Abstract
This paper presents a glacial geomorphological map of over 17,000 landforms on the bed of a major palaeo-ice stream in Marguerite Bay, western Antarctic Peninsula. The map was compiled using various geophysical datasets from multiple marine research cruises. Eight glacial landform types are identified: mega-scale glacial lineations, crag-and-tails, whalebacks, gouged, grooved and streamlined bedrock, grounding-zone wedges, subglacial meltwater channels, gullies and channels, and iceberg scours. The map represents one of the most complete marine ice-stream signatures available for scrutiny, and these data hold much potential for reconstructing former ice sheet dynamics, testing numerical ice sheet models, and understanding the formation of subglacial bedforms beneath ice streams. In particular, they record a complex bedform signature of palaeo-ice stream flow and retreat since the last glacial maximum, characterised by considerable spatial variability and strongly influenced by the underlying geology. The map is presented at a scale of 1: 750,000, designed to be printed at A2 size, and encompasses an area of 128,420 km2.
1. Introduction
Rapidly flowing ice streams and outlet glaciers account for dynamic mass loss from the Greenland and Antarctic ice sheets, and recent observations of their thinning and acceleration suggest that their contribution to sea-level rise is increasing (CitationPritchard, Arthern, Vaughan, & Edwards, 2009; CitationShepherd et al., 2012). Their pattern of retreat is, however, characterised by a variable, often asynchronous behaviour at short (decadal) time-scales (e.g. CitationMoon, Joughin, Smith, & Howat, 2012; CitationPritchard et al., 2009). This short-term variability introduces considerable uncertainty in understanding recent ice-sheet changes and predicting near future sea-level rise, as highlighted by the Intergovernmental Panel on Climate Change (CitationIPCC, 2007). To provide a long-term context for recent changes, therefore, numerous workers have recognised the potential of reconstructing palaeo-ice streams. There are now well-established criteria for recognising former ice streams in the geological record (CitationDyke & Morris, 1988; CitationStokes & Clark, 1999), which represent important archives for reconstructing ice-stream dynamics and investigating basal processes (e.g. CitationGraham et al. 2009; CitationÓ Cofaigh et al., 2005).
In Antarctica, the advent of multi-beam swath bathymetry systems in the mid 1990s was fundamental in facilitating the identification of marine-terminating palaeo-ice streams from the glacial geomorphic imprint preserved on the sea-floor. Over 30 palaeo-ice streams have now been documented in deep cross-shelf bathymetric troughs surrounding Antarctica, detailing the expansion of ice out towards the continental shelf edge prior to deglaciation during the last glacial maximum (cf. CitationLivingstone et al., 2012 for a review) and several of these have been mapped in detail (e.g. CitationCamerlenghi et al., 2001; CitationEvans, Pudsey, Ó Cofaigh, Morris, & Domack, 2005; CitationGraham et al., 2009; CitationJakobsson et al., 2012; CitationNitsche et al. 2013; CitationReinardy et al., 2011).
One of the best-studied palaeo-ice streams, with a wealth of geophysical data, was located in the area now known as Marguerite Bay on the west side of the Antarctic Peninsula (). It constitutes a 50–80 km wide bathymetric trough, which extends for about 370 km from the inner bay at the mouth of George VI Sound to the continental shelf edge. The trough is characterised by a reverse-sloping bed that deepens from 500 m at the continental shelf edge to 1600 m on the inner shelf () (CitationJamieson et al., 2012). The inner shelf of Marguerite Bay comprises rugged bedrock characterised by large, isolated basins (up to 900 m deep), leading westwards into a network of smaller connected basins 800–1000 m deep (CitationAnderson & Oakes-Fretwell, 2008).
Figure 1. Overview map of Marguerite Bay Ice Stream. Swath bathymetry tracks are as follows: dark blue line: NBP0201 RV/IB Nathaniel B. Palmer cruise tracks; yellow line: JR59; white line: JR71; and orange line: JR157 RRS James Clark Ross cruise tracks. Ages (in calibrated years before present, BP) derived from marine sediment cores (as per CitationLivingstone et al., 2012) are displayed with 1 sigma error and the dates in bold refer to the most reliable core dates (i.e. those derived from calcareous micro-(fossils) and not affected by iceberg turbation). Note that the dates suggest rapid retreat of the outer-mid shelf at ∼14 cal. ka BP, followed by a period of slower retreat through the mid shelf and then another phase of rapid retreat off the inner shelf ∼9 cal. ka BP (see CitationHeroy & Anderson, 2007; CitationKilfeather et al., 2011). APIS: Antarctic Peninsula Ice Sheet; EAIS: East Antarctic Ice Sheet; WAIS: West Antarctic Ice Sheet; LC = Larsen C Ice Shelf; FIS = Filchner Ice Shelf.
