ABSTRACT
Phosphates particularly zinc phosphates is a family of conventional dental cements that was developed a century ago and has been in use until today despite the fact that a number of new resin composite cements have been introduced in the market through the years. A close inspection of the in vivo aged samples provides insides to the margins and to the micro-leakage thus would sharpen the understanding and inspire the future development of the cements and cementation interfaces between the dental restorations and the natural tooth or implant abutment. This study highlights the cementation interface and its structural change during in vivo aging. Besides the focused studies on phosphate cements, limited investigation has also been performed on resin composite cements as references.
Introduction
Dental restorations are luted on the natural teeth or implant abutments to ensure decent retention and to seal the margin from infiltration of saliva and intraoral fluids. Many types of luting cement materials have been used by the date with different properties that include zinc phosphate, zinc polycarboxylate, glass ionomer, resin ionomer, composites and adhesive resin [Citation1]. The newly developed resin composite cements have usually better adhesion behaviours than the classical phosphate cements. They are less soluble and are faster to harden, thus decreases the treatment time. One drawback of strong concern on resin composite cements is the fact that the excess cement squeezed inside the gingiva gap is more difficult to remove and may cause pulp irritation [Citation1,Citation2].
Even though the adhesion provided by calcium or zinc phosphate cements is known to be lower than that provided by the newer resin-based cements, there are evidence indicating that the phosphate cement luted restorations stayed in use without an obvious problem. Zinc phosphate cement anchors the restorations purely mechanically. In general, it has good compressive strength whereas low tensile strength. It undergoes dissolution/chemical changes in the oral environment [Citation3]. However, despite or owning to these problems zinc phosphate appeared a reliable cement with success rate of incredible 74% after 15 years with conventional fixed partial dentures [Citation4] and restorations with over 20 and even up to 45 years working time [Citation2,Citation5].
In practice, a cementation gap is always present between the restorations and tooth/implant abutment and its width is determined by the design and the type of cement used, as well the preparation method and the skills of the dentist. This cementation gap close to margin end, known as marginal gap, effects the bonding strength, the dissolution of the cement and the marginal leakage [Citation6]. Different luting cements affect the sealing ability and the possible obstructions of dental fluid penetration into the marginal leakage as well as the resistance to bite stresses [Citation7,Citation8].
McLean and von Fraunhofer suggested that the maximum tolerable cementation gap should be 120 µm but this figure was questioned by Kydd et al. who showed that even the marginal gaps up to a width of 244 µm in gold crown zinc phosphate lasted longer than 20 years [Citation5,Citation9]. The width of the cementation gap will affect the micro-leakage that is depending on the cementation layer thickness. When the thickness of the cementation layer was 25–75 µm there was not seen any changes in the micro-leakage whereas when this thickness was greater than 150 µm the micro-leakage became significantly higher [Citation5,Citation10].
Even though the zinc phosphate cement luted restorations have shown to last long time, it has been reported that the marginal leakage in restorations may be responsible for different failures of the restorations. One such failures is the bacterial colonisation that in the minor case can result in marginal discolouration and in severe cases may lead to pulpal pathosis, periodontal disease, and microbiological ingress and secondary caries [Citation11–14].
Not only has the marginal gap caused problem to the restorations but also the bacterial adhesion on the excess cement. The excess cement squeezed out from the marginal end generates a rough surface in the gingiva gap that favours the bacterial adhesion and can behave as the origin to peri-implant mucosities and peri-implantitis [Citation15,Citation16]. It has been shown that surface finish and proper removal of the excess cement will minimise the bacterial adhesion positively [Citation17].
A close inspection of the in vivo aged samples would provide insides to the margins and to the micro-leakage thus sharpen the understanding and inspire the future development of the cements and cementation interface. The aim of this work was to study the cementation interfaces and the structural change of the cement during in vivo aging. All the samples were extracted teeth and implants with cemented restorations remaining on them. Although the designed focus was on phosphate cements, limited amount of samples with resin composite cements collected were also investigated and discussed as the references.
Materials and methods
Collection of the samples
Dental restorations after a varying time of in vivo service were removed from the patients and collected. The detailed list of the collected samples is presented in . It is noted that the restoration b and f were fractured during removal and e was removed because of an infection. The restoration a, c, and d were in good conditions but no patient’s situation and other information were recorded. However, it is noted that the loss of coherence between the restoration and natural tooth or implant abutment was not the reason.
