Abstract
A 1:15,000 scale map of the glacial geomorphology and surficial geology of the Heinabergsjökull and Skalafellsjökull glacier forelands in southeast Iceland depicts a landsystem imprint of actively receding temperate glaciers in a mountain terrain with a high glacifluvial sediment yield. The landsystem is characterised by the three diagnostic depositional domains for active temperate glacier systems (marginal morainic; subglacial; glacifluvial/glacilacustrine) together with site-specific landform-sediment assemblages indicative of jökulhlaup drainage from ice-dammed lakes. Other features are overridden moraines and fluted kame terraces, indicative of ice-marginal and glacifluvial palimpsests preserved beneath temperate glacier ice. A significant outwash head in front of Heinabergsjökull records the long-term accumulation of proglacial outwash and was responsible for a radical change in proglacial drainage patterns (topographically unrestricted to restricted) once the glacier snout had receded from the ice-contact face of the landform. The Heinabergsjökull/ Skalafellsjökull foreland constitutes a modern analogue for active temperate piedmont lobes associated with the construction of large outwash heads fed by high glacifluvial sediment yields. This is one of the most common glacial depositional scenarios associated with the more restricted, mountain-based, average glaciation style during a typical cold stage.
1. Introduction
The aim of this study was to map, at a scale of 1:15,000, the distribution and evaluate the evolution of the component landform-sediment assemblages which comprise the deglaciated forelands of active temperate piedmont lobes, specifically those at the margins of Heinabergsjökull and Skalafellsjökull, where significant outwash heads and hence depositional overdeepenings have been constructed in upland terrain. The map is then employed in the formulation of a conceptual model for use in palaeoglaciological reconstructions in ancient glaciated terrains, particularly upland settings where thick and extensive sequences of ice-contact glacifluvial deposits predominate but also where landsystem signatures may contain subtle evidence of active temperate glacier behaviour (cf. Kvíárjökull, CitationBennett, Evans, Carbonneau, & Twigg 2010; CitationBennett & Evans 2012).
2. Methods
As no new ground survey was undertaken in the study area, the landform and surficial geology mapping was undertaken on three, non-rectified, and manually stitched aerial photographs taken in 1989 by Landmaelingar Islands. Contours are derived from the existing 1:50,000 topographic map and are also produced by Landmaelingar Islands based on the ISN 93 datum. As the relief of most landforms is less than the existing 20 m contour intervals, the lack of newly surveyed contours does not significantly detract from the representation of the glacial geomorphology. Additionally, distortion inherent in the use of non-rectified aerial photographs was kept to a minimum by the use of the central portions of the images for mapping. The glacier surface is represented by a mask compiled directly from the aerial photographs.
3. Historical evolution of Heinabergsjökull and Skalafellsjökull
Heinabergsjökull and Skalafellsjökull are outlet glaciers of the southeast margin of the Vatnajökull ice cap. Skalafellsjökull presently descends steeply from a lava plateau onto a low elevation foreland, forming a piedmont lobe that is topographically confined on its northern margin by the mountain spur of Hafrafellsháls. Heinabergsjökull presently has the characteristics of a valley glacier due its topographic confinement by the mountain spurs of Hafrafellsháls and Geitakinn. This topographic setting and glacier morphology has resulted in the damming of mountain valleys along the northern margin of Heinabergsjökull, a modern analogue of which is Vatnsdalur (), which periodically drains beneath the glacier snout to produce jokulhlaups on the foreland. During the Little Ice Age the snout also dammed a lake (Dalvatn) in Heinabergsdalur (CitationThorarinsson 1939), which is recorded by flights of shorelines and extensive outcrops of ice-proximal subaqueous lake sediments (CitationBennett, Huddart, & McCormick 2000; CitationEvans, Archer, & Wilson 1999) and which finally emptied during a jokulhlaup in 1938. Since the 1950s the snout of Heinabergsjökull has been in contact with a proglacial lake, which has developed on the ice-proximal side of an ice-contact fan (outwash head, sensu CitationBenn, Kirkbride, Owen, & Brazier 2003; CitationKirkbride 2000).
Figure 1. View over Vatnsdalur in 2008 after a lake drainage event. Icebergs have been stranded on the former lake bed after drainage of lake waters beneath the ice margin.
