ABSTRACT
Because of their outstanding mechanical properties, chemical stability, and biocompatibility, 3-mol % yttria-stabilised tetragonal zirconia polycrystals (3Y-TZP), known as zirconia ceramics in dentistry, are an important choice for various types of prosthesis. In addition to extensive use for crown and bridge construction, considerable interest has been generated for applications in implant dentistry, including full-contour zirconia crowns as supra-constructions, zirconia abutments, and novel zirconia implants. However, their use among dentist and researchers is controversial, especially compared with the well-established implants made of titanium alloys. As a latecomer, the merits and limitations of 3Y-TZP are awaiting careful investigation. Design, manufacturing, and clinical operation guidelines are urgently needed. The aim of this review was to address the present status of the application of zirconia ceramics related to implant dentistry by analysing the published data from both in vitro and in vivo studies. Suggestions are provided for potential improvements and suitable applications of zirconia ceramics in metal-free implant dentistry.
Introduction
Since Branemark et al. [Citation1] inserted the first titanium screw implant in a patient in 1965, titanium has been established as the preferred metal for dental implants. In the past 50 years, the use of titanium dental implants has been well-documented, with high survival and success rates. Titanium dental implants consist of roughly 97% of the total dental implant material market. Combined with titanium implants, metal abutments, and metal ceramic crowns are also applied to implant restoration. However, with more and more applications of this kind of implant system, concerns have been raised in relation to the use of metals.
Titanium hypersensitivity [Citation2–5] is a growing concern. Sicilia et al. [Citation6] reported that the prevalence of titanium allergy in 1500 dental implant patients was about 0.6%, which may induce implant failure by inhibiting implant integration. Although titanium allergy is uncommon, the appearance of significant complications in particularly sensitive patients cannot be disregarded [Citation7]. The accumulation of metal ions in the vicinity of dental and in regional lymph nodes has been verified, despite the excellent corrosion resistance of titanium. Poor aesthetic results are another problem of titanium implants and abutments (). The metallic colour cannot be fully hidden by the soft tissue peri-implant, which induces a greyish appearance, especially for patients with thin soft tissue [Citation8–10]. It has been demonstrated that over 60% of cases showed a colour mismatch between single implant restoration and natural tooth gingiva [Citation11]. After gingiva recession, the titanium implant becomes exposed [Citation11,Citation12]. Moreover, the release of metal ions could induce discolouration of gingiva after implantation in a slow and continuous way, leading to dissatisfaction with the long-term aesthetic results.
Figure 1. Aesthetic problems caused by titanium implants. (a) Gingiva appears greyish when the titanium implant is covered by relatively thin soft tissue. (b) Greyish implant is exposed after gingiva recession.
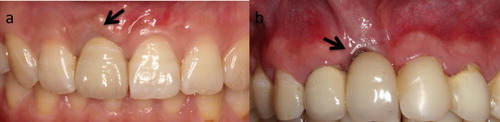
Although an appearance matching that of standard gingiva can be achieved by a bone-level titanium implant with a ceramic abutment, a metal-free implant system in which all parts are made from ceramics, without the potential hypersensitivity risk and using a material very similar to bone, is of particular interest. The 3 mol % yttria partially stabilised tetragonal zirconia (3Y-TZP), known as zirconia in dental applications, is the strongest and toughest material in the field of dental ceramics. It has high flexural strength ranging from 900 to 1200 MPa and fracture toughness of about 8–10 MPa m1/2. These properties suggest that zirconia has great potential for applications in implant dentistry [Citation13], although many doubts have been raised concerning the feasibility of its clinical application. In this paper, we systematically review the published literature on zirconia ceramics related to their application as implants, abutments, and restorations. It is hoped that our detailed analysis of the properties and especially the failures observed during in vitro and in vivo studies will inspire the development of improved material properties, the optimisation of manufacturing and clinical operation procedures, and establish the long-term success of zirconia ceramics in implant dentistry.
Zirconia as dental implants
The first ceramic implant, made of alumina, was developed to solve problems caused by metal implants. It was called the Tübingen implant and was introduced in 1974. Although it showed excellent biocompatibility and plaque adhesion properties, the alumina implant was withdrawn from the market because of frequent fractures and a poor survival rate [Citation13]. Zirconia has much better mechanical properties than alumina, and the first zirconia implant system (the Z-system®) was developed by Volz [Citation14]. It initiated the application of zirconia ceramics in implant dentistry.
The white colour of zirconia will not impair the aesthetic appearance even when gingival recession occurs or in the case of very thin gingiva [Citation9]. Carinci et al. [Citation15] conducted a genome-wide expression analysis and found that zirconia can modulate immunity, which means a low level of immunity reaction. Zirconia was successfully used as a substitute for titanium in patients who exhibited hypersensitivity after titanium implantation [Citation16]. Zirconia implant systems have additionally demonstrated good integration ability with both hard and soft tissues. Various surface modifications of zirconia implants can improve osteoblast cell attachment, differentiation, and bone–implant contact [Citation17]. The three main strategies applied to date are surface roughening, surface coating with active compositions [Citation18,Citation19] to change the bio-inert surface to a bio-active one, and decreasing surface contamination to increase surface hydrophilicity. The application methods include surface treatments by acid-etching [Citation20], oxygen plasmas [Citation21], ultraviolet irradiation [Citation22,Citation23], or hydrogen peroxide [Citation24]. shows that a much rougher surface can be generated from an already rough surface of zirconia implants formed by traditional computer numerical controlled milling. Many studies have revealed that zirconia implants can establish similar or even better osseointegration than titanium implants. Schultze-Mosgau et al. [Citation25] and Dubruille et al. [Citation26] observed higher bone-to-implant contact ratios with zirconia than with titanium implants. Peri-implant bone volume density was superior with submerged zirconia implants than that with titanium implants [Citation27].
Figure 2. Two types of surface microstructural features of zirconia implants (magnification ×2000). (a) Surface roughened by sandblasting with 110-μm alumina particles at 4-bar pressure. (b) Porous surface developed by sintering coarse zirconia particles.
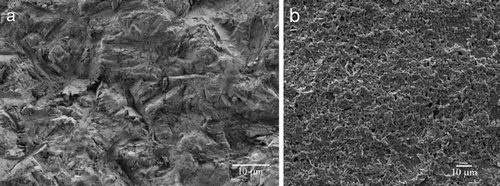
Soft tissue integration is very important to the stability of the peri-implant tissues and the prevention of peri-implantitis. In vitro, zirconia showed reduced bacterial colonisation, plaque accumulation, improved fibroblast attachment and growth, and reduced inflammation response, which indicated good soft tissue integration [Citation28]. Medeiros et al. [Citation29] reviewed the peri-implant soft tissue in contact with zirconia abutments; the results revealed gingival soft tissue attachment. The bacterial attachment and biofilm formation and the healthy condition of the gingiva were found to be comparable or superior to those of a titanium abutment. Linkevicius et al. [Citation30] reviewed the influence of abutment material on the stability of peri-implant tissues in 182 patients. They showed that a zirconia abutment could maintain an equivalent bone level and soft tissue reaction to those of titanium, gold, and alumina abutments. Clinical follow-ups of 1121 patients also indicated that zirconia abutments had similar biological outcomes compared with titanium abutments, namely, the plaque index, probing bleeding index, and tissue health were the same. Furthermore, the zirconia abutment showed less soft tissue recession compared with the titanium abutment [Citation31,Citation32].
Titanium implant is traditionally designed as a two-piece system: bone-anchored body part and abutment are manufactured separately. They are screwed together after implant insertion or even after the establishment of osseointegration. A similar two-piece zirconia implant system has been developed () [Citation33,Citation34]. The two zirconia parts, namely, the abutment and the implant, are adhered together. However, once a zirconia abutment fails, it is difficult to remove from the implant or repair. The fabrication of the two ceramic parts with high precision is a technical challenge because of the brittleness of the ceramic. A gap present between the body part and the abutment increases the risk of bacterial accumulation and thus increases the risk of peri-implant inflammation reaction and bone loss [Citation35]. Therefore, this kind of construction does not prevail in the marketplace.