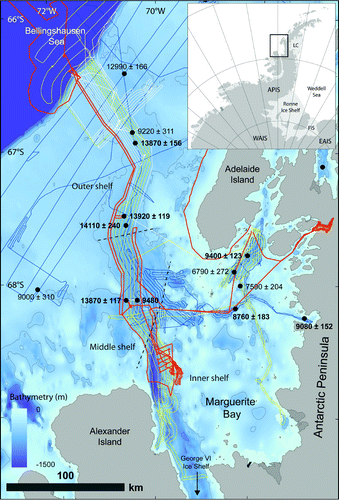
2. Previous work in marguerite bay
Previous marine geophysical and geological studies of the glacial geomorphology of Marguerite Bay document a number of glacial landforms orientated along the axis of the trough and interpreted to indicate streaming flow to the shelf edge during the last glaciation (CitationÓ Cofaigh, Pudsey, Dowdeswell, & Morris, 2002). This includes a transition from ice-moulded bedrock, drumlins and subglacial meltwater channels formed predominantly in crystalline bedrock in the inner bay, to classical drumlins, highly attenuated drumlins and mega-scale glacial lineations (MSGLs) on the mid-shelf. MSGLs up to 20 km in length are formed in sediment across the outer shelf (e.g. CitationAnderson & Oakes-Fretwell, 2008; CitationDowdeswell, Ó Cofaigh, & Pudsey, 2004a, Citation2004b; CitationKennedy & Anderson, 1989; CitationÓ Cofaigh et al., 2002, Citation2005, CitationÓ Cofaigh, Evans, Dowdeswell, & Larter, 2007). Marine sediment cores recovered from the main trough in Marguerite Bay suggest a non-linear pattern of ice-stream retreat characterised by rapid deglaciation of the outer shelf at ∼14 cal. ka BP, followed by a slower phase of retreat through the mid shelf, thought to be associated with the break-up of an ice-shelf (CitationKilfeather et al., 2011). Thereafter, retreat proceeded rapidly to the inner-shelf at ∼9 cal. ka BP (CitationHeroy & Anderson, 2007), with George VI Sound becoming ice free between 6.6 and 9.6 cal. ka BP (CitationHjort, Bentley, & Ingólfsson, 2001; CitationSmith et al., 2007; CitationSugden & Clapperton, 1981). This final phase of deglaciation was accompanied by rapid ice-sheet thinning in the hinterland (CitationBentley et al., 2011). However, despite the wealth of geomorphological, and sedimentological and chronological data available for the Marguerite Bay Palaeo-Ice Stream (MBIS), a detailed map of the ice stream bed has not yet been produced. This paper draws together the available geophysical datasets and compiles a comprehensive map of the glacial landforms and sediments along MBIS (see main map) in order to develop a baseline dataset for investigating the basal properties of ice streams and for testing ice-stream models (e.g. CitationJamieson et al., 2012).
3. Methods
Marine geophysical data were collected on cruises JR59, JR71 and JR157 of the RRS James Clark Ross (JCR) and NBP0201 of the RV/IB Nathaniel B. Palmer (NBP) (). Swath bathymetry data were obtained using Kongsberg EM120 (JCR) and hull-mounted SeaBeam 2100 (NBP) systems. The EM120 system has a 1° × 1° beam configuration and emits 191 beams each with a frequency of 12 kHz and the hull-mounted SeaBeam 2100 system emits 120 beams at 12 kHz. Swath data were processed in MB-System (CitationCaress & Chayes, 2003) to remove anomalous data points, corrected to include sound-velocity profiles, and gridded at 15 × 45 m cell sizes. Vertical and horizontal uncertainties are about 1 and 5 m, respectively. Solar relief-shaded visualisations of the compiled grid were used to conduct the mapping. Two orthogonally shaded images were used to avoid azimuth bias (e.g. CitationSmith & Clark, 2005) and the images were vertically exaggerated 20–50 times to aid identification. Glacial bedforms along Marguerite Trough were manually identified and systematically mapped in ArcGIS 9.3. The bedforms were identified according to conventional criteria (e.g. CitationGraham et al., 2009) listed in and discussed below.
Table 1. Criteria for identifying and mapping glacial landforms (modified from CitationGraham et al., 2009).
4. Glacial landforms
4.1 Mega-scale glacial lineations (MSGLs)
MSGLs are highly attenuated parallel sets of grooves and ridges formed in till and commonly observed on the beds of Antarctic marine palaeo-ice streams (e.g. CitationCanals, Urgeles, & Calafat, 2000; CitationEvans et al., 2005; CitationGraham et al., 2009; CitationShipp, Anderson, & Domack, 1999). Over 5000 MSGLs have been mapped along the length of MBIS, extending all the way to the shelf break, where they are increasingly disrupted by iceberg ploughmarks (). MSGLs are confined to regions of weak till (e.g. CitationÓ Cofaigh et al., 2007) and are 100–18,000 m long and 100–600 m wide, with amplitudes and wavelengths typically between 2 and 8 m and 100–700 m, respectively. The longest MSGLs are observed along the central axis of the outer-shelf trough, with the bedrock-dominated inner- and mid-shelf restricted to shorter bedforms. Although subtle shifts in MSGL orientation are observed along the length of MBIS, often associated with grounding-zone wedge (GZW) positions, they generally conform to the orientation of the trough.