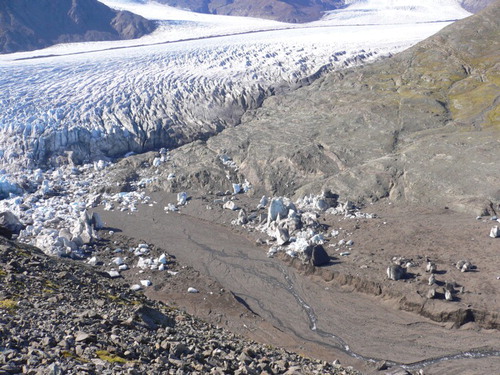
Comparisons of glacier snout positions on aerial photographs taken in 1947, 1957, 1969 and 1989 reveal the rate and extent of snout recession prior to our mapping date (). Refinement of the chronology of snout recession as well as proglacial meltwater channel evolution has been attempted using lichenometry by CitationEvans et al. (1999) and CitationMcKinzey, Orwin, and Bradwell (2004, Citation2005). The employment of different lichenometric dating techniques in these two studies has resulted in contrasting age assignments, especially for the older moraines on the glacier forelands. The age-size approach adopted by CitationEvans et al. (1999), together with their acceptance of historical documentation of an 1887 AD maximum age for the outermost Little Ice Age moraine and associated glacial lake shorelines (CitationThorarinsson 1939), yields a post 1887 AD recession pattern, during which the proglacial outwash migrated from an unconfined easterly flowing incised fan to a southerly draining, topographically confined and terraced, linear sandur that was channelled between the receding glacier snouts and their long-term outwash heads (). The use of the size-frequency method, together with the employment of tephras recovered from the outermost moraines on the foreland, prompted CitationMcKinzey et al. (2004, Citation2005) to propose a longer chronology in which the Little Ice Age limit appears to date to either 1850–1887 AD, using CitationBradwell's (2004) age-gradient curve, or 1818–1886 AD, using CitationBradwell's (2001) age-size curve (); the moraines used for these calculations lie inside CitationEvans et al. (1999) outermost (1887 AD) moraine, thereby inferring that CitationEvans et al. (1999) outer moraine records an older ice limit.
Figure 2. Aerial photograph extracts (Landmaelingar Islands) from 1947, 1957, 1969 and 1989, showing the extent of glacier ice in each year and the sequential development of the proglacial landforms.
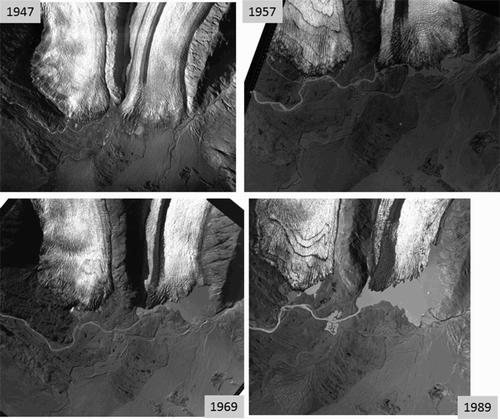
Figure 3. Reconstruction of the historical development of the glacier forelands based upon age-size lichenometric dating and using post1945 aerial photography and historical documentation (from CitationEvans et al. 1999). The coloured arrows depict the generalised ages and flow directions of the proglacial outwash system.
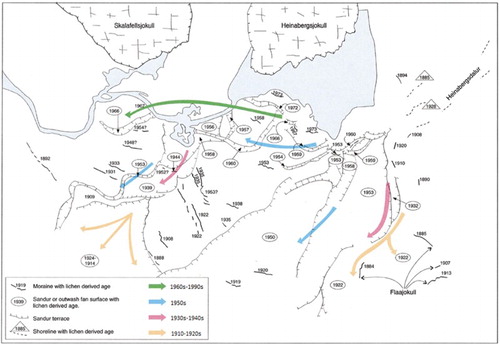
Regardless of the veracity of the exact absolute ages proposed above, the sequential development of the landform-sediment assemblages on the Heinabergsjökull-Skalafellsjökull foreland provides an excellent modern analogue for the historical evolution of active temperate landsystems that have developed in mountain terrains with high glacifluvial sediment yields. This is manifest in: (a) the extensive development of the unconfined sandur fans and large-scale destruction of subglacial and ice-marginal domains during the early stages of post Little Ice Age recession; and (b) the subsequent confinement of outwash into ice-marginal parallel, linear sandar after the later recession of snouts into depositional overdeepenings (CitationCook & Swift 2012) created by outwash heads (CitationBenn et al. 2003; CitationBennett & Evans 2012; CitationKirkbride 2000).
4. Glacial geomorphology and surficial geology
Eight mapping units are identified on the glacier forelands and are now reviewed in turn together with their associated landforms. The units are colour-coded on the map and landforms are depicted using symbology, both following the protocol established for previous Icelandic glacier foreland maps (CitationBennett et al. 2010; CitationEvans & Twigg 2002; CitationEvans, Twigg, & Shand 2006a, Citation2007, Citation2009a, Citation2009b, Citation2010; CitationHowarth & Welch 1969a, Citation1969b).