Figure 3. Zirconia implant systems. The two at the left are zirconia abutment and zirconia implant bodies, which can be adhered together as a two-piece zirconia implant system. The two at the right are different designs of one-piece zirconia implants.
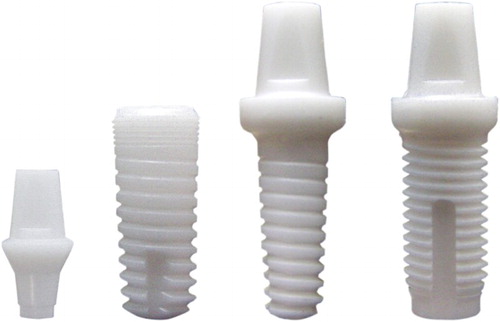
The zirconia implants discussed below are one-piece systems in which the bone-anchored body and abutment are fabricated as an integrated unit (). The static fracture strength of a one-piece zirconia implant ranges from 725 to 850 N, which is within the limits of clinical acceptance [Citation36]. Fatigue does not influence the lifetime of ceramic implants at loads under 600 N [Citation37]. In a 4-year follow-up study of the combination of one-piece zirconia implants and crowns, no failures, such as pain, paresthesia, and peri-implantitis, were reported [Citation38]. Nevertheless, Depprich et al. [Citation39] reviewed the available clinical data on zirconia implants published between 2006 and 2011 and found that the survival rates of such implants ranged from 74 to 98% after 12–56 months, and the success rates ranged from 79.6 to 91.6% after 6–12 months of function. Koller et al. [Citation40] reported survival rates of titanium implants ranging from 97 to 100% after 3–10.4 years. These data suggest that the reliability of zirconia implants is currently inferior to that of titanium implants. The main failure modes are early implant loss and fracture. Excessive bone loss is also observed in zirconia-restored cases.
Implant loss and fracture
Osman et al. [Citation41] reported a fracture rate of zirconia implants of 4.1% after 1 year. A similar percentage (5.4%, two of 37 cases) of one-piece zirconia implants fractured during the final torquing in the maxillary ridge using the prescribed hand torque wrench [Citation42]. Fracture during the torquing and functioning may be related to the design of the implant and its operation. Zirconia is strong under compressive stress, but it is sensitive to shear and tensile stresses. The greater the angle between the biting force direction and the implant axis, the greater the shear stresses and an enhanced risk of zirconia implant fracture [Citation41–43].
The strength of zirconia is process-sensitive and can be easily deteriorated by any defects introduced during the entire processing chain, for example, powder granulation, dry-pressing, machining, and surface treatments [Citation44]. The primary defects include bulk defects such as voids and hard aggregates, surface defects such as notches, scratches, and cracks, and local stress concentrations induced by phase transformations. These defects can be exacerbated by environment conditions and clinical function, which will lead to mechanical overloading or crack growth during chewing loads, and finally cause fracture of the implants [Citation45].
The clinical study by Dahlert et al. agreed with this finding [Citation43]. They reported that the fracture rate of one-piece zirconia implants was nearly 10%, where 13 of 170 inserted implants fractured with an average in situ period of 36.75 ± 5.34 months. Among the failed implants, 12 of them were diameter-reduced implants (3.25 mm in diameter), which were too thin to withstand high loads. The other case was an implant having a normal diameter, but the patient was suffering from bruxism. All failures were mechanical overloading by bending forces. The origin of the fracturing was sandblasting-induced surface defects and stress concentrations. Therefore, it is important to improve the manufacturing processes to reduce serious defects. Suitable indications and designs are also needed.
Excessive marginal bone loss
Kohal et al. conducted two 1-year prospective cohort studies on one-piece zirconia implants. When a zirconia implant was used to replace a single tooth, the average marginal bone loss was 1.31 mm after 1 year among 65 patients, 34% of which was at least 2 mm, and 14% of which was more than 3 mm [Citation46]. In another study, when zirconia implants supported a three-unit fixed dental prosthesis, the average marginal bone loss was 1.95 mm after 1 year. In 40% of the cases, the bone loss was more than 2 mm, and in 28% cases the bone loss was more than 3 mm, which was more serious than for the single-tooth implantation [Citation47]. In another study conducted by Borgonovo et al. [Citation38], a high marginal bone loss of 1.79 mm was also observed during the first year after implant insertion. However, some new bone formation was evident at 12–24 months, and the marginal bone level remained stable in the following 2 years.
This phenomenon might be ascribed to four factors: surface roughness of the implant, the elastic modulus mismatch between the zirconia implant and natural bone, the shape of the implant, and the torque used during insertion. First, the surface roughness of zirconia implants is always lower than that of titanium implants for a given surface treatment. A moderately rough surface is beneficial for osseointegration; hence, zirconia implants with smooth surfaces might integrate relatively weakly with bone tissues, which would further lead to high marginal bone loss [Citation41]. Second, the elastic modulus of 3Y-TZP is 210 GPa, compared with 104 GPa for titanium and 18 GPa for human bone. Theoretically, the greater the discrepancy between the elastic modulus of the implant material and the bone tissue, the higher the stress concentration in the peri-implant bone; this may induce more marginal bone loss [Citation44]. However, this hypothesis has been questioned. A finite element analysis showed that there was no significant difference of the stress–strain distribution between zirconia and titanium implants supporting maxillary overdentures [Citation48]. Several studies reported similar stress distributions of the stress level in bone and in implants at the bone/implant interface for zirconia and titanium implants [Citation49–52]. The third possible reason might be the tapered form of the implant or residual cement irritation [Citation35]. Finally, frequently increased bone loss may result from overtorquing during insertion. A high stress concentration at the neck of the implant would lead to bone trauma and loss [Citation46].
Zirconia as dental abutment
As the transmucosal component in the implant system, the abutment is exposed to the oral cavity directly or indirectly. Therefore, its appearance has a major impact on the aesthetics. Using zirconia abutments has indeed improved the aesthetics. In an 11-year follow-up study of zirconia implant abutments, Zembic et al. [Citation53] reported excellent long-term outcomes in anterior and premolar regions. As noted above, a zirconia abutment is rarely applied separately when a zirconia implant is chosen. However, because of its important aesthetic effect and the frequent application with titanium implants, the issues related to the combined titanium implant–zirconia abutment applications are discussed below.
Structural design
Zirconia abutments are available in two basic designs. The first is a one-piece abutment (), in which all parts are made of zirconia in a unit and the abutment is connected to the implant directly. The other design is the two-piece abutment introduced by Brodbeck [Citation54] (), which includes a secondary metallic component as the connecting part. The zirconia structure is adhered to the metallic component and then mounted onto the implant. Many studies have verified that the fracture strength of two-piece abutments is higher than that of the one-piece design [Citation55–57]. The fracture of the one-piece zirconia abutments always occurs under relatively low loads and before damage or plastic deformation of the screw or implant [Citation56,Citation58]. In an 11-year follow-up study, no one-piece zirconia abutment fractured, but two screws loosened [Citation53]. This was considered as a drawback of the external hexagon implant system. Meanwhile, severe wear between a zirconia abutment and titanium implant could induce loosening of the abutment connection, which could further increase the wear between the abutment and implant. Furthermore, the titanium wear debris may induce peri-implant gingivitis, gingiva discolouration, or marginal bone adsorption.
Figure 4. One-piece zirconia abutment for titanium implants. (a) All components have a direct connection via a tightening screw of the one-piece zirconia abutment to the titanium implant. (b) (c) A photograph and an SEM image of the cross-section of the one-piece zirconia abutment/titanium implant system connected by a central screw. Misfit at the interface between the zirconia abutment and the titanium implant is evident.