Figure 2. Example of mega-scale glacial lineations (MSGLs) on the outer-shelf of the palaeo-ice stream. The left-hand panel is the relief-shaded image and the right-hand panel shows the mapped landforms (colours are the same as in the main map). The relief-shaded image is x20 exaggeration and is shaded from the NE.
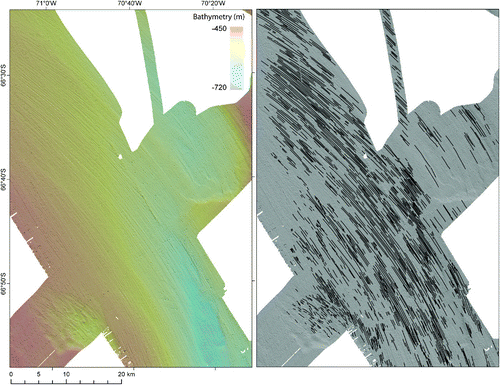
4.2 Crag-and-tails
Crag-and-tails are tear-drop shaped bedforms with exposed bedrock at the stoss end and a tail of tapering sediment (CitationGraham et al., 2009). The tail of sediment relates to the protection or inflow of sediment in the lee of the bedrock crag. These features are restricted to areas of bedrock with an upstream supply of sediment, either as isolated features or broad swathes in the lee of escarpments. Over 450 crag-and-tail bedforms have been mapped along MBIS, predominantly on the mid-shelf where there is a mix of bedrock and unconsolidated sediment (). They display similar characteristics to neighbouring MSGLs, including orientation and size.
4.3 Grooved, gouged and streamlined bedrock
The crystalline bedrock of the inner and mid-shelf has been preferentially eroded parallel to palaeo-ice flow, creating a grooved and streamlined appearance (), similar to other regions around Antarctica (cf. CitationLivingstone et al. 2012; CitationWellner, Heroy, & Anderson, 2006). Grooves, gouges and streamlined ridges tend to be concentrated along the axis of the trough, and across highs separating and surrounding basins (), while the trough flanks and the rough interior of Marguerite Bay are crudely streamlined. The features are typically 10 s of metres deep/high, although streamlined hills up to 200 m high are also observed, albeit rarely. In contrast to MSGLs, the grooves and gouges are often curvilinear, probably related to the structure of the underlying bedrock.
Figure 4. Example of grooved, gouged and streamlined bedrock on the mid-shelf at the transition between crystalline bedrock and sediment. Note the deep roughly parallel gouges on the east side of the image, which give way into streamlined hills, whalebacks and MSGLs further west and north. The left-hand panel is the relief-shaded image and the right-hand panel shows the mapped landforms (colours are the same as in the main map). The relief-shaded image is x20 exaggeration and is shaded from the NE.
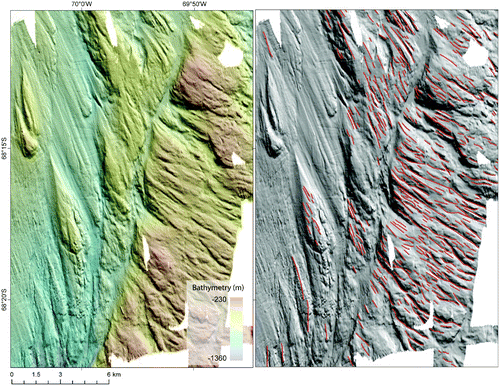
4.4 Whalebacks
Whalebacks are ‘tear-drop’ or ‘oval’ shaped hills formed in bedrock and can be described as symmetric or classically asymmetric (, e.g. CitationRoberts & Long, 2005). They are commonly found on the inner- and mid-shelf of MBIS in crystalline bedrock, and range from crudely sculpted symmetrical features <3 km long, with elongation ratios of 2:1–4:1, to large asymmetric whalebacks up to 10 km long and with elongation ratios up to 18:1 (). In places, whalebacks are associated with crescentic scours around their stoss ends. A clear evolution is apparent between the stubbier, crudely streamlined forms on the inner shelf, especially outside of the main trough, and the more elongate, increasingly asymmetric whalebacks observed towards the mid-shelf and on the floors of the large isolated and connected basins on the rugged inner shelf. Nearly 3000 whalebacks have been mapped along MBIS, whereas drumlins formed in unconsolidated sediment are entirely absent.