Table 1. The list of collected samples of in vivo aged restorations.
The collected restorations were in use for different in vivo times, varying from 6 to 25 years. The materials used for making restorations and abutments changed between the samples and also the type of phosphate cements and the way of using the phosphate cements. Both calcium and zinc phosphates and resin composite cements containing alumina and silica as fillers are included in the samples. The phosphate cements were used both in luting and in cavity lining.
The collected restorations were first inspected with a light microscope (S8APO, Leica Microsystems, Germany) followed by the characterisation using scanning electron microscope (SEM). The as-received restorations were washed with ethanol in ultra-sonic bath prior to SEM where the outside surface and interfaces between the restoration/cement/abutment were investigated. After the characterisation of the outside surface, the restorations were cut horizontally or/and vertically with low-speed saw and investigated with SEM without polishing and after polishing to 1 µm surface finish. More detailed investigation of the interfaces was conducted after the cross-sections polishing by an Argon-ion beam polisher (CP-09010, JEOL, Japan) with the acceleration voltage of 5 kV for 15 h. An field emission SEM (JSM-7000F, Jeol, Japan) equipped with an energy dispersive spectroscopy (EDS) detector (INCAx-sight, Oxford Instruments, UK) and a tabletop scanning electron microscope (TM3000, JEOL) were used for SEM investigations of the clean surfaces without any additional coating.
XRD and Raman spectra were used for analysing the phase assembly in in vivo aged cements that were compared to freshly made zinc phosphate cement prepared according to the instruction from the producer (Harward cement, Hoppegarten, Germany). After the microstructure characterisation the cements were carefully removed and analysed using XRD with Cuα1 radiation (X´Pert Pro, PANalytical, Netherlands) and Raman spectra normalised to the peak at 110 cm−1 (785 nm red laser, LabRAM HR, HORIBA, USA).
Results
Pores and porosity
Pores or voids with different sizes generated during the mixing and setting of the cement, as shown in and 2, were observed both in freshly made and aged cements. They can be classified into two types, of which one is spherical-shaped with the formation of faceted crystals inside whereas the other with irregular shapes having smooth inner surfaces without crystals inside. The formation of these pores can be originated to the inclusion of the air bubbles during the mixing or evaporation of the water during the setting. The latter most likely leads to the formation of micro-pores up to 10 µm in diameter while the former produces pores up to 172 µm. The spherical pores show crystal formation independent of the size. It is noted that the pores on the surface of phosphate cements exposed to gingiva or oral liquids are covered with body tissue, indicating a good biocompatibility of the cements.
Crystallisation and growth of hopeite
The uneven reaction during setting gives possibility for crystal growth in the pores as seen in for freshly made zinc phosphate cement as an example. Similar crystals are seen in the in vivo aged zinc phosphate samples, as seen in (a, b), and it is obvious that the crystals have grown in size during the aging. The XRD analysis confirms that the crystals can be attributed to the crystalline form of zinc phosphate Zn3(PO4)2·4H2O (‘hopeite’) [Citation18,Citation19] together with the typically unreacted ZnO and MgO in an amorphous matrix, (a). The XRD on the freshly made zinc phosphate cement did not show peaks for hopeite even though the visual investigation reveals the crystals indicating a larger formation and growth of the hopeite during the aging.
Figure 2. SEM images revealing the formation of large crystals inside the pores in a zinc phosphate cement after 6 years in vivo aging, sample a (a), the pores on the surface of the cement after 25 years in vivo aging, sample b, exposed to oral environment that are covered with body tissues appeared dark colour in the BS mode (b), and the crystal growth in a calcium phosphate cement after 25 years in vivo aging in contacting with gingiva, sample b (d).
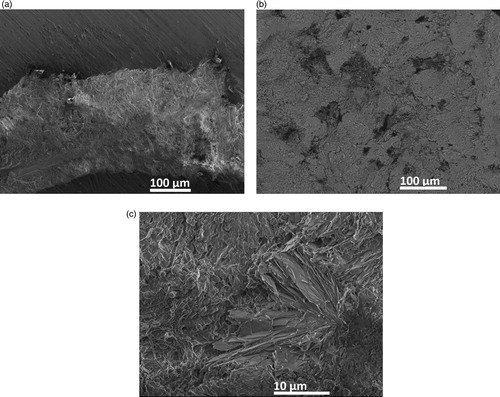
Figure 3. XRD patterns taken on the horizontally cut surface of a zinc phosphate cement in vivo aged for 6 years (sample a) (a), and on two calcium phosphate cement samples in vivo aged for 25 years (sample b) and 21 years (sample d), respectively (b).