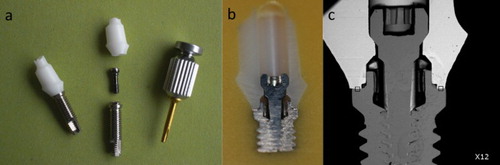
Figure 5. Two-piece zirconia abutment for titanium implants. (a) The two components of the two-piece zirconia abutment: the metallic titanium base and the zirconia superstructure. (b) The two components were glued together as a unit, which is then connected to the titanium implant with a central screw. (c) Computer-aided design of a customised two-piece zirconia abutment.
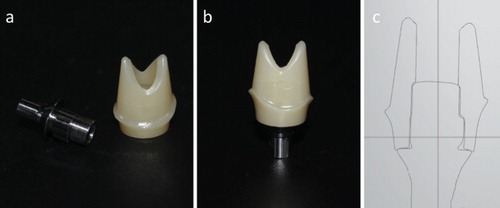
Metal has a higher ductility than zirconia and can withstand higher tensile forces through elastic deformation. Therefore, deformation of the metallic parts, sometimes followed by abutment fracture and screw loosening, is the main fracture mode of two-piece abutments [Citation56]. Meanwhile, a metallic part will protect the interface between the abutment and implant from serious wear, especially when micromotion is present [Citation59]. For two-piece abutments, the interface between the zirconia and metallic parts is the weak link. Gehrke et al. [Citation60] noted that complete adhesive failure was the main failure mode of a two-piece abutment, leaving the detached zirconia coping and the titanium insert undamaged. Although this finding has not been frequently verified in a clinical context, it indicates that the resin cement should be carefully chosen and adhesion optimised under laboratory conditions [Citation61].
Rosentritt et al. [Citation61] believed that the weakest point is not the bonding connection but rather the design of the connecting parts. Generally, the type of connection can be divided into the external connection and the internal connection. With the external connection system, there is an external anti-rotation mechanical structure on the implant shoulder for connecting the abutment. With the internal connection system, the connection formed through inserting the extending part of the abutment into the interior of the implant. The internal connection is more popular than the external one because it better distributes the load and thereby provides higher fracture strength [Citation56,Citation62]. In the internal connection system, the abutment connector can have various geometries, including hexagonal, octagonal, and conical (Morse taper) shapes. Schmitt et al. performed a careful review of the performance of these connections [Citation63]. Although there was no significant difference among them in terms of implant success and survival rate in in vivo studies, several advantages have been found for the conical design. Conical connection systems seem to be good at bacterial sealing, inhibiting micromotion, resisting torque loss, and reducing abutment screw stresses and load distribution. A 12-year follow-up study of zirconia abutments with different connection designs showed that both survival and success rates of standard platform abutments were higher than those of platform switching abutments [Citation64]. For the platform switching design, there is abutment fracture risk because the thickness of the abutment is reduced. However, there remain many opposing views, warranting additional scientific studies and clinical tests.
Thickness and angulation
Aboushelib and Salameh [Citation65] reviewed several clinical fracture cases of zirconia implant abutment. Two abutments fractured because of over-reduction of the axial walls. Additionally, rotational load fatigue testing performance of zirconia abutments is dependent on the abutment diameter [Citation66]. To resist the applied functional loads, the minimal wall thickness should not be reduced beyond 0.5 mm. For the one-piece zirconia abutment, when the abutment is sufficiently thick (i.e. >0.7 mm), further increasing the thickness will not further increase the strength [Citation67]. However, for the two-piece zirconia abutment, strength and thickness are positively correlated [Citation68]. From this perspective, insufficient thickness may be a deficiency of the internal connection abutment. In Ferrari’s study [Citation69], for same-diameter implants, the thickness of the zirconia abutment with the internal hexagonal connection was limited to 177–370 μm, but the thickness of the zirconia abutment with the external hexagonal connection was about 1 mm, which is thick enough to resist torque stress to 25 N cm−1 as well as occlusal stresses.
One important function of angulated abutments is to adjust the direction of the implant for the final restoration. Concerning one-piece zirconia abutments, angulated ones had lower fracture strength than straight designs [Citation67]. Compared with the buccal side, tilting the implant apex to the lingual side significantly reduced the strength of the angulated zirconia abutment because the lingual surface could better withstand tension during loading. Decreasing the angle between the occlusal force and the long axis of the implant lowers the fracture strength [Citation70]. However, for the two-piece abutments, the results were opposite: the angulated abutments exhibited higher strength than the straight ones at the same thickness [Citation68]. One possible explanation for this is differing force distributions in the two abutment systems.
Manufacturing processes
Two kinds of one-piece zirconia abutment, the prefabricated ones and the custom-made ones, are available. The former designs are uniform and standardised, but if the position or angulation of the fixture is not appropriate or if the height of the surrounding soft tissue is insufficient, this fully dense abutment is difficult to grind. Material loss and defect generation will further reduce the fracture strength [Citation71]. Custom-made zirconia abutments are fabricated according to individual models. The model or implant fixture in the mouth is digitised and the abutment is digitally designed to take into account the occlusion/angulation/emergence profile and provide room for the final aesthetic restoration.
Both customised and prefabricated standard zirconia abutments have comparable fracture strengths [Citation72]. The strength is sufficiently high to restore anterior teeth, but more attention should be paid when the abutment is located in the posterior area [Citation73].
Park et al. [Citation74] found that the abutment/implant interface was the weakest area of one-piece zirconia abutments. A microgap in this region cannot always be completely avoided. An irregular or large microgap can lead to mechanical and biological injury, such as screw loosening or fracture, bacterial accumulation, and peri-implant bone deficiency [Citation75]. Titanium and zirconia abutments were reported to have similar initial leakages, but after 195 h the leakage for zirconia abutments was almost nine-fold higher [Citation76]. Several studies reported that the dimensional accuracy of customised abutments is inferior to that of prefabricated ones [Citation72,Citation74,Citation77]. Microgap at the customised abutment–implant interface is larger than that at the prefabricated abutment–implant interface. Although a microgap is clinically acceptable, the precision of the design and processing should be further improved to strengthen this weak region. For the customised two-piece zirconia abutment, the misfit problem is less obvious because the connecting metallic part is prefabricated.
Abdelhamed et al. [Citation76] reported a clear correlation between torque and microleakage for zirconia abutments. Higher applied torque can effectively reduce the microgap between the zirconia abutment and the implant and thereby reduce the risk of microleakage and inflammation [Citation76,Citation78,Citation79]. Rosentritt et al. [Citation61] suggested that sufficiently high torque moments and early re-screwing in a clinical context be used to reduce the microgap and risk of screw loosening. More attention should be paid when tightening a zirconia abutment than a titanium one; Carrillo reported two zirconia abutments that fractured when tightened at the required torque [Citation80].
Zirconia abutments are typically manufactured by manual copy-milling and computer-aided milling. The raw material can be presintered blocks or green bodies. Injection moulding has also been used to obtain parts having excellent strength. Yang et al. [Citation81] produced one-piece zirconia abutments by this technique and loaded them with forces from different angles to simulate the real fracture risk of anterior teeth. They found that zirconia abutments with titanium implants are sufficiently strong for clinical application.
Full-contour monolithic zirconia restorations
The initial application of zirconia in dentistry was to replace metal as copings. However, a major issue occurred as porcelain-fused-to-zirconia restorations became increasingly applied in clinical settings. Chipping of veneering porcelain frequently occurs and it is the primary cause of restoration failure. Full-contour monolithic zirconia restorations without veneer () were proposed as a possible solution to this issue [Citation82]. In metal-free implant dentistry, full-contour zirconia restorations may have more opportunities than previously. However, concerns remain for the application of zirconia as material for full-contour restorations. The issues mainly relate to the following three aspects: (1) aesthetics, (2) wear, and (3) long-term reliability.
Figure 6. Full-contour zirconia crown supported by an implant (Erran Self-glazed Zirconia®). (a) A screw-retained monolithic zirconia crown. The hole provides a pathway to inspect and tighten the screw even after restoration. (b) A customised crown/abutment construction. It will be adhered to a secondary metallic base and then connected to the implant.