Figure 5. Examples of asymmetric whalebacks on the mid-shelf (the polygons have been filled with a red colour to further pick out the features). Note, how these features have been crudely streamlined. The left-hand panel is the relief-shaded image and the right-hand panel shows the mapped landforms (colours are the same as in the main map). The relief-shaded image is x20 exaggeration and is shaded from the NE.
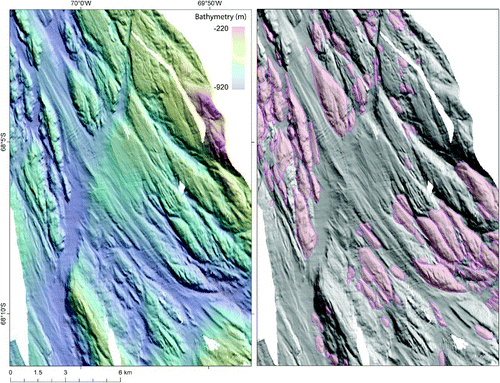
4.5 Meltwater channels
An extensive network of subglacial meltwater channels incised into crystalline bedrock has been identified on the inner- and mid-shelf sections of MBIS (, e.g. CitationAnderson & Oakes-Fretwell, 2008). The channels range from straight to sinuous; have U- to V-shaped cross-profiles; abrupt initiation and termination points; undulating thalwegs; and orientations that vary from parallel to oblique to the palaeo-ice flow direction. Meltwater channels in the rugged eastern interior of Marguerite Bay are small isolated features that typically extend into large basins from bedrock highs and dissect bedrock knolls. These channels evolve westward into a coherent, anastomosing network of large meltwater channels, 300–550 m wide and 30–200 m deep, which connect deep basins and converge towards the main trough. The trough is also characterised by an anastomosing network of well-connected channels that are up to 1.5 km wide and 100 m deep (). The meltwater channels become more disparate seawards of the transition from bedrock to sedimentary substrate, and are not observed on the sediment-floored outer-shelf.
Figure 6. Example of anastomosing meltwater channel network on the mid shelf incised into crystalline bedrock. The left-hand panel is the relief-shaded image and the right-hand panel shows the mapped landforms (colours are the same as in the main map). The relief-shaded image is x20 exaggeration and is shaded from above (birds-eye view).
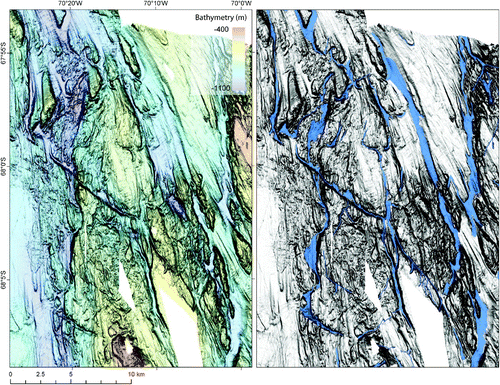
4.6 Grounding zone wedges (GZWs)
GZWs are common features of Antarctic palaeo-ice streams (e.g. CitationGraham et al., 2009; CitationLarter & Vanneste, 1995; CitationÓ Cofaigh et al., 2005; CitationShipp et al., 1999), and are characterised by a steep distal sea-floor ramp and shallow, commonly lineated back-slope (). They are composed of diamicton tens of metres thick and extend for tens of kilometres (CitationLivingstone et al., 2012). They are thought to form by the advection and deposition of sediment at the grounding-line during temporary still-stands or minor readvances (e.g. CitationAlley, Blankenship, Rooney, & Bentley, 1989), although the exact process for sediment advection is debated (see CitationLivingstone et al., 2012). Nine GZWs have been identified on the mid and outer shelf of MBIS, occurring both along the central axis (e.g. ) and flanks of the trough (see also CitationJamieson et al., 2012). Three additional GZWs have been mapped outside the confines of the main trough close to the shelf edge. These GZWs are associated with the partial preservation of MSGLs in front of their scarps. The GZWs are typically 10–40 m thick (maximum 80 m), 5–14 km wide and 3–14 km long.
Figure 7. Example of grounding zone wedge on the outer shelf. Note the subtle difference in orientation of the MSGLs either side of the wedge crest (white line). The left-hand panel is the relief-shaded image and the right-hand panel shows the mapped landforms (colours are the same as in the main map). The relief-shaded image is x20 exaggeration and is shaded from the NE.
