen de larves d'amphibien (Xenopus laevis, Pleurodeles waltl). AFNOR – Paris, pp 17.
- Bottini M, Bruckner S, Nika K, Bottini N, Bellucci S, Magrini A, Bergamaschi A, Mustelin T. Multi-walled carbon nanotubes induce T lymphocyte apoptosis. Toxicol Lett 2006; 160(2)121–126
- Borm PJA, Robbins D, Haubold S, Kuhlbusch T, Fissan H, Donaldson K, Schins R, Stone V, Kreyling W, Lademann J, Krutmann J, Warheit D, Oberdorster E. 2006. The potential risks of nanomaterials: A review carried out for ECETOC. Review. Particle Fibre Toxicol 3–11.
- Cheng J, Flahaut E, Cheng SH. Effect of carbon nanotubes on developing zebrafish (Danio rerio) embryos. Env Toxicol Chem 2007; 26(4)708–716
- Colomer JF, Henrard L, Flahaut E, Van Tendeloo G, Lucas AA, Lambin P. Rings of Double-Walled Carbon Nanotube bundles. Nano Lett 2003; 3(5)685–689
- Cui D, Tian F, Ozkan CS, Wang M, Gao H. Effect of Single Wall Carbon Nanotubes on human HEK293 cells. Toxicol Lett 2005; 155(1)73–85
- Fernandez M, L'Haridon J, Gauthier L, Zoll-Moreux C. Amphibian micronucleus test(s): A simple and reliable method for evaluating in vivo genotoxic effects of freshwater pollutants and radiations. Initial assessment. Mut Res 1993; 292(1)83–99
- Ferrier V, Gauthier L, Zoll-Moreux C, L'Haridon J. Genotoxicity tests in amphibians – a review. Microscale Testing in Aquatic Toxicology: Advances. techniques and practice 1998; 35: 507–519
- Flahaut E, Peigney A, Laurent C, Rousset A. Synthesis of Single-Walled Carbon Nanotube-Co-MgO composite powders and extraction of the nanotubes. J Mater Chem 2000; 10: 249–252
- Flahaut E, Agnoli F, Sloan J, O'Connor C, Green MLH. CCVD synthesis and characterization of cobalt-encapsulated nanoparticles. Chem Mater 2002; 14: 2553–2558
- Flahaut E, Bacsa R, Peigney A, Laurent C. 2003. Gram-scale CCVD synthesis of Double-Walled Carbon Nanotubes. Chem Commun 1442–1443.
- Gauthier L. The amphibian micronucleus test, a model for in vivo monitoring of genotoxic aquatic pollution. Review Paper. Int J Batrachol 1996; 14(2)53–84
- Gauthier L, Tardy E, Mouchet F, Marty J. Biomonitoring of the genotoxic potential (micronucleus assay) and detoxifying activity (EROD induction) in the river Dadou (France), using the amphibian Xenopus laevis. Sci Total Environ 2004; 323: 47–61
- Godet F, Vasseur P, Babut M. Essais de génotoxicité in vivo et in vitro applicables à l'environnement hydrique. Rev Sci Eau 1993; 6: 285–314
- ISO. 2006. ISO International Standard. Water quality – Evaluation of genotoxicity by measurement of the induction of micronuclei – Part 1: Evaluation of genotoxicity using amphibian larvae. ISO 21427-1, ICS: 13.060.70, GENOVA – CH, Août 2006. 15 p.