4.1. Glacifluvial deposits
Similar to the forelands of other southern Vatnajökull piedmont lobes, the forelands of Heinabergsjökull and Skalafellsjökull are dominated by extensive glacial outwash sands and gravels or sandar (). These occur as either coalescent proglacial outwash fans, with pitted and/or collapsed ice-contact surfaces (outwash heads), which have been prograded eastwards over low-relief bedrock outcrops towards the coast, or as narrow strips of ribbon sandur deposited in channels incised through areas of upstanding moraines and fluted subglacial materials. Pitted or collapsed surfaces are manifest as either small, near circular pits or large kettles, the former possessing the characteristics of iceberg melt-out hollows and obstacle marks (CitationFay 2002; CitationMaizels 1992; CitationRussell et al. 2006) and the latter documenting the larger-scale melt-out of remnant glacier ice and concomitant collapse of overlying outwash (e.g. CitationEvans & Rea 2003; CitationEvans, Shand, & Petrie 2009b; CitationEvans & Twigg 2002; CitationFleisher 1986; CitationPrice 1969). The presence of buried glacier ice beneath the large kettles is illustrated by the emergence of an esker in the flooded depression in the former coalescence zone of the two glaciers (). Other large pits located on the steep, terraced ice-contact face of the northernmost Heinabergsjökull fan document the evolution of ice-margin parallel, southerly draining linear sandar (effectively kames interspersed with gravelly push moraines; ) on the proximal face of a large outwash head once the glacier snout had receded below the top of the main westerly draining fan surface. These larger pits contrast with adjacent ice block obstacle marks on the apex of the outwash head, which document the operation of a jokulhlaup-fed sandur when the lake waters from Dalvatn decanted through the margin of Heinabergsjökull in the 1920s and 1930s (CitationThorarinsson 1939). Similar pits on lower and younger easterly draining surfaces within the incised fan document later jokulhlaup drainage, likely fed by the decanting of Vatnsdalur in the 1940s to early 1950s before the snout retreated from the outwash head apex.
Figure 4. View southeastwards across the outwash head at Heinabergsjökull, showing (in the distance) the expansive, terraced surfaces of the proglacial outwash fans grading to the coast and (in the middle distance) the staircases of pitted kame terraces and associated push moraines on the ice-contact face. The tents lie in a large melt-out hollow that abruptly terminates one of the kame terraces feeding in from the left and which demarcates the extent of glacier ice previously buried by glacifluvial terrace sediment. The terraced alluvium that feeds into the modern lake delta is visible in the foreground, with the modern lake on the right.
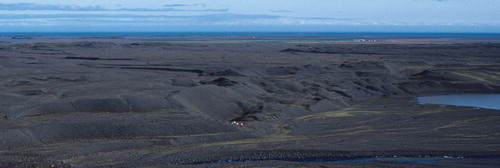
Figure 5. Aerial photograph extracts (Landmaelingar Islands) from 1947, 1957, 1969 and 1989, showing the development of the melt-out of buried glacier ice and concomitant emergence of an esker beneath the glacifluvial outwash corridor at the former suture zone of Heinabergsjökull and Skalafellsjökull.
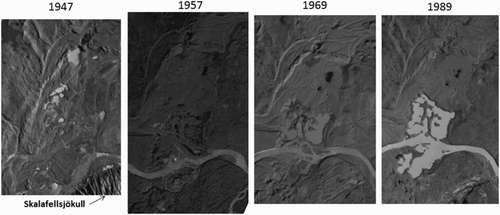
Figure 6. View across the push moraines on the terraced and pitted ice-contact face of the outwash head at Heinabergsjökull. These features document contemporaneous kame terrace formation, snout melt-out and push moraine construction.
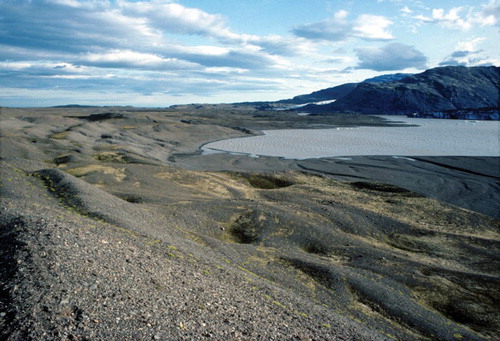
Eskers are rare on the glacier forelands and are concentrated in the former coalescence zone of the two glaciers. In addition to the eskers emerging from the large kettle lake in this zone (), a significant esker ridge stretches along the northern margin of the linear sandur tract and marks the boundary of the moraine-covered subglacial surface of the Heinabergsjökull foreland (). This landform is remarkable in that it has significant relief (up to 10 m) and is composed of boulder gravels. It disappears at the western end of the linear sandur tract where it has been washed out by later meltwater incision and sandur development but it appears to have been originally connected to the apex of the proglacial outwash fan that emanated from the re-entrant produced by the coalescence of the two piedmont lobes at the Little Ice Age maximum. The large grain size characteristics of this esker suggest that it was likely constructed during one of the glaciolimnologically driven jokulhlaups typical of Heinabergsjökull but it is unclear why it was channelled through the suture zone on the south side of the snout rather than through the outwash fan system to the north.