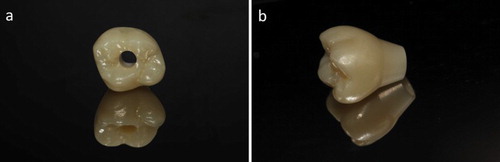
Aesthetics
To mimic the natural look of teeth, two important optical effects should be reproduced: colour and translucency. In 1998, Cales [Citation83] proposed that zirconia ceramics could be coloured by doping with special transition metal and rare-earth oxides to reproduce the colour of natural teeth. The most common method is to dip presintered zirconia crowns into the staining solution. The final colour appears after drying and complete sintering [Citation84]. However, the final colour always varies because of the influence of many factors, including the porosity of the zirconia blocks [Citation85], concentration of the colouring liquid [Citation84], immersion time [Citation86], and sintering temperature [Citation87]. The colour difference cannot be distinguished before sintering, so the dental technician cannot adjust it during the processing procedure. Thus, precise colour reproduction is very challenging by this approach. The zirconia microstructure is also affected by the colouring oxides. The pigments may segregate inside the grain boundaries or deposit on the outer surface of the products, yielding elongated grain structures, surface lifts, and numerous small pores [Citation88]. These changes are detrimental to the strength, and can occur especially when the concentration of the dipping solution is high [Citation89–91] or the shading time is prolonged [Citation91]. An alternative colouring method is to dope zirconia powders or blocks directly with metal oxides. This can be done at the industrial scale with good colour precision, and coloured zirconia blocks are readily available.
Translucency is the second most important character of natural teeth. Heffernan et al. [Citation92] and Chen et al. [Citation93] studied the translucency of several all-ceramic systems. Zhang [Citation94] analysed the factors affecting the translucency of zirconia in detail, and suggested that a nanocrystalline structure was needed to enhance the density, eliminate pores, and provide translucency. Matsuzaki et al. [Citation95] produced monolithic translucent zirconia having translucency comparable to that of porcelain. In addition, Klimke et al. [Citation96] successfully developed transparent zirconia with very high in-line transmission, which would completely satisfy the restorative requirement. Intense shading can reduce the translucency of zirconia [Citation97], which is an additional problem that needs to be resolved.
Wear performance
The ideal restoration should have good wear-resistance and not induce excessive wear of the opposite natural teeth. Zirconia is a very stiff ceramic and has wear resistance that is much better than those of both human enamel and porcelain. Whether zirconia induces significant wear on the opposite natural teeth, especially when it is not veneered, has been studied. Oh et al. [Citation98] analysed various factors related to the abrasion of enamel by dental ceramics and found no obvious relationship between the hardness of the ceramics and enamel abrasion. The microstructure, roughness of the contact area of the ceramics, and the patient’s intrinsic risk factors are important. For full-contour zirconia crowns, proper surface treatment is particularly important. Beuer et al. [Citation99] suggested that glazing is an effective method to maintain the wear resistance of zirconia and to avoid excessive abrasion of opposite natural teeth. However, this 30–50-μm-thick glazed layer is destroyed or gets worn out after 6 months and the exposed rough surface can then lead to increased contact wear. Polishing can provide zirconia with a very smooth surface, like that obtained by glazing, and further enhances the wear resistance of zirconia. However, full-contour zirconia polished by a 3-μm diamond paste failed to protect the enamel from excessive abrasion. Jung et al. [Citation100] and Wang et al. [Citation101] agreed with Beuer that glazing is not effective to protect the opposite natural teeth during long-term clinical service. Once the glazed layer has been destroyed, the rough contact surface having several acute angles will increase the local frictional stress and enamel wear. In their studies, fine polishing with 10-μm SiC polishing papers or using diamond rubber burrs with 106–125- and 20–30-μm grain sizes did effectively reduce enamel abrasion. Many other studies also verified that finely polished zirconia will not induce excessive abrasion of natural teeth and even provide better protection than other dental ceramics having different surface treatments [Citation102–106]. A 1-year follow-up study revealed that polished full-contour zirconia crowns led to less wear of antagonist enamel, 42.10 ± 4.30 μm for premolar and 127.00 ± 5.03 μm for molar, than porcelain-fused-metal crowns, which were 69.20 ± 4.10 μm for premolar and 179.70 ± 8.09 μm for molar. However, the wear was much more than for natural teeth, which was about 30–40 μm in the posterior area [Citation107]. Recently, good wear performance of a new product, called Erran Self-glazed Zirconia®, has been reported [Citation108]. This new grade of zirconia has a gradient microstructure with a naturally formed smooth surface that mimics the structure of tooth enamel, which helps protect the opposite natural teeth. The two SEM images shown in reveal the surface structure difference between the self-glazed surface and the conventional milled surface. Long-term clinical follow-up studies are needed to determine the wear behaviour of full-contour zirconia crowns fabricated by various techniques and to identify the optimal solution.
Long-term reliability
Low-temperature degeneration (LTD) of zirconia has long been a concern of dentists. Unlike the rapid stress-induced phase transformation that affects toughness, a spontaneous slow transformation from the tetragonal phase to the monoclinic phase may occur in zirconia grains at relatively low temperatures and in a humid environment. This aging phenomenon influences the long-term reliability. Possible LTD mechanisms of zirconia are classified into four categories: (1) corrosion, (2) destabilisation, (3) stress-induced transformation, and (4) alternative mechanisms [Citation96,Citation109]. Although no single mechanism is completely accepted, it is established that the aging does begin with contact with water. Papanagiotou et al. [Citation110] found that the loss of stabilising Y3+ ions does occur during aging. Thus, full-contour zirconia crowns without veneering porcelain may face an increased risk of aging.
Flinn et al. [Citation111] investigated the aging resistance of three commercial 3Y-TZP zirconia grades: Lava, Zirkonzahn, and Zirprime. After aging for 200 h in an autoclave at 134 °C and 0.2 MPa, the monoclinic phase content was 25–80%, the depth of the transformed zone ranged from 2 to 60 μm, and the biaxial flexural strength was 100–700 MPa. The study indicated that the aging resistance of zirconia is closely related to the properties of the starting materials, processing techniques, and microstructure. Reducing the grain size of zirconia could reduce the thermodynamic driving force of the phase transformation and enhance the stability of the tetragonal phase, thereby improving the aging resistance [Citation109]. Chen et al. [Citation112] and Munoz-Saldana et al. [Citation113] found that aging could be effectively inhibited when the grain size was equal to or less than 0.35 μm. However, the reduced grain size might accelerate aging through forming monoclinic crystal cores at the grain boundaries. This negative effect might be avoided by increasing the density [Citation114]. The relationship between sintering temperature and aging resistance of zirconia has also been identified [Citation115]. A higher sintering temperature increases the rate of phase transformation and creates a rougher microstructure. Hallmann et al. [Citation116] found that zirconia ceramics sintered at 1350–1450 °C had better aging resistance because the grains were relatively small and round and the stress distribution was more homogeneous. The tensile/compressive stress ratio increased and the monoclinic phase content increased with increasing sintering temperature. Doping is another way of improving the stability; adding elements such as Al, Ce, and Si has been studied. The mechanisms associated with the deceleration of LTD by doping alumina and silica and their combined effect were investigated [Citation117]. In alumina, the dopants were segregated at the grain boundaries and strengthened the grain boundaries to limit cracks. In silica, they were mainly located as an amorphous phase at the multiple grain junctions and reduced the strain within the grains to prevent nucleation and propagation of LTD. These characteristics were also observed in co-doped zirconia samples. In summary, it appears that the zirconia recommended for full-contour crowns should have small and homogeneous grains, relatively high density, be sintered at low temperature, and combined with effective dopants. Defects such as those that would lead water into the bulk should be critically controlled.
The aging resistance of coloured zirconia has not yet been rigorously studied. Ardlin [Citation118] found that the bending strength of coloured zirconia after aging did not greatly change even though the monoclinic phase content increased slightly. All of these results confirmed that the strength of zirconia after aging is still sufficient to withstand the loads in the posterior area, although the strength is slightly reduced by aging. Clearly, the aging resistance of zirconia, coloured or uncoloured, is not of great concern in dentistry applications [Citation119].