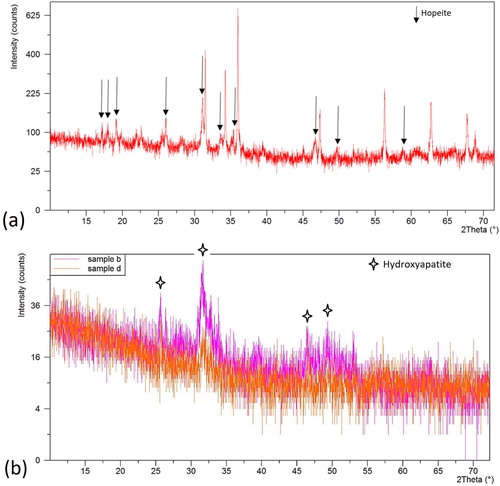
The crystals are also observed in the calcium phosphate cement in contact with gingiva as shown in (c), the composition of these crystals could not be confirmed due to the difficulties to take the XRD but they are presumably hydroxyapatite. However, similar cement in the oral environment did not show such crystals in SEM but the XRD in (b) shows increased intensity of the peaks for hydroxyapatite in an otherwise amorphous cement, confirming the good biocompatibility as already seen in the SEM. It is worth to note that no significant crystalline peaks attributed to solid filler particles (alumina, silica, fluorine) were detected after in vivo aging indicating the retention of the calcium phosphate cement in the oral environment.
Cracks and changes in zinc oxide content
Cracks were observed in all in vivo aged phosphate cement samples in the cement matrix along the interfaces either between cement and tooth/abutment or between cement and restoration. The location of the detached interface (gap) depended on the cement material used. The most severe cracking in the cement is located at the margin end, as demonstrated in , where the SEM image was taken on a vertically cross-sectioned sample a is presented. Close to the margin the cement is severely cracked and even lost. The cracks formed in the cement expose the cement to the oral environment thus affect the chemical composition of the cement. The zinc phosphate cement is known to decompose/dissolve [Citation10,Citation20,Citation21] in the oral environment and this is also the case here. The EDS analysis confirms the chemical changes of the cement. The EDS results for the sample with 9 years in vivo close to the margin end of the restoration are shown in . The change of composition is confirmed also with Raman, shown in after 25 years in vivo aging in comparison with the freshly made cement. The Raman shift connected to ZnO (101, 388 and 437 cm−1) are decreased during aging when compared to a freshly made cement. The typical microstructure of zinc phosphate remains deeper in the marginal gap, as seen in the insets in . The microstructure after the ZnO dissolution becomes more porous and fragile deteriorating the mechanical properties of the cement It is noted that the chemical composition of the phosphate cement remains intact and only zinc oxide particles are disappeared. The dissolution starts where there is an access to the oral environment. So, the cement starts to dissolve in the interfacial gaps between the cement and restoration or between the cement and tooth/abutment as well close to the cracks. The dissolution process ought to be time depended [Citation20,Citation21]; the longer time the restoration is in use the larger dissolution of zinc oxide and deterioration of the mechanical properties of the cement but it is not seen clearly because there are even other factors affecting the reliability of the restoration such as mechanical erosion and the gap width.
Figure 4. SEM images taken on a vertically cross-sectioned zinc phosphate cement sample in vivo aged for 6 years (sample a) revealing cracking and loss of cement at the margin end. Insets are images taken with higher magnification at the spots indicated by the circles.
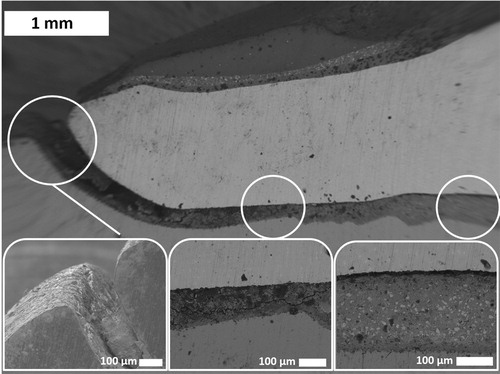
Figure 5. EDS analysis at the margin end of a zinc phosphate cement samples in vivo aged for 9 years (sample c), indicating the change of zinc and carbon content. The inserts show the EDX results for indicated area on the cement.