4.2. Till and moraines
The tills of the foreland are typically less than 3 m thick and display all the characteristics of subglacial traction till (CitationEvans 2000; CitationEvans, Phillips, Hiemstra, & Auton 2006b). Thicker tills occur on the low-relief terrain of the foreland where pre-existing outwash fans provided sufficient unconsolidated material for widespread cannibalisation for the subglacial deformation process. Elsewhere, particularly on the southern foreland of Skalafellsjökull, where stepped bedrock outcrops form an undulatory topography, the tills only thicken on the lee sides of roches moutonnées (). Till surfaces are prominently fluted () and they locally thicken to form recessional push moraines, which are constructed on an annual basis, especially on the Skalafellsjökull foreland (Sharp 1984). The moraines typically have a characteristic saw-tooth or crenulated plan form (), which relates to formation along a glacier snout strongly indented by closely spaced, longitudinal crevasses or pecten (CitationPrice 1970). Where closely spaced, the moraines are often locally superimposed.
Figure 8. The thin till cover and stepped bedrock outcrops of the southern margin of Skalafellsjökull: (a) aerial photograph extract showing flutings developed at oblique angles to the crests of elongate bedrock outcrops. Fluted kame terraces are also visible in the top centre of the image (see ); (b) details of the patchy and fluted till cover over bedrock, illustrated by flutings extending from the lee-side faces of roches moutonnées.
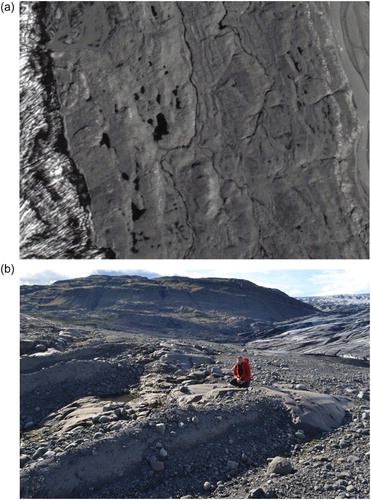
Figure 9. Flutings on the foreland of Skalafeslljökull: (a) aerial photograph extract (Loftmyndir ehf 2007), showing an arcuate zone of flutings associated with a closely spaced cluster of push moraines; (b) ground view of one of the flutings in the aerial photograph, showing striated stoss boulder.
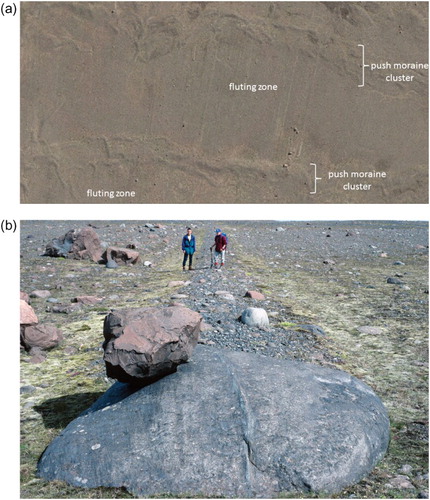
Figure 10. Aerial photograph extract (Loftmyndir ehf 2007) of the Skalafellsjökull foreland showing partially overprinted sawtooth push moraines.
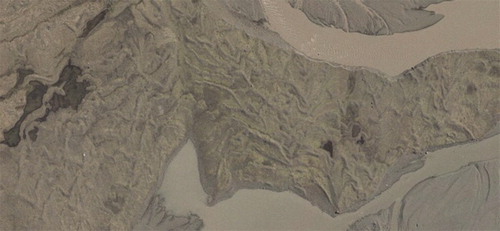
An area of significant moraine superimposition on the Heinabergsjökull foreland is manifest as a complex of relatively higher relief gravelly ridges that cross-cut lower amplitude push moraines ((a)). Unlike the till-cored push moraines, the gravelly ridges contain glacitectonically deformed, stratified sands and gravels ((b)) and therefore likely record a readvance over glacifluvial deposits at some time prior to the 1947 aerial photographs (). Many of these ridges also display rectilinear relationships with one another and lack a consistent ice margin-parallel alignment, appearing instead to be similar to the crevasse-squeeze ridges recognised at other Icelandic glacier margins (cf. CitationEvans & Rea 2003; CitationEvans & Twigg 2002; CitationSharp 1985) but in contrast being composed of sand and gravel and hence classified here as ‘crevasse fill ridges’.
Figure 11. Gravelly push moraine ridges on the Heinabergsjökull foreland: (a) aerial photograph extract (Loftmyndir ehf 2007) showing the pattern of cross-cutting by the higher relief gravelly ridges of the lower amplitude push moraines; (b) exposure through one of the gravelly push moraine ridges showing glacitectonically deformed, stratified sands and gravels.
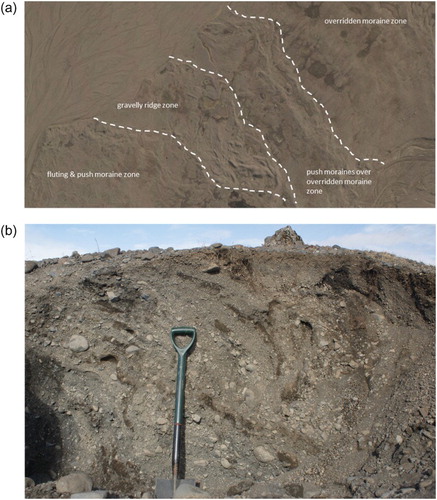
The glacial overriding and preservation of kame terraces beneath flutings is recorded on the south Skalafellsjökull foreland. Here flutings cross a small valley and then lie over terraces deposited along the former glacier margin when it lay parallel to a small valley incised into bedrock ().