Application with dental implants
For implant-supported crowns, monolithic crowns exhibit higher fracture strength than conventional veneered ones. Among different materials, monolithic zirconia crowns performed the best [Citation120]. An in vivo follow-up study confirmed this finding, since chipping frequently occurred [Citation59]. The thickness of zirconia crowns definitely influences the fracture behaviour [Citation121]. For tooth-supported monolithic zirconia crowns, those with a 0.5-mm-wide chamber and 0.5-mm occlusal thickness could fulfil the fracture resistance requirement for posterior areas. However, Lan et al. [Citation122] suggested that a 0.7-mm occlusal thickness is necessary when applied with an implant. This difference may relate to the loss of periodontal receptors in the implant and the relatively high sensitivity to load compared with natural teeth [Citation123]. As discussed above, rescrewing after a complete restoration in a clinical context is beneficial to reduce screw loosening. Screw-retained crowns are recommended rather than cement-retained ones. A monolithic zirconia crown with an occlusal/lingual opening hole provides a screwing pathway and enables the screw to be checked, retightened, and even easily replaced without destroying the crowns ((a)). A passive fit is also suggested for implant-supported crowns because of lower mobility than natural teeth. Karl et al. found that full-contour monolithic restorations had better passive fit than veneered restorations, and that copy-milled ones had fewer strains than those prepared by CAD/CAM. These findings suggest that the precision of design needs improvement [Citation124]. To date, there have been very few clinical studies concerning monolithic zirconia crowns supported by implants; this should be remedied.
Fabrication of a zirconia crown/abutment unit ((b)) may be feasible using advanced CAD/CAM processing. This device would improve the precision and mechanical properties of the implant system.
Conclusion
Zirconia ceramics have been well received in implant dentistry, especially for abutments and full-contour crowns. However, there remains a need to improve the processing method to minimise processing defects, raise fabrication accuracy, and increase material reliability, and a need to improve the esthetics and surface roughness. There is insufficient scientific clinical data to recommend using zirconia implants for routine clinical use.
Disclosure statement
No potential conflict of interest was reported by the authors.
Additional information
Funding
References
- Branemark PI, Hansson BO, Adell R, et al. Osseointegrated implants in the treatment of the edentulous jaw experience from a 10-year period. Scand J Plast Reconstr Surg Suppl. 1977;16:1–132.
- Muller K, Valentine-Thon E. Hypersensitivity to titanium: clinical and laboratory evidence. Neuro Endocrinol Lett. 2006;27(Suppl. 1):31–35.
- Lhotka CG, Szekeres T, Fritzer-Szekeres M, et al. Are allergic reactions to skin clips associated with delayed wound healing? Am J Surg. 1998;176(4):320–323. doi: 10.1016/S0002-9610(98)00197-4
- Merritt K, Rodrigo JJ. Immune response to synthetic materials. Clin Orthop Relat R. 1996;326:71–79. doi: 10.1097/00003086-199605000-00009
- Valentine-Thon E, Schiwara HW. Validity of Melisa for metal sensitivity testing. Neuro Endocrinol Lett. 2003;24(1–2):57–64.
- Sicilia A, Cuesta S, Coma G, et al. Titanium allergy in dental implant patients: a clinical study on 1500 consecutive patients. Clin Oral Implant Res. 2008;19(8):823–835. doi: 10.1111/j.1600-0501.2008.01544.x
- Egusa H, Ko N, Shimazu T, et al. Suspected association of an allergic reaction with titanium dental implants: a clinical report. J Prosthet Dent. 2008;100(5):344–347. doi: 10.1016/S0022-3913(08)60233-4
- Chang M, Wennstrom LJ, et al. Implant supported single-tooth replacements compared to contralateral natural teeth. Crown and soft tissue dimensions. Clin Oral Implant Res. 1999;10(3):185–194. doi: 10.1034/j.1600-0501.1999.100301.x
- Jung RE, Sailer I, Hammerle CH, et al. In vitro color changes of soft tissues caused by restorative materials. Int J Periodontics Restorative. 2007;27(3):251–257.
- Park SE, Da Silva JD, Weber HP, et al. Optical phenomenon of peri-implant soft tissue. Part I. Spectrophotometric assessment of natural tooth gingiva and peri-implant mucosa. Clin Oral Implant Res. 2007;18(5):569–574. doi: 10.1111/j.1600-0501.2007.01391.x
- Furhauser R, Florescu D, Benesch T, et al. Evaluation of soft tissue around single-tooth implant crowns: the pink esthetic score. Clin Oral Implant Res. 2005;16(6):639–644. doi: 10.1111/j.1600-0501.2005.01193.x
- Oates TW, West J, Jones J, et al. Long-term changes in soft tissue height on the facial surface of dental implants. Implant Dent. 2002;11(3):272–279. doi: 10.1097/00008505-200207000-00013
- Andreiotelli M, Wenz HJ, Kohal RJ. Are ceramic implants a viable alternative to titanium implants? A systematic literature review. Clin Oral Implant Res. 2009;20(4):32–47. doi: 10.1111/j.1600-0501.2009.01785.x
- Volz U. Dental implant. WO2004096075 A1; 2004.
- Carinci F, Pezzetti F, Volinia S, et al. Zirconium oxide: analysis of MG63 osteoblast-like cell response by means of a microarray technology. Biomaterials. 2004;25(2):215–228. doi: 10.1016/S0142-9612(03)00486-1
- Oliva X OJ, Oliva JD. Full-mouth oral rehabilitation in a titanium allergy patient using zirconium oxide dental implants and zirconium oxide restorations. A case report from an ongoing clinical study. Eur J Esthet Dent. 2010;5(2):190–203.
- Depprich R, Ommerborn M, Zipprich H, et al. Behavior of osteoblastic cells cultured on titanium and structured zirconia surfaces. Head Face Med. 2008;4(1):1–9. doi: 10.1186/1746-160X-4-1
- Lee J, Sieweke JH, Rodriguez NA, et al. Evaluation of nano-technology-modified zirconia oral implants: a study in rabbits. J Clin Periodontol. 2009;36(7):610–617. doi: 10.1111/j.1600-051X.2009.01423.x
- Kim HW, Georgiou G, Knowles JC, et al. Calcium phosphates and glass composite coatings on zirconia for enhanced biocompatibility. Biomaterials. 2004;25(18):4203–4213. doi: 10.1016/j.biomaterials.2003.10.094
- Uchida M, Kim HM, Kokubo T, et al. Apatite-forming ability of a zirconia/alumina nano-composite induced by chemical treatment. J Biomed Mater Res. 2002;60(2):277–282. doi: 10.1002/jbm.10071
- Ye H, Gu Z, Gracias DH. Kinetics of ultraviolet and plasma surface modification of poly(dimethylsiloxane) probed by sum frequency vibrational spectroscopy. Langmuir. 2006;22(4):1863–1868. doi: 10.1021/la052030r
- Watanabe H, Saito K, Kokubun K, et al. Change in surface properties of zirconia and initial attachment of osteoblastlike cells with hydrophilic treatment. Dent Mater J. 2012;31(5):806–814. doi: 10.4012/dmj.2012-069
- Altmann B, Kohal RJ, Steinberg T, et al. Distinct cell functions of osteoblasts on UV-functionalized titanium- and zirconia-based implant materials are modulated by surface topography. Tissue Eng. Part C, Methods. 2013;19(11):850–863. doi: 10.1089/ten.tec.2012.0695
- Noro A, Kaneko M, Murata I, et al. Influence of surface topography and surface physicochemistry on wettability of zirconia (tetragonal zirconia polycrystal). J Biomed Mater Res. Part B, Appl Biomater. 2013;101B(2):355–363. doi: 10.1002/jbm.b.32846
- Schultze-Mosgau S, Schliephake H, Radespiel-Troger M, et al. Osseointegration of endodontic endosseous cones - Zirconium oxide vs titanium. Oral Surg Oral Med Oral Pathol Oral Radiol Endod. 2000;89(1):91–98. doi: 10.1016/S1079-2104(00)80022-0
- Dubruille JH, Viguier E, Le Naour G, et al. Evaluation of combinations of titanium, zirconia, and alumina implants with 2 bone fillers in the dog. Int J Oral Max Impl. 1999;14(2):271–277.