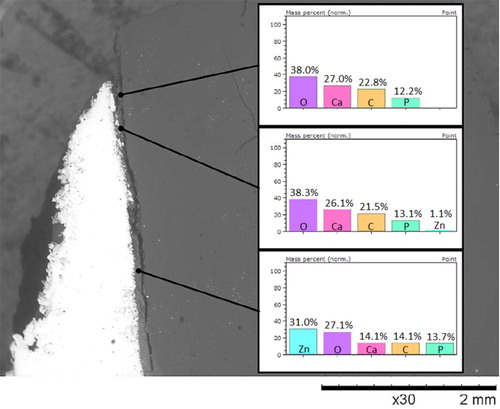
Figure 6. Normalised Raman shifts recorded in freshly made zinc phosphate cement and the one after 25 years in vivo aging, sample b.
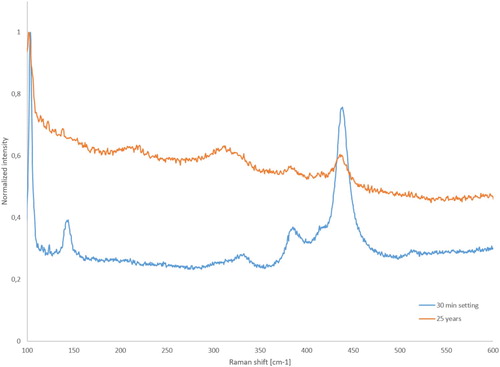
The calcium phosphate cement shows similar retention behaviour close to the marginal end of the restoration with cracks inside the cement. EDS analysis revealed also a chemical change where the filler particles made of alumina, fluoride and silica are hard to detect close to the margin end. They can be detected again at similar distances from the margin as Zn for zinc phosphate cement shown in .
Debonding and the formation of interfacial gaps
The observed crack formation along the interfaces either between cement and tooth/abutment or between cement and restoration in all in vivo aged restorations led to the interfacial detachment, i.e. the formation of interfacial gaps. The location of the interfacial gap varies depending on the cement used. The zinc phosphate cement is detached often inside the cement or on the interface between cement and tooth, see (a), while the interface between cement and metal restoration remained intact. It must be noted that the interfaces between metal and zinc phosphate are generally good and the cracking of the cement occurs mainly inside the cement matrix, not on the interfaces as shown in where the Titanium was used as abutment and CoCr as restoration.
Figure 7. SEM images taken on the vertically cross-sectioned samples revealing the interfacial debonding taking place on the interface between the zinc phosphate and tooth observed in sample c (a), respectively, on the interface between the resin cement and crown that is turn to the interface between zinc phosphate cement and tooth when zinc phosphate cement is used for lining (upper right corner), sample e (b).
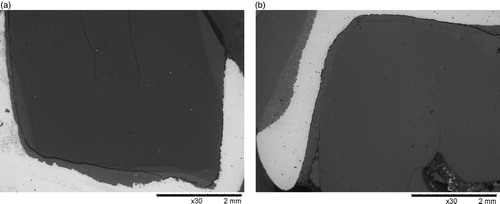
Calcium phosphate cement detached on the interface between cement and tooth and also in some cases on the interface between cement and restoration. Sample e is a single crown luted by resin composite cement with alumina and silica as fillers and zinc phosphate cement was used at the top of the tooth for lining. The resin composite cement detached on the interface between cement and crown. When the interfacial gap got closer to zinc phosphate lining it moved to the interface between cement and tooth as seen in (b). It is also noted that the interface debonding is a common feature that is observed in all the samples investigated in the current study.
Carbon contamination
The SEM image shown in revealed that the marginal gap was filled by biological masses in sample a after 6 years in vivo aging. As the cementation gap of the restoration at the margin end was exposed to oral environment once cracked, the infiltration of saliva/oral fluid may not only affect the overall chemical composition and mechanical properties of the cements but also enable the deposition/accumulation of infiltrated biological matter that can be recognised as carbon contamination. This is seen in all the samples as demonstrated in , and , where the backscatter SEM images reveal carbon contamination existing even inside the pores beside along the interfacial gaps of the cement at the margin end of the restorations. The carbon contamination was not only observed in the marginal end of the restorations but also on the top of the tooth, as is seen in where the zinc phosphate lining at the very top of the tooth is contaminated with carbon. The detected carbon signalises the infiltration of carbon-containing biological masses to the top through the interfacial gaps formed between resin composite cement and the restoration. Furthermore, it must be mentioned that the zinc phosphate lining appeared very brittle, inside which many cracks formed during cutting of the sample if not already formed during the in vivo service.