- Jaylet A, Deparis P, Gaschinard D. Induction of micronuclei in peripheral erythrocytes of axolotl larvae following in vivo exposure to mutagenic agents. Mutagen 1986; 1(3)211–215
- Jaylet A, Gauthier L, Zoll C. Micronucleus test using peripheral red blood cells of amphibian larvae for detection of genotoxic agents in freshwater pollution. In situ evaluations of biological hazards of environmental pollutants, SS Sandhu, et al. Plenum Press, New York 1990; 71–80
- Jia G, Wang H, Yan L, Wang X, Pei R, Yan T, Zhao Y, Guo X. Cytotoxicity of carbon nanomaterials: single-wall nanotube, multi-wall nanotube and fullerene. Environ Sci Technol 2005; 39(5)1378–1383
- Krauter PW, Anderson SL, Harisson FL. Radiation-induced micronuclei in peripheral erythrocytes of Rana catesbeiana: An aquatic animal model for in vivo genotoxicity studies. Environ Mol Mutagen 1987; 10: 285–291
- Lam C, James JT, McCluskey R, Hunter RL. Pulmonary toxicity of single-wall carbon nanotubes in mice 7 and 90 days after intratracheal instillation. Toxicol Sci 2004; 77: 126–134
- Malins DC, Ostrander GK, Haimanot R, Williams P. A novel DNA lesion in neoplastic livers of feral fish: 2,6-diamino-4-hydroxy-5-formamidopyrimidine. Carcinogen 1990; 11(6)1045–1047
- Manna SK, Sarkar S, Barr J, Wise K, Barrera EV, Jejelowo O, Rice-Flicht AC, Ramesh GT. Single-walled carbon nanotube induces oxidative stress and activates nuclear transcription factor- kb in human keratinocytes. Nano Lett 2005; 5: 1676–1684
- McGill R, Tuckey J, Larsen W. Variations of box plots. Am Statist 1978; 32: 12–16
- Monteiro-Rivière NA., Nemanich RJ, Inman AO, Wang YY, Riviere JE. Multi-walled carbon nanotube interactions with human epidermal keratinocytes. Toxicol Lett 2005; 155(3)377–384
- Mouchet F, Gauthier L, Mailhes C, Ferrier V, Devaux A. Comparative evaluation of the genotoxicity of captan in amphibian larvae (Xenopus laevis and Pleurodeles waltl) using the comet assay and the micronucleus test. Environ Toxicol 2006; 21(3)264–277
- Muller J, Huaux F, Moreau N, Misson P, Heiler JF, Delos M, Arras M, Fonseca A, Nagy JB, Lison D. Respiratory toxicity of multi-wall carbon nanotubes. Toxicol App Pharmacol 2005; 207(3)221–231
- Oberdörster E. 2004a. Toxicity of nC60 fullerenes to two aquatic species. Daphnia and largemouth bass. Am Chem Soc Abstract.
- Oberdörster E. Manufactured nanomaterials (fullerenes, C60) induce oxidative stress in the brain of juvenile largemouth bass. Environ Health Perspect 2004b; 112: 1058–1062
- Oberdörster E, Zhu S, Blickley MT, McClellan-Green P, Haasch ML. Ecotoxicology of carbon-based engineered nanoparticles: Effects of fullerene (C60) on aquatic organisms. Carbon 2006; 44: 1112–1120
- Salvador-Morales C, Flahaut E, Sim E, Sloan J, Green MLH., Sim RB. Complement activation and protein adsorption by carbon nanotubes Mol Immunol 2006; 43: 193–201
- Shvedova AA, Castranova V, Kirsin ER, Murray AR, Schwegler-Berry D, Gandelsman VZ, Maynard A, Baron P. Exposure to carbon nanotube material: Assessment of nanotube cytotoxicity using human keratinocyte cells. J Toxicol Environ Health A 2003; 66(20)1909–1926
- Smart SK, Cassady AI, Lu GQ, Martin DJ. The biocompatibility of carbon nantubes. Carbon 2006; 44: 1034–1047
- Smith CJ, Shaw BJ, Handy RD. Toxicity of Single Walled Carbon Nanotubes on Rainbow Trout (Oncorhynchus mykiss): Respiratory toxicity, organ pathologies, and other physiological effects. Aqua Tox 2007; 82(2)94–109
- Tamura K, Takashi N, Akasaka T, Roska ID, Uo M, Totsuka Y, Watari F. Effects of micro/nano particle size on cell function and morphology. Key Eng Mater 2004; 254(6)919–922
- Templeton RC, Lee Ferguson P, Washburn KM, Scrivens WA, Chandler GT. Life-cycle effects of Single-Walled Carbon Nanotubes (SWNTs) on an Estuarine Meiobenthic Copepod. Environ Sci Technol 2006; 40: 7387–7393
- Vuillaume M. Reduced oxygen species, mutation, induction and cancer initiation. Mutat Res 1987; 186(1)43–72
- Wang H, Wang J, Deng X, Sun H, Shi Z, Gu Z, Liu Y, Zhao Y. Biodistribution of carbon single-wall carbon nanotubes in mice. J Nanosci Nanotech 2004; 4: 1019–1024
- Warheit DB, Laurence BR, Reed KL, Roach DH, Reynolds GA, Webb TR. Comparative pulmonary toxicity assessment of single-wall carbon nanotubes in rats. Toxicol Sci 2004; 77: 117–125
- Zoll C, Ferrier V, Gauthier L. Use of aquatic animals for monitoring genotoxicity in unconcentrated water samples. Mechanisms of environmental mutagenesis-carcinogenesis, A Kappas. Plenum Press, New York 1990; 233–244