- Gahlert M, Rohling S, Wieland M, et al. Osseointegration of zirconia and titanium dental implants: a histological and histomorphometrical study in the maxilla of pigs. Clin Oral Implant Res. 2009;20(11):1247–1253. doi: 10.1111/j.1600-0501.2009.01734.x
- Hisbergues M, Vendeville S, Vendeville P. Zirconia: Established facts and perspectives for a biomaterial in dental implantology. J Biomed Mater Res. Part B, Appl Biomater. 2009;88B(2):519–529. doi: 10.1002/jbm.b.31147
- De Medeiros RA, Vechiato-Filho AJ, Pellizzer EP, et al. Analysis of the peri-implant soft tissues in contact with zirconia abutments: an evidence-based literature review. J Contemp Dent Pract. 2013;14(3):567–572. doi: 10.5005/jp-journals-10024-1364
- Linkevicius T, Apse P. Influence of abutment material on stability of peri-implant tissues: a systematic review. Int J Oral Max Impl. 2008;23(3):449–456.
- Bidra AS, Rungruanganunt P. Clinical outcomes of implant abutments in the anterior region: a systematic review. J Esthet Restor Dent. 2013;25(3):159–176. doi: 10.1111/jerd.12031
- Sailer I, Philipp A, Zembic A, et al. A systematic review of the performance of ceramic and metal implant abutments supporting fixed implant reconstructions. Clin Oral Implant Res. 2009;20(Suppl 4):4–31. doi: 10.1111/j.1600-0501.2009.01787.x
- Nevins M, Camelo M, Nevins ML, et al. Pilot clinical and histologic evaluations of a two-piece zirconia implant. Int J Periodontics Restorative Dent. 2011;31(2):157–163.
- Aydin C, Yilmaz H, Bankoglu M. A single-tooth, two-piece zirconia implant located in the anterior maxilla: a clinical report. J Prosthet Dent. 2013;109(2):70–74. doi: 10.1016/S0022-3913(13)00027-9
- Albrektsson T, Gottlow J, Meirelles L, et al. Survival of NobelDirect implants: an analysis of 550 consecutively placed implants at 18 different clinical centers. Clin Implant Dent Relat Res. 2007;9(2):65–70. doi: 10.1111/j.1708-8208.2007.00054.x
- Zinelis S, Thomas A, Syres K, et al. Surface characterization of zirconia dental implants. Dent Mater. 2010;26(4):295–305. doi: 10.1016/j.dental.2009.11.079
- Silva NR, Coelho PG, Fernandes CA, et al. Reliability of one-piece ceramic implant. J Biomed Mater Res. Part B, Appl Biomater. 2009;88B(2):419–426. doi: 10.1002/jbm.b.31113
- Borgonovo AE, Censi R, Vavassori V, et al. Evaluation of the success criteria for zirconia dental implants: a four-year clinical and radiological study. Int J Dent. 2013;2013:1–7. doi: 10.1155/2013/463073
- Depprich R, Naujoks C, Ommerborn M, et al. Current findings regarding zirconia implants. Clin Implant Dent Relat Res. 2014;16(1):124–137. doi: 10.1111/j.1708-8208.2012.00454.x
- Koller B, Att W, Strub JR. Survival rates of teeth, implants, and double crown-retained removable dental prostheses: a systematic literature review. Int J Prosthodont. 2011;24(2):109–117.
- Osman RB, Swain MV, Atich M, et al. Ceramic implants (Y-TZP): are they a viable alternative to titanium implants for the support of overdentures? A randomized clinical trial. Clin Oral Implant Res. 2014;25(12):1366–1377. doi: 10.1111/clr.12272
- Osman RB, Ma S, Duncan W, et al. Fractured zirconia implants and related implant designs: scanning electron microscopy analysis. Clin Oral Implant Res. 2013;24(5):592–597. doi: 10.1111/j.1600-0501.2011.02411.x
- Gahlert M, Burtscher D, Grunert I, et al. Failure analysis of fractured dental zirconia implants. Clin Oral Implant Res. 2012;23(3):287–293. doi: 10.1111/j.1600-0501.2011.02206.x
- Shen ZJ, Adolfsson E. Effects of granule density on strength and granule related defects in zirconia. J Eur Ceram Soc. 2012;32:2653–2659. doi: 10.1016/j.jeurceramsoc.2012.02.059
- Zhao J, Zhang K, Liu YH, et al. Effect of multistep processing technique on the formation of micro-defects and residual stresses in zirconia dental restorations. J Prosthodont. 2014;23(3):206–212. doi: 10.1111/jopr.12094
- Kohal RJ, Knauf M, Larsson B, et al. One-piece zirconia oral implants: one-year results from a prospective cohort study. 1. Single tooth replacement. J Clin Periodontol. 2012;39(6):590–597. doi: 10.1111/j.1600-051X.2012.01876.x
- Kohal RJ, Patzelt SB, Butz F, et al. One-piece zirconia oral implants: one-year results from a prospective case series. 2. Three-unit fixed dental prosthesis (FDP) reconstruction. J Clin Periodontol. 2013;40(5):553–562. doi: 10.1111/jcpe.12093
- Osman RB, Elkhadem AH, Ma S, et al. Titanium versus zirconia implants supporting maxillary overdentures: three-dimensional finite element analysis. Int J Oral Maxillofac Implants. 2013;28(5):e198–208. doi: 10.11607/jomi.3019
- Chang CL, Chen CS, Yeung TC, et al. Biomechanical effect of a zirconia dental implant-crown system: a three-dimensional finite element analysis. Int J Oral Maxillofac Implants. 2012;27(4):e49–57.
- Caglar A, Bal BT, Karakoca S, et al. Three-dimensional finite element analysis of titanium and yttrium-stabilized zirconium dioxide abutments and implants. Int J Oral Maxillofac Implants. 2011;26(5):961–969.
- Mobilio N, Stefanoni F, Contiero P, et al. Experimental and numeric stress analysis of titanium and zirconia one-piece dental implants. Int J Oral Maxillofac Implants. 2013;28(3), e135–142. doi: 10.11607/jomi.2335
- Kohal RJ, Papavasiliou G, Kamposiora P, et al. Three-dimensional computerized stress analysis of commercially pure titanium and yttrium-partially stabilized zirconia implants. Int J Prosthodont. 2002;15(2):189–194.
- Zembic A, Philipp AO, Hammerle CH, et al. Eleven-year follow-up of a prospective study of zirconia implant abutments supporting single all-ceramic crowns in anterior and premolar regions. Clin Implant Dent Relat Res. 2015;17(Suppl 2): e417–426. doi: 10.1111/cid.12263
- Brodbeck U. The ZiReal post: a new ceramic implant abutment. J Esthet Restor Dent. 2003;15(1):10–24. doi: 10.1111/j.1708-8240.2003.tb00278.x
- Truninger TC, Stawarczyk B, Leutert CR, et al. Bending moments of zirconia and titanium abutments with internal and external implant-abutment connections after aging and chewing simulation. Clin Oral Implant Res. 2012;23(1):12–18. doi: 10.1111/j.1600-0501.2010.02141.x
- Sailer I, Sailer T, Stawarczyk B, et al. In vitro study of the influence of the type of connection on the fracture load of zirconia abutments with internal and external implant-abutment connections. Int J Oral Maxillofac Implants. 2009;24(5):850–858.