Figure 8. An SEM image revealing the filling of the marginal gap by biological masses in sample a after 6 years in vivo aging when a zinc phosphate cement was used.
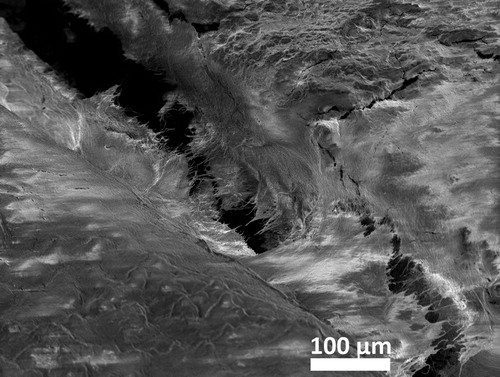
Figure 9. The SEM/EDS mapping images taken on the sample e after 6 years in vivo aging when resin composite cement and zinc phosphate cement were used for lining and for luting, respectively. The strong element contrast of aluminium and zinc detected in different regions confirmed the use of a zinc phosphate cement and a resin composite cement containing alumina and silica fillers. The detected carbon content inside the zinc phosphate cement indicated the contamination of the zinc phosphate cement by saliva/ oral fluid via the formed interfacial gap.
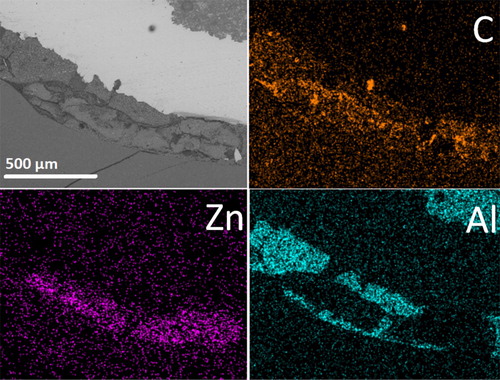
Excess cements
At the margin end of the restorations luted by phosphate cement cracks were observed but no excess cement outside the marginal end in any investigated samples. This was not the case when resin composite was used as luting cement. Even though the resin composite containing alumina and silica fillers did not show any chemical change during in vivo aging, excess of resin composite cement squeezed outside the marginal end was observed, as seen in . It is clear that this excess resin composite cement in contact with gingiva will give rise to bacterial growth near the margin and may cause severe problems to patients in the form of infections and indeed this was the case with the samples e that was extracted from the patient because of an infection.
Discussions
Phosphate cements are defined as chemically bonded ceramics that are a family of materials formed and consolidated by chemical reaction instead of thermal diffusion. As these room-temperature-setting materials are easy to produce, they were developed over a century ago. From the results presented above, it is clear that the phosphate cements appear brittle thus are sensitive to cracking unlike resin composite cement that does not show major cracking. The problems encountered at the marginal end are related to the cementation material used and the mechanical stresses applied. Øilo et al. [Citation22] showed that the chewing causes tensile hoop stresses on the tip of the crowns that would affect the cracking and the debonding of the cement. Interfacial debonding as a common feature was observed in all three types of cements. According to the literature the zinc phosphate debonds mainly on the interface between cement and tooth [Citation5,Citation23]. This early finding was confirmed in the current study, which revealed that the zinc phosphate preferred the bonding with metallic restorations beyond the bonding to the natural teeth. The different metals used for abutments and crowns made it difficult to draw final conclusions on the calcium phosphate and resin composite cements. There is a small uncertainty in the investigation concerning the interface debonding caused by the drying of the natural teeth during the preparation work. The drying might increase the stresses in the interface and increase the cracking/debonding probability. After the removal from the oral environment, there is no longer a carbon source present and thus the infiltration of the carbon to the top of the tooth is a distinct evidence proving that the cracking and the debonding is an existing problem existing before sample preparation. The cracking and debonding generate problems that cause the micro-leakage of intraoral fluids into the marginal gaps and the possible infiltration/deposition of biological masses and even bacterial colonisation on the surface close to the margin end.