Figure 12. Kame terraces with fluted surfaces on the southern foreland of Skalafellsjökull: (a) aerial photograph extract (Loftmyndir ehf 2007) showing the fluted kame terraces in the top right of the image. The terraces were originally deposited on the bedrock slopes of the river valley that runs from the bottom right to top left of the image; (b) ground view across the striated bedrock steps towards the fluted kame terraces.
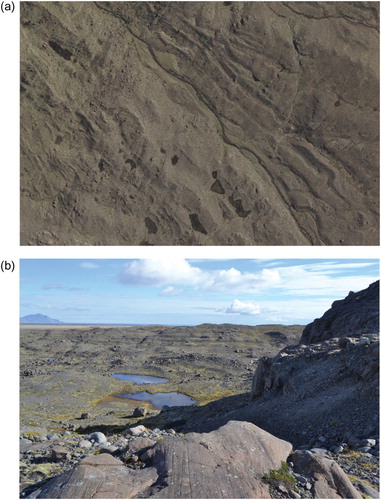
4.3. Overridden moraines
Inset sequences of fluted, low amplitude arcuate ridges represent overridden moraines (cf. CitationEvans et al. 1999, Citation2009b; CitationEvans & Twigg 2002; CitationKruger 1994) deposited prior to or during glacier advance to the historical Little Ice Age limit ((a)). They are superimposed by sharper relief recessional push moraines, whose crests are predominantly orientated parallel with the exception of the gravelly readvance moraines of the Heinabergsjökull foreland; the gravelly moraines cross cut the overridden ridges at an oblique angle ((a)).
4.4. Push moraines developed in ice-contact glacifluvial deposits
The thick and extensive glacifluvial gravels that comprise the Heinabergsjökull outwash head and its associated kame terraces were bulldozed by glacier snout oscillations immediately prior to the taking of the 1957 aerial photographs (). This resulted in the construction of push moraine ridges composed of gravel, interspersed with discontinuous terrace surfaces and melt-out hollows (kettles) (). Small exposures through the moraines near the present lake shoreline reveal glacitectonically folded and thrust stratified sands and fine gravels, previously deposited in the shallow waters of the lake margin.
4.5. Glacilacustrine deposits
Glacilacustrine sediments are well preserved in Heinabergsdalur where they record the former existence of the Dalvatn ice-dammed lake. Prominent lake shorelines are preserved along both sides of the valley up to 140 m and date to the historical Little Ice Age maximum (CitationThorarinsson 1939). More degraded lake shorelines continue up slope to approximately 290 m and include a wide bench at 220–230 m. These higher, more degraded lake deposits record an ice-dammed lake that dates to a pre-Little Ice Age (pre1880s) glacier advance. River cliffs display a sequence of glacilacustrine rhythmites which coarsen upwards into more proximal, gravelly subaqueous mass flow deposits and shallow foreset bedding (CitationBennett et al. 2000; CitationEvans et al. 1999).
4.6. Bedrock and residuum
Outcrops of basalt lava bedrock occur as steep mountain side cliffs and summit tors on Geitakinn, Grástakkur and Hafrafellsháls as well on the lower elevation ridges that rise above the sandar on the glacier forelands. Heavily abraded rock outcrops also occur on Hjallar, on the south part of the Skalafellsjökull foreland, where they are partially covered by fluted till (). In situ bedrock weathering products and mountain summit blockfield is classified as residuum. This can contain patches of deeply weathered or wind deflated pre-Little Ice Age till and is locally draped by aeolian (tephra) deposits and thick peat, especially in the area of Austurland.
4.7. Paraglacial deposits
In addition to reworked glacial deposits on steeper slopes, this minor map unit includes scree slopes and debris flow fans derived from the mechanically weathered bedrock outcrops on Geitakinn, Grástakkur and Hafrafellsháls. In Heinabergsdalur the paraglacial deposits likely represent the remnants of pre-Little Ice Age lake sediments that have been reworked by slope processes on the northeasterly facing surfaces. Numerous small bedrock outcrops also occur within the areas mapped as paraglacial deposits.
4.8. Alluvium
This minor component of the map includes stream deposits filling localised drainage courses. Although this is mainly derived from local slope and stream runoff since deglaciation, a significant body of alluvium is represented by the delta surface on the north side of the Heinabergsjökull proglacial lake. This has been prograded and incised in stages during the recession of the glacier margin and the concomitant expansion and water level drop of the lake. It contains boulder gravels in a series of terraces that have been developed in lower Heinabergsdalur between 80 and 50 m ((a)). The gravels have been likely reworked from the bluffs containing the Dalvatn lake deposits and they lie unconformably over glacitectonically deformed ice-contact sands and gravels ((b)).