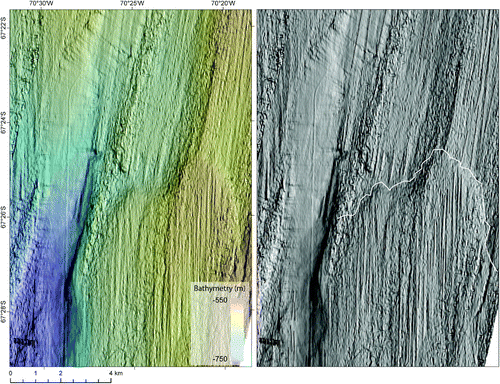
4.7 Gullies and channels
Gullies are preserved on the uppermost continental slope in front of and to either side of the trough mouth (, also see CitationDowdeswell et al., 2004a; CitationNoormets, Dowdeswell, Larter, Ó Cofaigh, & Evans, 2009; CitationÓ Cofaigh, Taylor, Dowdeswell, & Pudsey, 2003). They are characteristically straight to sinuous channels formed in sediment, often with a dendritic pattern converging down-slope. The gullies rarely extend more than 10 km down-slope and display a V-shaped profile. In front of the trough, the gullies display wavelengths between 300 and 1500 m and are typically <120 m deep. Gullies on either side of the trough mouth are typically initiated at the shelf edge, but those offshore of the trough mouth are frequently seeded further down-slope. The gullied upper slope gives way to a smooth lower slope and then into large channels and sediment drifts on the upper continental rise ().
Figure 8. Example of gullies and channels on the continental slope and rise, respectively. The left-hand panel is the relief-shaded image and the right-hand panel shows the mapped landforms (colours are the same as in the main map). The relief-shaded image is x20 exaggeration and is shaded from the NE.
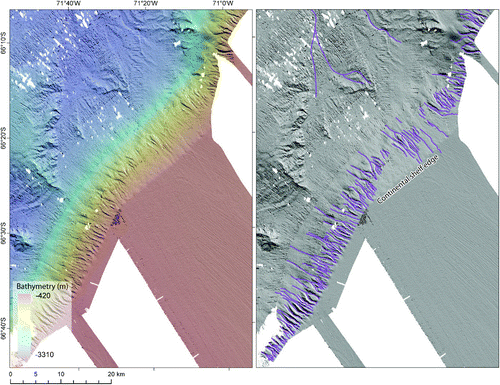
4.8 Iceberg scours
Iceberg scours are present on the sediment-floored mid- and outer-shelf forming cross-cutting curvilinear furrows that are superimposed onto all the other mapped glacial features (). They occur in water depths of 300–750 m, with a mean of 480 m, and become more prevalent towards the outer shelf, where the bed shallows. Although the iceberg scours display multiple orientations, a general SW-NE trend is apparent.
Figure 9. Example of iceberg scours on the outer-shelf. Note that the lineations are increasingly disrupted by iceberg scours and eventually disappear in a westward direction. The left-hand panel is the relief-shaded image and the right-hand panel shows the mapped landforms (colours are the same as in the main map). The relief-shaded image is x20 exaggeration and is shaded from the NE.
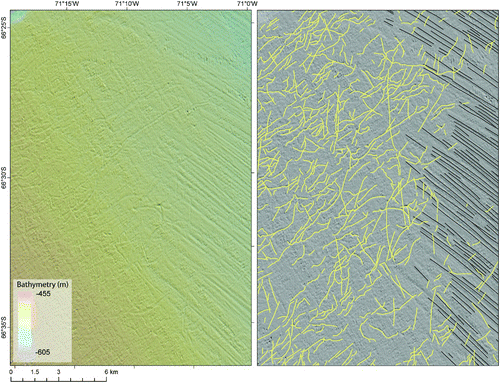
5. Discussion
The map depicts over 17,000 glacial landforms in Marguerite Bay, which record the extent of a significant (>370 km long) palaeo-ice stream along the trough that was fed by ice from George VI Sound and surrounding tributary regions on the inner shelf (cf. CitationÓ Cofaigh et al., 2002, Citation2005). The distribution of MSGLs indicates that ice flow along the central axis of the over-deepened trough was rapid and that ice reached the shelf edge at some point during the last glacial. The identification of twelve GZWs on the landward-sloped outer- and mid-shelf documents the advection and build-up of glacigenic sediment during temporary pauses in the ice stream's recession. These GZWs are within a region thought to have undergone rapid (millennial-scale) retreat from the outer-shelf (CitationKilfeather et al., 2011) and must, therefore, have formed rapidly.
The spatial distribution and evolution of glacial bedforms along the MBIS is typical of Antarctic palaeo-ice streams (e.g. CitationCamerlenghi et al., 2001; CitationCanals et al., 2000; CitationEvans et al., 2005; CitationGraham et al., 2009; CitationJakobsson et al., 2012; CitationWellner et al., 2006). The rough bedrock-dominated inner-shelf region is characterised by a wide variety and spatial arrangement of bedforms, including patches of lineated sediment and a deeply incised anastomosing network of meltwater channels, which converge on the main trough. The highly variable size, elongation and depth of bedrock incisions imply a complex ice-flow history conditioned by the bedrock properties. The bedforms associated with bedrock (channels, whalebacks, and gouged, grooved and streamlined bedrock) were probably eroded over several glacial cycles (and different stages of ice-stream activity) and therefore reflect a long history of seascape evolution heavily influenced by the bedrock structure and geology (e.g. CitationAnderson & Oakes-Fretwell, 2008; CitationCanals et al., 2000; CitationGraham et al., 2009; CitationNitsche et al., 2013; CitationWellner et al., 2006). Conversely, the bedforms in glacial sediments (crag-and-tail, GZWs and MSGLs) are likely to have formed during the most recent phase of ice streaming (e.g. CitationGraham et al., 2009; CitationÓ Cofaigh et al., 2005).