- Yilmaz B, Salaita LG, Seidt JD, et al. Load to failure of different zirconia abutments for an internal hexagon implant. J Prosthet Dent. 2015;114(3):373–377. doi: 10.1016/j.prosdent.2015.03.015
- Foong JK, Judge RB, Palamara JE, et al. Fracture resistance of titanium and zirconia abutments: an in vitro study. J Prosthet Dent. 2013;109(5):304–312. doi: 10.1016/S0022-3913(13)60306-6
- Nothdurft FP, Nonhoff J, Pospiech PR. Pre-fabricated zirconium dioxide implant abutments for single-tooth replacement in the posterior region: success and failure after 3 years of function. Acta Odontol Scand. 2014;72(5):392–400. doi: 10.3109/00016357.2013.863970
- Gehrke P, Alius J, Fischer C, et al. Retentive strength of two-piece CAD/CAM zirconia implant abutments. Clin Implant Dent Relat Res. 2014;16(6):920–925. doi: 10.1111/cid.12060
- Rosentritt M, Rembs A, Behr M, et al. In vitro performance of implant-supported monolithic zirconia crowns: Influence of patient-specific tooth-coloured abutments with titanium adhesive bases. J Dent. 2015;43(7):839–845. doi: 10.1016/j.jdent.2015.04.011
- Maeda Y, Satoh T, Sogo M. In vitro differences of stress concentrations for internal and external hex implant-abutment connections: a short communication. J Oral Rehabil. 2006;33(1):75–78. doi: 10.1111/j.1365-2842.2006.01545.x
- Schmitt CM, Nogueira-Filho G, Tenenbaum HC, et al. Performance of conical abutment (Morse Taper) connection implants: a systematic review. J Biomed Mater Res. Part A. 2014;102(2):552–574. doi: 10.1002/jbm.a.34709
- Passos SP, Linke B, Larjava H, et al. Performance of zirconia abutments for implant-supported single-tooth crowns in esthetic areas: a retrospective study up to 12-year follow-up. Clin Oral Implant Res. 2016;27(1):47–54. doi: 10.1111/clr.12504
- Aboushelib MN, Salameh Z. Zirconia implant abutment fracture: clinical case reports and precautions for use. Int J Prosthodont. 2009;22(6):616–619.
- Nguyen HQ, Tan KB, Nicholls JI. Load fatigue performance of implant-ceramic abutment combinations. Int J Oral Maxillofac Implants. 2009;24(4):636–646.
- Albosefi A, Finkelman M, Zandparsa R. An in vitro comparison of fracture load of zirconia custom abutments with internal connection and different angulations and thickness: part I. J Prosthodont. 2014;23(4):296–301. doi: 10.1111/jopr.12118
- Zandparsa R, Albosefi A. An in vitro comparison of fracture load of zirconia custom abutments with internal connection and different angulations and thicknesses: Part II. J Prosthodont. 2016;25(2):151–155. doi: 10.1111/jopr.12292
- Ferrari M, Vichi A, Zarone F. Zirconia abutments and restorations: from laboratory to clinical investigations. Dent Mater. 2015;31(3), e63–76. doi: 10.1016/j.dental.2014.11.015
- Thulasidas S, Givan DA, Lemons JE, et al. Influence of implant angulation on the fracture resistance of zirconia abutments. J Prosthodont. 2015;24(2):127–135. doi: 10.1111/jopr.12182
- Alqahtani F, Flinton R. Postfatigue fracture resistance of modified prefabricated zirconia implant abutments. J Prosthet Dent. 2014;112(2):299–305. doi: 10.1016/j.prosdent.2013.08.023
- Hjerppe J, Lassila LV, Rakkolainen T, et al. Load-bearing capacity of custom-made versus prefabricated commercially available zirconia abutments. Int J Oral Maxillofac Implants. 2011;26(1):132–138.
- Gibbs CH, Anusavice KJ, Young HM, et al. Maximum clenching force of patients with moderate loss of posterior tooth support: a pilot study. J Prosthet Dent. 2002;88(5):498–502. doi: 10.1067/mpr.2002.129062
- Park JI, Lee Y, Lee JH, et al. Comparison of fracture resistance and fit accuracy of customized zirconia abutments with prefabricated zirconia abutments in internal hexagonal implants. Clin Implant Dent Relat Res. 2013;15(5):769–778.
- Baixe S, Fauxpoint G, Arntz Y, et al. Microgap between zirconia abutments and titanium implants. Int J Oral Maxillofac Implants. 2010;25(3):455–460.
- Abdelhamed MI, Galley JD, Bailey MT, et al. A comparison of zirconia and titanium abutments for microleakage. Clin Implant Dent Relat Res. 2015;17(Suppl. 2):e643–651. doi: 10.1111/cid.12301
- Alikhasi M, Monzavi A, Bassir SH, et al. A comparison of precision of fit, rotational freedom, and torque loss with copy-milled zirconia and prefabricated titanium abutments. Int J Oral Maxillofac Implants. 2013;28(4):996–1002. doi: 10.11607/jomi.2937
- Smith NA, Turkyilmaz I. Evaluation of the sealing capability of implants to titanium and zirconia abutments against Porphyromonas gingivalis, Prevotella intermedia, and Fusobacterium nucleatum under different screw torque values. J Prosthet Dent. 2014;112(3):561–567. doi: 10.1016/j.prosdent.2013.11.010
- Sui X, Wei H, Wang D, et al. Experimental research on the relationship between fit accuracy and fracture resistance of zirconia abutments. J Dent. 2014;42(10):1353–1359. doi: 10.1016/j.jdent.2014.02.008
- Carrillo de Albornoz A, Vignoletti F, Ferrantino L, et al. A randomized trial on the aesthetic outcomes of implant-supported restorations with zirconia or titanium abutments. J Clin Periodontol. 2014;41(12):1161–1169. doi: 10.1111/jcpe.12312
- Yang J, Wang K, Liu G, et al. Fracture resistance of inter-joined zirconia abutment of dental implant system with injection molding technique. Clin Oral Implant Res. 2013;24(11):1247–1250.
- Marchack BW, Sato S, Marchack CB, et al. Complete and partial contour zirconia designs for crowns and fixed dental prostheses: a clinical report. J Prosthet Dent. 2011;106(3):145–152. doi: 10.1016/S0022-3913(11)60112-1
- Cales B. Colored zirconia ceramics for dental applications. Bioceramics Conference. 1998;11(11):2–4.
- Suttor D, Hauptmann H, Schnagl R, et al. Coloring ceramics by way of ionic or complex-containing solutions. US6709694 B1; 2004 Mar 23.
- Lin YZ, Yi YF, Dong LM, et al. Research on the coloration of dental zirconia. Rare Met Mater Eng. 2009;38(Suppl. 2):859–862.
- Wen N, Yi YF, Deng B, et al. Measurement of chromaticity of five hued zirconia. J South Med Univ. 2009;29(5):3.
- Filser F. Direct ceramic machining of ceramic dental restorations [doctor's thesis]. Zurich Swiss Federal Institute of Technology Zurich; 2001.
- Aboushelib MN, Kleverlaan CJ, Feilzer AJ. Effect of zirconia type on its bond strength with different veneer ceramics. J Prosthodont. 2008;17(5):401–408. doi: 10.1111/j.1532-849X.2008.00306.x
- Shah K, Holloway JA, Denry IL. Effect of coloring with various metal oxides on the microstructure, color, and flexural strength of 3Y-TZP. J Biomed Mater Res Part B – Appl Biomater. 2008;87B(2):329–337. doi: 10.1002/jbm.b.31107
- Pittayachawan P, McDonald A, Petrie A, et al. The biaxial flexural strength and fatigue property of Lava™ Y-TZP dental ceramic. Dent Mater. 2007;23(8):1018–1029. doi: 10.1016/j.dental.2006.09.003
- Hjerppe J, Narhi T, Froberg K, et al. Effect of shading the zirconia framework on biaxial strength and surface microhardness. Acta Odontol Scand. 2008;66(5):262–267. doi: 10.1080/00016350802247123
- Heffernan MJ, Aquilino SA, Diaz-Arnold AM, et al. Relative translucency of six all-ceramic systems. Part I: core materials. J Prosthet Dent. 2002;88(1):4–9. doi: 10.1067/mpr.2002.126794
- Chen YM, Smales RJ, Yip KH, et al. Translucency and biaxial flexural strength of four ceramic core materials. Dent Mater. 2008;24(11):1506–1511. doi: 10.1016/j.dental.2008.03.010
- Zhang Y. Making yttria-stabilized tetragonal zirconia translucent. Dent Mater. 2014;30(10):1195–1203. doi: 10.1016/j.dental.2014.08.375
- Matsuzaki F, Sekine H, Honma S, et al. Translucency and flexural strength of monolithic translucent zirconia and porcelain-layered zirconia. Dent Mater J. 2015;34(6):910–917. doi: 10.4012/dmj.2015-107
- Klimke J, Trunec M, Krell A. Transparent tetragonal yttria-stabilized zirconia ceramics: influence of scattering caused by birefringence. J Am Chem Soc. 2011;94(6):1850–1858.