5. Glacial landsystem model and its wider implications
Together the component landform suites on the Heinabergsjökull/Skalafellsjökull foreland display the characteristics of the active temperate glacial landsystem previously identified for Icelandic piedmont lobes (CitationEvans 2003, Citation2005, Citation2013; CitationEvans & Twigg 2002; CitationKruger 1994; ). These include the three diagnostic depositional domains: (a) the marginal morainic domain, consisting of inset sequences of minor, annual push moraines, interrupted in places with larger composite moraines related to periods of glacier stillstand (e.g. CitationBoulton 1986; CitationEvans & Hiemstra 2005; CitationKruger 1993, Citation1995); (b) the subglacial domain, including assemblages of flutings, drumlins, and overridden push moraines, locally thinning to reveal ice-scoured bedrock; and (c) the glacifluvial and glacilacustrine domain, comprising proglacial sandur fans, ice margin-parallel outwash corridors/kame terraces, glaciolacustrine depo-centres within lake-filled depressions, and simple, sinuous esker ridges grading into recessional ice-contact fans and pitted outwash.
Figure 14. Oblique Google Earth view across the Heinabergsjökull/Skalafellsjökull foreland showing the main components of the active temperate piedmont lobe and outwash head landsystem.
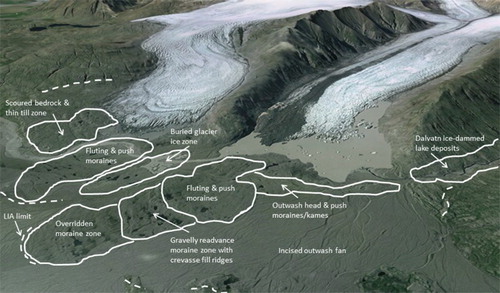
It has been previously acknowledged that these major depositional domains can contain landform-sediment assemblages indicative of site-specific processes, represented at this site by jokulhlaup features (iceberg obstacle marks and melt-out pits) specifically on the proglacial outwash fans of Heinabergsjökull (CitationFay 2002; CitationMaizels 1992, Citation1997; CitationRussell et al. 2006) and documenting the production of a jokulhlaup-fed sandur by the repeated decanting of the Dalvatn (1920s–1930s) and Vatnsdalur (1940s–1950s) ice-dammed lakes (CitationBjornsson 1976; CitationThorarinsson 1939). The development of larger melt-out hollows in the more recently deposited, topographically constrained glacifluvial outwash landforms most likely records the decanting of the ice-dammed lake waters over the glacier snout to later produce a pitted fan, in the same fashion as that observed by CitationPrice (1971) at Breiðamerkurjökull.
Also unusual are the overridden kame terraces on the Skalafellsjökull foreland (). Although overridden moraines are now widely reported on Icelandic active temperate glacier forelands and related to either Little Ice Age glacier advance or snout recession phases during earlier phases of late Holocene glacier activity (CitationEvans et al. 1999, Citation2009b; CitationEvans & Twigg 2002; CitationKruger 1994), the survival of other glacial landforms such as kame terraces has not been previously reported. The fluted kame terraces at Skalafellsjökull therefore serve as an important modern analogue for a number of studies on palimpsest glacial landform records where cold-based ice conditions (in contrast to the temperate snouts of this study) are widely assumed to be a critical factor in landform survival (e.g. CitationDavis, Briner, Coulthard, Finkel, & Miller 2006; CitationForman, Mann, & Miller 1987; CitationHattestrand & Stroeven 2002; CitationLandvik et al. 2005).
A further significant landform component on the Heinabergsjökull side of the foreland is the large depositional overdeepening (cf. CitationCook & Swift 2012), likely created by the long-term accumulation of proglacial outwash fans which regionally account for the thick sequences of stratified glacifluvial gravels and sands that lie beneath Icelandic subglacial tills (cf. Boulton 1987; CitationEvans 2000; CitationEvans & Twigg 2002). The ice-contact face of this feature constitutes an outwash head (sensu CitationBenn et al. 2003; CitationKirkbride 2000) adorned with pitted outwash tracts, glacier snout melt-out hollows and kame terraces partially bulldozed into gravelly push moraines (). This provides us with a modern analogue for the complexities of landsystem development in active temperate settings associated with substantial glacifluvial sedimentation and overdeepenings, where proglacial drainage patterns change significantly during ice recession due to the development of topographic constraints on glacial deposition. A similar landform assemblage is presently emerging from the receding snout of Kvíárjökull (CitationBennett et al. 2010; CitationBennett & Evans 2012) where contemporaneous-controlled moraine development and ice-marginal pushing of glacifluvial sediments accumulating on the ice-contact face of an outwash fan are operating at the margins of a bedrock overdeepening (cf. CitationEvans 2009; CitationSpedding & Evans 2002). In contrast to the Kvíárjökull setting, where significant englacial debris concentrations are driving the development of incremental stagnation on the outwash head within the topographic confinement of an unusually large latero-frontal moraine amphitheatre, the Heinabergsjökull depositional setting is characterised by push moraine construction by a relatively debris-poor snout on an unconfined foreland. This has resulted in the overprinting of outwash fan surfaces with gravelly, annual recessional push moraines and flutings and easterly draining outwash fans (), a landsystem signature similar to that of other active temperate piedmont lobes of south Vatnajökull. Recession back inside the overridden and substantial long-term outwash head in the 1950s resulted in the abandonment of the unrestricted outwash fans and a fundamental change in depositional style as a consequence of the confinement of glacifluvial outwash tracts between the thinning glacier snout and the emerging outwash head.