The outer shelf is dominated by highly elongate MSGLs formed in till (e.g. CitationÓ Cofaigh et al., 2007), which probably relate to the final imprint of palaeo-ice stream activity. Although there is considerable variation in MSGL elongation, it generally increases downstream and towards the centre of the trough. Unlike the bedrock-floored inner- and mid-shelf there is no evidence of meltwater channels or related fluvial sediments on the sedimentary substrate of the outer-shelf (e.g. CitationKilfeather et al., 2011; CitationÓ Cofaigh et al., 2007). Bedrock channels on the inner shelf are interpreted to have formed over many glacial cycles (e.g. CitationAnderson & Oakes-Fretwell, 2008) and thus reflect an entirely separate temporal signature of subglacial water production and flow. It is therefore not surprising that similar features are not documented on the sediment-floored outer shelf. However, it is unknown how subglacial meltwater drained through this sector of the palaeo ice-stream. Possible mechanisms include: (i) water flow through meltwater channels or canals below the resolution of the instruments; (ii), flow as part of a dynamic network, where channels were constantly evolving due to the ‘competition’ between water flux and sediment creep; or (iii), via Darcian flow through the sediment (see CitationNoormets et al., 2009).
6. Conclusions and implications
This paper presents a detailed geomorphological map of the glacial landforms along the MBIS. It shows a complex landform arrangement characterised by a progression from bedrock moulded whalebacks, gouged and grooved terrain and deeply incised meltwater channels on the inner shelf to MSGLs formed in unconsolidated sediment on the outer shelf. The mapping carried out in this study is a useful dataset for further detailed quantitative analysis of the bed characteristics of the MBIS and the formation of subglacial bedforms. Furthermore, it offers robust boundary conditions for ice-stream modelling experiments (e.g. CitationJamieson et al., 2012), which require data about the spatial extent and direction of flow in combination with data about the strength of the ice stream bed. With this in mind, the geomorphological record is supplemented by information from marine sediment cores and shallow acoustic seismic profiles collected along the length of the palaeo-ice stream (e.g. Ó Cofaigh et al., Citation2002, Citation2005, Citation2007; CitationKilfeather et al., 2011), which provide further data on the sedimentology and deglacial chronology of the region. The latter is well constrained by marine radiocarbon dates predominantly obtained from calcareous (micro-)fossils (CitationHeroy & Anderson, 2007; CitationKilfeather et al., 2011; CitationLivingstone et al., 2012).
Software
MB-System was used to process and grid the geophysical data collected on the research cruises. Relief-shaded visualisations of the bathymetry data and on-screen digitisation of the landforms were produced using Esri ArcMap 9.3. The map was initially produced in ArcMap 9.3 and then exported to Adobe Illustrator for editing.
Main Map: Glacial Geomorphology of Marguerite Bay Palaeo-Ice Stream, Western Antarctic Peninsula
Download PDF (25.5 MB)Acknowledgments
This work was funded by a NERC standard grant NE/G015430/1. The data is available upon request from the lead author. The authors gratefully acknowledge the comments of two anonymous reviewers that helped improve the paper.
References
- Alley , R. B. , Blankenship , D. D. , Rooney , S. T. and Bentley , C. R. 1989 . Sedimentation beneath ice shelves – The view from Ice Stream B . Marine Geology , 85 : 101 – 120 .
- Anderson , J. B. and Oakes-Fretwell , L. 2008 . Geomorphology of the onset area of a palaeo-ice stream, Marguerite Bay, Antarctica Peninsula . Earth Surface Processes and Landforms , 33 : 503 – 512 .
- Bentley , M. J. , Johnson , J. S. , Hodgson , D. A. , Dunai , T. , Freeman , S. P. H. T. and Cofaigh , Ó. 2011 . Rapid deglaciation of Marguerite Bay, western Antarctic Peninsula in the Early Holocene . Quaternary Science Reviews , 30 : 3338 – 3349 .
- Camerlenghi , A. , Domack , E. , Rebesco , M. , Gilbert , R. , Ishman , S. , Leventer , A. , Brachfeld , S. and Drake , A. 2001 . Glacial morphology and post-glacial contourites in northern Prince Gustav Channel (NW Weddell Sea, Antarctica) . Marine Geophysical Researchers , 22 : 417 – 443 .
- Canals , M. R. , Urgeles , R. and Calafat , A. M. 2000 . Deep sea-floor evidence of past ice streams off the Antarctic Peninsula . Geology , 28 : 31 – 34 .