No restorations luted by zinc phosphate were extracted due to the infections in this study. It indicates that the observed micro-leakage is not harmful to the reliability of the phosphate cement luting as it has been already stated in several earlier studies [Citation5,Citation11,Citation24–26]. But if the interfacial gaps are broad enough (>50 µm) [Citation27] caution should be taken that such broader gaps might make possible a bacterial ingress and secondary caries not only close to gingiva but even inside the restorations. The major cracking in phosphate cements luted restorations occurs at the margin end where the cement is exposed to oral fluids and often withstands micro-movements under the biting stresses. Both mechanisms will increase the cracking/dissolution of the cement and in that way increase the micro-leakage. The micro-leakage is also depended on the thickness of the cementation layer. According to the literature, the layer thickness above 150 µm will increase the dissolution speed of the zinc oxide and thus micro-leakage [Citation10]. It is worth to emphasise that in this study the thickness of the cementation layer larger than 150 µm was observed, but the possible formation of secondary caries was not the reason for the removal of the restorations luted by zinc phosphate cement.
The micro-leakage changes the chemical composition of zinc phosphate cement. Nevertheless, the dissolution did not prohibited that the zinc phosphate luted restorations were used over 20 years without bacterial colonisation and obvious increased inflammatory failures in comparison to the resin composite cement luted restorations [Citation5,Citation28]. Another issue is the observed missing of alumina and silica fillers at the margin end in calcium phosphate cement. It is hardly possible for alumina and silica particles to dissolve in the oral environment, so, the observed missing of alumina and silica fillers most likely ought to be connected to either the harsh sample preparation with the low-speed saw causing pullout during the process or to the poorer mechanical properties of the calcium phosphate cement. The reduction of the chemical retention of the phosphate cements by in vivo aging and the physical movement of the cement during biting could be other possible reasons responsible for the pullout of the filler particles.
The long-lasting time of restorations luted by zinc phosphate cement should also be connected to the easier removal of the excess cement squeezed out the marginal end. The cleaner surface towards gingiva reduces the possibility of bacterial adhesion at the marginal end opposite to the resin composite cement luted restorations. The removal of the excess resin composite cement is difficult because it is not brittle and very hard to detect with the radiographic method [Citation28–30]. In this study, excess cement facing gingiva or close to gingiva was observed in two resin composite cement luted restoration samples. The excess cement outside the marginal end will give a growing platform for bacterial adhesion that may be quoted as the origin of secondary caries that would increase the risk of infections. This is evidenced by the fact that one of the restorations luted by resin composite cement in this study was removed because of the inflammation. The excess cement may also cause the peri-implant disease as discussed in review of Valente et al [Citation31]. Linkevicius et al. showed that 85% of 72 implants developed peri-implant disease when there was found excess cement and that all the patients with excess cement and with earlier problems with peridontitis developed peri-implantitis [Citation32].
With proper phosphate cement preparation, the content of pores with hopeite caused by air or water evaporation during the mixing will decrease, thus, the mechanical strength of the cement will increase [Citation33–35]. This will inhibit the cracking of the cement. In order to decrease the risks caused by excess cement, marginal leakage and debonding on the margins a better marginal fit should be achieved together with the better mixing and working habits with all types of cements.
Conclusions
Within the limitation of this study, it was revealed that pores presented in all samples, with apparently higher concentration in zinc phosphate cement. The formation of platelet tertiary zinc phosphate (‘hopeite’) crystals was found confined inside these pores, in which the growth of the crystals continued during in vivo aging. The crack formation as a common feature was observed in all phosphate cements, but not in resin composite cements. Severe cracking and loss of cement were observed at the marginal end of the cementation gap of all the samples, which may indicate the risk of marginal leakage after long-term aging. The unreacted zinc oxide particles initially imbedded in the zinc phosphate cement matrix dissolved on the surface of the interfacial debonding gaps close to the margin end. Interfacial debonding was observed at one of the two interfaces between crown and cement respectively between cement and tooth/abutment, i.e. at the cement-tooth interface when zinc phosphate cement was used, and at both the cement-crown and cement abutment interface when calcium phosphate and resin composite cements were used. The detected carbon infiltrated along the interfacial debonding gaps and cracks from the marginal surface up to the restorations top may indicate the penetration of protein-containing saliva or possibly even bacteria. The excess calcium phosphate cement piece stuck on the outside of porcelain surface of the crown sample b was stable and showed significant retention after a 25 years period in the oral cavity. When resin composite cements were used, excess cements were observed in gingiva gap outside the marginal end of the cementation gap, which rationalised the difficulty commonly faced in daily practice in cleaning up the resin composite cement leaked into the gingiva gap. Comparably, the brittleness of zinc phosphate cement appears an advantage as it enables the easy cleaning of the excess cements squeezed out the marginal end thus reduces the risk of bacterial colonisation and the formation of secondary caries.