The mapping of forelands of receding glacier snouts in glacierised terrains such as Iceland, allow us to elucidate the nature of spatial and temporal landform evolution and hence provide modern analogues for palaeoglaciological reconstruction. In particular, the Heinabergsjökull/Skalafellsjökull foreland is an instructive modern analogue for the evolution of active temperate landsystems that have developed in mountain terrains with high glacifluvial sediment yields. This is a palaeoglaciological setting that numerical palaeo-ice sheet modelling (e.g CitationHubbard et al. 2009) reveals to be the dominant or average style of glaciation (CitationMcCarroll 2006; CitationPorter 1989) during a typical cold stage (i.e. upland glaciation with lowland piedmont lobes or valley glaciers). Such average glacial conditions are therefore likely responsible for the most significant glacial landform imprints in ancient glacial landscapes, specifically in the hard, resistant bedrock terrains that acted as centres of ice inception and dispersal throughout most of a cold stage.
6. Conclusion
The 1:15,000 scale map of the glacial geomorphology and surficial geology of the Heinabergsjökull and Skalafellsjökull glacier forelands in southeast Iceland depicts the landform-sediment assemblages that record the recession of active temperate glaciers in a mountain terrain with a high glacifluvial sediment yield fed by frequent catastrophic ice-dammed lake drainage events (). The glacial landsystem model that emerges from this mapping is characterised by the three diagnostic depositional domains for active temperate glacier systems (marginal morainic; subglacial; glacifluvial/glacilacustrine) together with site-specific landform-sediment assemblages indicative of jokulhlaup drainage from ice-dammed lakes. In addition to the overridden moraines that are routinely identified in the subglacial domain of the active temperate glacial landsystem, the foreland also contains fluted kame terraces, indicative of glacifluvial palimpsests preserved beneath temperate glacier ice. A significant outwash head in front of Heinabergsjökull records the long-term accumulation of proglacial outwash and was responsible for a radical change in proglacial drainage patterns (topographically unrestricted to restricted) once the glacier snout had receded from the ice-contact face. This is manifest in the development of pitted outwash tracts, glacier snout melt-out hollows and kame terraces partially bulldozed into gravelly push moraines on the ice-contact face (). The Heinabergsjökull/Skalafellsjökull foreland constitutes a modern analogue for active temperate piedmont lobes associated with the construction of large outwash heads fed by high glacifluvial sediment yields. In a palaeoglaciological sense this is likely one of the most common glacial depositional scenarios within the average style of glaciation during a typical cold stage.
Main Map: Heinabergsjökull and Skalafellsjökull, Iceland: Active Temperate Piedmont Lobe and Outwash Head Glacial Landsystem
Download JPEG Image (37.8 MB)References
- Benn, D. I., Kirkbride, M. P., Owen, L. A., & Brazier, V. (2003). Glaciated valley landsystems. In D. J. A. Evans (Ed.), Glacial landsystems (pp. 372–406). London: Arnold.
- Bennett, G. L., & Evans, D. J. A. (2012). Glacier retreat and landform production on an overdeepened glacier foreland: The debris-charged glacial landsystem at Kvíárjökull, Iceland. Earth Surface Processes and Landforms, 37, 1584–1602. doi: 10.1002/esp.3259
- Bennett, G. L., Evans, D. J. A., Carbonneau, P., & Twigg, D. R. (2010). Evolution of a debris-charged glacier landsystem, Kvíárjökull, Iceland. Journal of Maps, 2010, 40–76. doi: 10.4113/jom.2010.1114
- Bennett, M. R., Huddart, D., & McCormick, T. (2000). The glaciolacustrine landform-sediment assemblage at Heinabergsjökull, Iceland. Geografiska Annaler, 82A, 1–16. doi: 10.1111/j.0435-3676.2000.00107.x
- Bjornsson, H. (1976). Marginal and supraglacial lakes in Iceland. Jökull, 26, 40–50.
- Boulton, G. S. (1986). Push moraines and glacier contact fans in marine and terrestrial environments. Sedimentology, 33, 677–698. doi: 10.1111/j.1365-3091.1986.tb01969.x
- Boulton, G. S. (1987). A theory of drumlin formation by subglacial sediment deformation. In J. Menzies & J. Rose (Eds.), Drumlin symposium (pp. 25–80). Rotterdam: Balkema.