- Caress, D. W., & Chayes, D. N. (2003). MB-System Version 5. Retrieved from http://www.ldeo.columbia.edu/pi/MB-System Open source software distributed from the MBARI and LDEO web sites
- Dowdeswell , J. A. , Ó Cofaigh , C. and Pudsey , C. J. 2004a . Continental slope morphology and sedimentary processes at the mouth of an Antarctic palaeo ice stream . Marine Geology , 204 : 203 – 214 .
- Dowdeswell , J. A. , Ó Cofaigh , C. and Pudsey , C. J. 2004b . Thickness and extent of the subglacial till layer beneath an Antarctic palaeo-ice stream . Geology , 32 : 13 – 16 .
- Dyke , A. S. and Morris , T. F. 1988 . Drumlin fields, dispersal trains and ice streams in Arctic Canada . Canadian Geographer , 32 : 86 – 90 .
- Evans , J. , Pudsey , C. J. , Ó Cofaigh , C. , Morris , P. W. and Domack , E. W. 2005 . Late quaternary glacial history, dynamics and sedimentation of the eastern margin of the Antarctic Peninsula Ice Sheet . Quaternary Science Reviews , 24 : 741 – 774 .
- Graham , A. G. C. , Larter , R. D. , Gohl , K. , Hillenbrand , C.-D. , Smith , J. A. and Kuhn , G. 2009 . Bedform signature of a West Antarctica palaeo-ice stream reveals a multi-temporal record of flow and substrate control . Quaternary Science Reviews , 28 : 2774 – 2793 .
- Heroy , D. C. and Anderson , J. B. 2007 . Radiocarbon constraints on Antarctic Peninsula Ice sheet retreat following the last glacial maximum (LGM) . Quaternary Science Reviews , 26 : 3286 – 3297 .
- Hjort , C. , Bentley , M. J. and Ingólfsson , O. 2001 . Holocene and pre-Holocene temporary disappearance of the George VI Ice Shelf, Antarctic Peninsula . Antarctic Science , 13 : 296 – 301 .
- Intergovernmental Panel on Climate Change (IPCC). (2007). Climate Change 2007: The physical basis: Contributions of working group 1 to the fourth assessment report, edited by S. Solomon et al., pp. 747–845, Cambridge University Press, Cambridge, U.K.
- Jakobsson , M. , Anderson , J. B. , Nitsche , F. O. , Gyllencreutz , R. , Kirshner , A. E. , Kirchner , N. , O'Regan , M. , Mohammad , R. and Eriksson , B. 2012 . Ice sheet retreat dynamics inferred from glacial morphology of the central Pine Island Bay Trough, West Antarctica . Quaternary Science Reviews , 38 : 1 – 10 .
- Jamieson , S. S. R. , Vieli , A. , Livingstone , S. J. , Ó Cofaigh , C. , Stokes , C. R. , Hillenbrand , C.-D. and Dowdeswell , J. A. 2012 . Ice-stream stability on a reverse bed slope . Nature Geoscience , 5 : 799 – 802 . doi: 10.1038/NGEO1600
- Kennedy , D. S. and Anderson , J. B. 1989 . Glacial-marine sedimentation and Quaternary glacial history of Marguerite Bay, Antarctic Peninsula . Quaternary Research , 31 : 255 – 276 .
- Kilfeather , A. A. , Ó Cofaigh , C. , Lloyd , J. M. , Dowdeswell , J. A. , Xu , S. and Moreton , S. G. 2011 . Ice stream retreat and ice shelf history in Marguerite Bay, Antarctic Peninsula: Sedimentological and formainiferal signatures . Geological Society of America Bulletin , 123 : 997 – 1015 .
- Larter , R. D. and Vanneste , L. E. 1995 . Relict subglacial deltas on the Antarctic Peninsula outer shelf . Geology , 23 : 33 – 36 .
- Livingstone , S. J. , Ó Cofaigh , C. , Stokes , C. R. , Hillenbrand , C.-D. , Vieli , A. and Jamieson , S. S. R. 2012 . Antarctic palaeo-ice streams . Earth-Science Reviews , 111 : 90 – 128 .
- Moon , T. , Joughin , I. , Smith , B. and Howat , I. 2012 . 21st Century evolution of Greenland outlet glacier velocities . Science , 336 : 576 – 578 .
- Nitsche , F. O. , Gohl , K. , Larter , R. D. , Hillenbrand , C.-D. , Kuhn , G. , Smith , J. A. , Jacobs , S. , Anderson , J. B. and Jakobsson , M. 2013 . Paleo ice flow and subglacial meltwater dynamics in Pine Island Bay, West Antarctica . The Cryosphere , 7 : 249 – 262 .
- Noormets , R. , Dowdeswell , J. A. , Larter , R. D. , Ó Cofaigh , C. and Evans , J. 2009 . Morphology of the upper continental slope in the Bellingshausen and Amundsen Seas – Implications for sedimentary processes at the shelf edge of West Antarctica . Marine Geology , 258 : 100 – 114 .