Acknowledgements
We thank Dr. Per Tidehag for valuable discussions.
Disclosure statement
No potential conflict of interest was reported by the author(s).
References
- Rosenstiel SF, Land MF, Crispin BJ. Dental luting agents: a review of the current literature. J Prosthet Dent. 1998;80(3):280–301. doi: 10.1016/s0022-3913(98)70128-3
- Ladha K, Verma M. Conventional and contemporary luting cements: an overview. J Indian Prosthodont Soc. 2010;10(2):79–88. doi: 10.1007/s13191-010-0022-0
- Leloup JM, Serraj S, Pauvert B, et al. Chemical characterization of in vivo aged zinc polycarboxylate dental cements. J Mater Sci Mater Med. 1998;9(9):493–496. doi: 10.1023/A:1008879603360
- Creugers NH, Käyser AF, van ‘t Hof MA. A meta-analysis of durability data on conventional fixed bridges. Community Dent Oral Epidemiol. 1994;22(6):448–452. doi: 10.1111/j.1600-0528.1994.tb00795.x
- Kydd WL, Nicholls JI, Harrington G, et al. Marginal leakage of cast gold crowns luted with zinc phosphate cement: an in vivo study. J Prosthet Dent. 1996;75(1):9–13. doi: 10.1016/s0022-3913(96)90411-4
- Blatz MB, Oppes S, Chiche G, et al. Influence of cementation technique on fracture strength and leakage of alumina all-ceramic crowns after cyclic loading. Quintessence Int. 2008;39(1):23–32.
- Yüksel E, Zaimoğlu A. Influence of marginal fit and cement types on microleakage of all-ceramic crown systems. Braz Oral Res. 2011;25(3):261–266. doi: 10.1590/S1806-83242011000300012
- Baldissara P, Comin G, Martone F, et al. Comparative study of the marginal microleakage of six cements in fixed provisional crowns. J Prosthet Dent. 1998;80(4):417–422. doi: 10.1016/s0022-3913(98)70005-8
- McLean JW, von Fraunhofer JA. The estimation of cement film thickness by an in vivo technique. Br Dent J. 1971;131(3):107–111. doi: 10.1038/sj.bdj.4802708
- Jacobs MS, Windeler AS. An investigation of dental luting cement solubility as a function of the marginal gap. J Prosthet Dent. 1991;65(3):436–442. doi: 10.1016/0022-3913(91)90239-s
- Bergenholtz G, Cox CF, Loesche WJ, et al. Bacterial leakage around dental restorations: its effect on the dental pulp. J Oral Pathol. 1982;11(6):439–450. doi: 10.1111/j.1600-0714.1982.tb00188.x
- Pereira-Cenci T, Cenci MS, Fedorowicz Z, et al. Antibacterial agents in composite restorations for the prevention of dental caries. Cochrane Database Syst Rev. 2013;(12):CD007819. doi: 10.1002/14651858.CD007819.pub3
- Lund MR. Secondary caries, a problem of primary concern. Oper Dent. 2009;34(3):249–250. doi: 10.2341/1559-2863-34.3.249
- Muliyar S, Shameem KA, Thankachan RP, et al. A. microleakage in endodontics. J Int Oral Health. 2014;6(6):99–104.