- Spyropoulou PE, Giroux EC, Razzoog ME, et al. Translucency of shaded zirconia core material. J Prosthet Dent. 2011;105(5):304–307. doi: 10.1016/S0022-3913(11)60056-5
- Oh WS, DeLong R, Anusavice KJ. Factors affecting enamel and ceramic wear: a literature review. J Prosthet Dent. 2002;87(4):451–459. doi: 10.1067/mpr.2002.123851
- Beuer F, Stimmelmayr M, Gueth JF, et al. In vitro performance of full-contour zirconia single crowns. Dent Mater. 2012;28(4):449–456. doi: 10.1016/j.dental.2011.11.024
- Jung YS, Lee JW, Choi YJ, et al. A study on the in-vitro wear of the natural tooth structure by opposing zirconia or dental porcelain. J Adv Prosthodont. 2010;2(3):111–115. doi: 10.4047/jap.2010.2.3.111
- Wang L, Liu Y, Si W, et al. Friction and wear behaviors of dental ceramics against natural tooth enamel. J Eur Ceram Soc. 2012;32(11):2599–2606. doi: 10.1016/j.jeurceramsoc.2012.03.021
- Mitov G, Heintze SD, Walz S, et al. Wear behavior of dental Y-TZP ceramic against natural enamel after different finishing procedures. Dent Mater. 2012;28(8):909–918. doi: 10.1016/j.dental.2012.04.010
- Sabrah AH, Cook NB, Luangruangrong P, et al. Full-contour Y-TZP ceramic surface roughness effect on synthetic hydroxyapatite wear. Dent Mater. 2013;29(6):666–673. doi: 10.1016/j.dental.2013.03.008
- Passos SP, Torrealba Y, Major P, et al. In vitro wear behavior of zirconia opposing enamel: a systematic review. J Prosthodont. 2014;23(8):593–601. doi: 10.1111/jopr.12167
- Amer R, Kurklu D, Kateeb E, et al. Three-body wear potential of dental yttrium-stabilized zirconia ceramic after grinding, polishing, and glazing treatments. J Prosthet Dent. 2014;112(5):1151–1155. doi: 10.1016/j.prosdent.2013.12.021
- Bai Y, Zhao J, Si W, et al. Two-body wear performance of dental colored zirconia after different surface treatments. J Prosthet Dent. 2016;116(4):584–590. doi: 10.1016/j.prosdent.2016.02.006
- Mundhe K, Jain V, Pruthi G, et al. Clinical study to evaluate the wear of natural enamel antagonist to zirconia and metal ceramic crowns. J Prosthet Dent. 2015;114(3):358–363. doi: 10.1016/j.prosdent.2015.03.001
- Liu YH, Wang Y, Wang DZ, et al. Self-glzed zirconia reducing the wear to tooth enamel. J Eur Ceram Soc. 2016;36(12):2889–2894. doi: 10.1016/j.jeurceramsoc.2015.11.029
- Lughi V, Sergo V. Low temperature degradation -aging- of zirconia: a critical review of the relevant aspects in dentistry. Dent Mater. 2010;26(8):807–820. doi: 10.1016/j.dental.2010.04.006
- Papanagiotou HP, Morgano SM, Giordano RA, et al. In vitro evaluation of low-temperature aging effects and finishing procedures on the flexural strength and structural stability of Y-TZP dental ceramics. J Prosthet Dent. 2006;96(3):154–164. doi: 10.1016/j.prosdent.2006.08.004
- Flinn BD, deGroot DA, Mancl LA, et al. Accelerated aging characteristics of three yttria-stabilized tetragonal zirconia polycrystalline dental material. J Prosthet Dent. 2012;108(4):223–230. doi: 10.1016/S0022-3913(12)60166-8
- Chen SY, Lu HY. Low-temperature aging map for 3 Mol-Percent Y2O3-ZrO2. J Mater Sci. 1989;24(2):453–456. doi: 10.1007/BF01107426
- Munoz-Saldana J, Balmori-Ramirez H, Jaramillo-Vigueras D, et al. Mechanical properties and low-temperature aging of tetragonal zirconia polycrystals processed by hot isostatic pressing. J Mater Res. 2003;18(10):2415–2426. doi: 10.1557/JMR.2003.0337
- Li JF, Watanabe R. Phase transformation in Y2O3-partially-stabilized ZrO2 polycrystals of various grain sizes during low-temperature aging in water. J Am Ceram Soc. 1998;81(10):2687–2691. doi: 10.1111/j.1151-2916.1998.tb02677.x
- Kosmac T, Kocjan A. Ageing of dental zirconia ceramics. J Eu Ceram Soc. 2012;32(11):2613–2622. doi: 10.1016/j.jeurceramsoc.2012.02.024
- Hallmann L, Mehl A, Ulmer P, et al. The influence of grain size on low-temperature degradation of dental zirconia. J Biomed Mater Res Part B-Appl Biomater. 2012;100B(2):447–456. doi: 10.1002/jbm.b.31969
- Samodurova A, Kocjan A, Swain MV, et al. The combined effect of alumina and silica co-doping on the ageing resistance of 3Y-TZP bioceramics. Acta Biomater. 2015;11:477–487. doi: 10.1016/j.actbio.2014.09.009
- Ardlin BI. Transformation-toughened zirconia for dental inlays, crowns and bridges: chemical stability and effect of low-temperature aging on flexural strength and surface structure. Dent Mater. 2002;18(8):590–595. doi: 10.1016/S0109-5641(01)00095-1
- Nakamura K, Harada A, Kanno T, et al. The influence of low-temperature degradation and cyclic loading on the fracture resistance of monolithic zirconia molar crowns. J Mech Behav Biomed Mater. 2015;47:49–56. doi: 10.1016/j.jmbbm.2015.03.007
- de Kok P, Kleverlaan CJ, de Jager N, et al. Mechanical performance of implant-supported posterior crowns. J Prosthet Dent. 2015;114(1):59–66. doi: 10.1016/j.prosdent.2014.10.015
- Nakamura K, Harada A, Inagaki R, et al. Fracture resistance of monolithic zirconia molar crowns with reduced thickness. Acta Odontol Scand. 2015;73(8):602–608. doi: 10.3109/00016357.2015.1007479
- Lan TH, Liu PH, Chou MM, et al. Fracture resistance of monolithic zirconia crowns with different occlusal thicknesses in implant prostheses. J Prosthet Dent. 2016;115(1):76–83. doi: 10.1016/j.prosdent.2015.06.021
- Hammerle CHF, Wagner D, Bragger U, et al. Threshold Of Tactile Sensitivity Perceived with Dental Endosseous Implants And Natural Teeth. Clin Oral Implant Res. 1995;6(2):83–90. doi: 10.1034/j.1600-0501.1995.060203.x
- Karl M, Graef F, Wichmann M, et al. Passivity of fit of CAD/CAM and copy-milled frameworks, veneered frameworks, and anatomically contoured, zirconia ceramic, implant-supported fixed prostheses. J Prosthet Dent. 2012;107(4):232–238. doi: 10.1016/S0022-3913(12)60067-5