- Ó Cofaigh , C. , Dowdeswell , J. A. , Allen , C. S. , Hiemstra , J. , Pudsey , C. J. , Evans , J. and Evans , D. J. A. 2005 . Flow dynamics and till genesis associated with a marine-based Antarctic palaeo-ice stream . Quaternary Science Reviews , 24 : 709 – 740 .
- Ó Cofaigh , C. , Evans , J. , Dowdeswell , J. A. and Larter , R. D. 2007 . Till characteristics, genesis and transport beneath Antarctic palaeo-ice streams . Journal of Geophysical Research , 112 : F03006 doi: 10.1029/2006JF000606
- Ó Cofaigh , C. , Pudsey , C. J. , Dowdeswell , J. A. and Morris , P. 2002 . Evolution of subglacial bedforms along a paleo-ice stream, Antarctic Peninsula continental shelf . Geophysical Research Letters , 29 10.1029/2001.GL014488, 41–1 to 41–4
- Ó Cofaigh , C. , Taylor , J. , Dowdeswell , J. A. and Pudsey , C. J. 2003 . Palaeo-ice streams, trough mouth fans and high-latitude continental slope sedimentation . Boreas , 32 : 37 – 55 .
- Pritchard , H. D. , Arthern , R. J. , Vaughan , D. G. and Edwards , L. A. 2009 . Extensive dynamic thinning on the margins of the Greenland and Antarctic ice sheets . Nature , 461 : 971 – 975 .
- Reinardy , B. T. I. , Larter , R. D. , Hillenbrand , C.-D. , Murray , T. , Hiemstra , J. F. and Booth , A. D. 2011 . Streaming flow of an Antarctic Peninsula palaeo-ice stream, both by basal sliding and deformation of substrate . Journal of Glaciology , 57 : 596 – 608 .
- Roberts , D. H. and Long , A. J. 2005 . Streamlined bedrock terrain and fast ice flow, Jakobshavns Isbrae, West Antarctica: Implications for ice stream and ice sheet dynamics . Boreas , 34 : 25 – 42 .
- Shepherd , A. , Ivins , E. R. , Geruo , A. , Barletta , V. R. , Bentley , M. J. , Bettadpur , S. , Briggs , K. H. , Bromwich , D. H. , Forsberg , R. , Galin , N. , Horwath , M. , Jacobs , S. , Joughin , I. , King , M. A. , Lenaerts , J. T. M. , Li , J. , Ligtenberg , S. R. M. , Luckman , A. , Luthcke , S. B. , McMillan , M. , Meister , R. , Milne , G. , Mouginot , J. , Muir , A. , Nicoas , J. P. , Paden , J. , Payne , A. J. , Pritchard , H. , Rignot , E. , Rott , H. , Sørensen , L. S. , Scambos , T. A. , Scheuchl , B. , Schrama , E. J. O. , Smith , B. , Sundal , A. V. , van Angelen , J. H. , van de Berg , W. J. , van den Broeke , M. R. , Vaughan , D. G. , Velicogna , I. , Wahr , J. , Whitehouse , P. L. , Wingham , D. J. , Yi , D. , Young , D. and Zwally , H. J. 2012 . A reconciled estimate of ice-sheet mass balance . Science , 338 : 1183 – 1189 .
- Shipp , S. S. , Anderson , J. and Domack , E. 1999 . Late Pleistocene – Holocene retreat of the West Antarctic Ice-Sheet system in the Ross Sea: Part 1 – geophysical results . Geological Society of America Bulletin , 111 : 1486 – 1516 .
- Smith , J. A. , Bentley , M. J. , Hodgson , D. A. , Roberts , S. J. , Leng , M. J. , Lloyd , J. M. , Barrett , M. S. , Bryant , C. and Sugden , D. E. 2007 . Oceanic and atmospheric forcing of early Holocene ice shelf retreat, George VI Ice Shelf, Antarctic Peninsula . Quaternary Science Reviews , 26 : 500 – 516 .
- Smith , M. J. and Clark , C. D. 2005 . Methods for the visualisation of digital elevation models for landform mapping . Earth Surface Processes and Landforms , 30 : 885 – 900 .
- Stokes , C. R. and Clark , C. D. 1999 . Geomorphological criteria for identifying Pleistocene ice streams . Annals of Glaciology , 28 : 67 – 74 .
- Sugden , D. E. and Clapperton , C. M. 1981 . An ice shelf moraine, George VI Sound, Antarctica . Annals of Glaciology , 2 : 135 – 141 .
- Wellner , J. S. , Heroy , D. C. and Anderson , J. B. 2006 . The death mask of the Antarctic ice sheet: Comparison of glacial geomorphic features across the continental shelf . Geomorphology , 75 : 157 – 171 .