- Korsch M, Walther W. Peri-implantitis associated with type of cement: a retrospective analysis of different types of cement and their clinical correlation to the peri-implant tissue. Clin Implant Dent Relat Res. 2014;17:e434–e443. doi: 10.1111/cid.12265
- Bahlou L, Mouradian N. Peri-implantitis and the risk factors. Oral Health; 2018. Available from: https://www.oralhealthgroup.com/features/peri-implantitis-risk-factors/
- Pereira S, Anami LC, Pereira CA, et al. Bacterial colonization in the marginal region of ceramic restorations: effects of different cement removal methods and polishing. Oper Dent. 2016;41(6):642–654. doi: 10.2341/15-206-L
- Jakeman RJB, Cheetham AK, Clayden NJ, et al. A Magic angle spinning nmr study of the phase diagram Ca3−xZnx(PO4)2. J Solid State Chem. 1989;78(1):23–34. doi: 10.1016/0022-4596(89)90124-2
- Crisp S, O’Neill IK, Prosser HJ, et al. Infrared spectroscopic studies on the development of crystallinity in dental zinc phosphate cements. J Dent Res. 1978;57(2):245–254. doi: 10.1177/00220345780570021801
- Dupuis V, Laviole O, Potin-Gautier M, et al. Solubility and disintegration of zinc phosphate cement. Biomaterials. 1992;13(7):467–470. doi: 10.1016/0142-9612(92)90168-N
- Flanagan D. Zinc phosphate as a definitive cement for implant-supported crowns and fixed dentures [cited Sep 14, 2019]. Available from: https://www.dovepress.com/zinc-phosphate-as-a-definitive-cement-for-implant-supported-crowns-and-peer-reviewed-fulltext-article-CCIDE. doi: 10.2147/CCIDE.S146544
- Øilo M, Quinn GD. Fracture origins in twenty-two dental alumina crowns. J Mech Behav Biomed Mater. 2016;53:93–103. doi: 10.1016/j.jmbbm.2015.08.006
- Yim NH, Rueggeberg FA, Caughman WF, et al. Effect of dentin desensitizers and cementing agents on retention of full crowns using standardized crown preparations. J Prosthet Dent. 2000;83(4):459–465. doi: 10.1016/s0022-3913(00)70042-4
- Dachi SF, Stigers RW. Reduction of pulpal inflammation and thermal sensitivity in amalgam-restored teeth treated with copal varnish. J Am Dent Assoc. 1967;74(6):1281–1285. doi: 10.14219/jada.archive.1967.0441
- Brännström M, Nyborg H. Cavity treatment with a microbicidal fluoride solution: growth of bacteria and effect on the pulp. J Prosthet Dent. 1973;30(3):303–310. doi: 10.1016/0022-3913(73)90187-x
- Brännström M. Communication between the oral cavity and the dental pulp associated with restorative treatment. Oper Dent. 1984;9(2):57–68.
- Indian dental academy. Microleakage/ Orthodontic Course by Indian Dental Academy, 07:08:31 UTC.
- Behr M, Rosentritt M, Wimmer J, et al. Self-adhesive resin cement versus zinc phosphate luting material: a prospective clinical Trial Begun 2003. Dent Mater. 2009;25(5):601–604. doi: 10.1016/j.dental.2008.11.003
- Wadhwani C, Rapoport D, La Rosa S, et al. Radiographic detection and characteristic patterns of residual excess cement associated with cement-retained implant restorations: a clinical report. J Prosthet Dent. 2012;107(3):151–157. doi: 10.1016/S0022-3913(12)60046-8
- Agar JR, Cameron SM, Hughbanks JC, et al. Cement removal from restorations luted to titanium abutments with simulated subgingival margins. J Prosthet Dent. 1997;78(1):43–47. doi: 10.1016/s0022-3913(97)70086-6
- Valente NA, Andreana S. Peri-implant disease: what we know and what we need to know. J Periodontal Implant Sci. 2016;46(3):136–151. doi: 10.5051/jpis.2016.46.3.136
- Linkevicius T, Puisys A, Vindasiute E, et al. Does residual cement around implant-supported restorations cause peri-implant disease? a retrospective case analysis. Clin Oral Implants Res. 2013;24(11):1179–1184. doi: 10.1111/j.1600-0501.2012.02570.x
- Gryksa M. Characterization of Zirconia/Phosphate Cement Interfaces in Dentistry.
- Whitaker A. The crystal structure of hopeite, Zn3(PO4)2.4H2O. Acta Cryst B. 1975;31(8):2026–2035. doi: 10.1107/S0567740875006784
- Cartz L, Servais G, Rossi F. Surface structure of zinc phosphate dental cements. J Dent Res. 1972;51(6):1668–1671. doi: 10.1177/00220345720510062901