- Bradwell, T. (2001). A new lichenometric dating curve for southeast Iceland. Geografiska Annaler, 83A, 91–101. doi: 10.1111/j.0435-3676.2001.00146.x
- Bradwell, T. (2004). Lichenometric dating in southeast Iceland – The size-frequency approach. Geografiska Annaler, 86A, 31–41. doi: 10.1111/j.0435-3676.2004.00211.x
- Cook, S. J., & Swift, D. A. (2012). Subglacial basins: Their origin and importance in glacial systems and landscapes. Earth Science Reviews, 115, 332–372. doi: 10.1016/j.earscirev.2012.09.009
- Davis, P. T., Briner, J. P., Coulthard, R. D., Finkel, R. W., & Miller, G. H. (2006). Preservation of Arctic landscapes overridden by cold-based ice sheets. Quaternary Research, 65, 156–163. doi: 10.1016/j.yqres.2005.08.019
- Evans, D. J. A. (2000). A gravel outwash/deformation till continuum, Skalafellsjökull, Iceland. Geografiska Annaler, 82A, 499–512. doi: 10.1111/j.0435-3676.2000.00137.x
- Evans, D. J. A. (2003). Ice-marginal terrestrial landsystems: Active temperate glacier margins. In D. J. A. Evans (Ed.), Glacial landsystems (pp. 12–43). London: Arnold.
- Evans, D. J. A. (2005). The glacier-marginal landsystems of Iceland. In C. J. Caseldine, A. J. Russell, J. Harjardottir, & O. Knudsen (Eds.), Iceland: Modern processes and past environments (pp. 93–126). Amsterdam: Elsevier.
- Evans, D. J. A. (2009). Controlled moraines: Origins, characteristics and paleoglaciological implications. Quaternary Science Reviews, 28, 183–208. doi: 10.1016/j.quascirev.2008.10.024
- Evans, D. J. A. (2013). The glacial and periglacial research – Geomorphology and retreating glaciers. In J. Shroder (Editor in Chief), R. Giardino, J. Harbor (Eds.), Treatise on geomorphology. Academic Press, San Diego, CA, vol. 8, Glacial and Periglacial Geomorphology, pp. 460–478.
- Evans, D. J. A., Archer, S., & Wilson, D. J. H. (1999). A comparison of the lichenometric and Schmidt hammer dating techniques based on data from the proglacial areas of some Icelandic glaciers. Quaternary Science Reviews, 18, 13–41. doi: 10.1016/S0277-3791(98)00098-5
- Evans, D. J. A., & Hiemstra, J. F. (2005). Till deposition by glacier submarginal, incremental thickening. Earth Surface Processes and Landforms, 30, 1633–1662. doi: 10.1002/esp.1224
- Evans, D. J. A., Phillips, E. R., Hiemstra, J. F., & Auton, C. A. (2006b). Subglacial till: Formation, sedimentary characteristics and classification. Earth Science Reviews, 78, 115–176. doi: 10.1016/j.earscirev.2006.04.001
- Evans, D. J. A., & Rea, B. R. (2003). Surging glacier landsystem. In D. J. A. Evans (Ed.), Glacial landsystems (pp. 259–288). London: Arnold.
- Evans, D. J. A., Shand, M., & Petrie, G. (2009b). Maps of the snout and proglacial landforms of Fjallsjökull, Iceland (1945, 1965, 1998). Scottish Geographical Journal, 125, 304–320. doi: 10.1080/14702540903364310
- Evans, D. J. A., & Twigg, D. R. (2002). The active temperate glacial landsystem: A model based on Breiðamerkurjökull and Fjallsjökull, Iceland. Quaternary Science Reviews, 21, 2143–2177. doi: 10.1016/S0277-3791(02)00019-7
- Evans, D. J. A., Twigg, D. R., & Orton, C. (2010). Satujökull glacial landsystem, Iceland. Journal of Maps, 6, 639–650. doi: 10.4113/jom.2010.1129
- Evans, D. J. A., Twigg, D. R., Rea, B. R., & Orton, C. (2009a). Surging glacier landsystem of Tungnaárjökull, Iceland. Journal of Maps, 5, 134–151. doi: 10.4113/jom.2009.1064
- Evans, D. J. A., Twigg, D. R., Rea, B. R., & Shand, M. (2007). Surficial geology and geomorphology of the Bruarjökull surging glacier landsystem. Journal of Maps, 3, 349–367. doi: 10.1080/jom.2007.9710850
- Evans, D. J. A., Twigg, D. R., & Shand, M. (2006a). Surficial geology and geomorphology of the Þorisjökull plateau icefield, west-central Iceland. Journal of Maps, 2, 17–29. doi: 10.4113/jom.2006.52
- Fay, H. (2002). Formation of ice block obstacle marks during the November 1996 glacier-outburst flood (jökulhlaup), Skeiðararsandur, southern Iceland. In I. P. Martini, V. R. Baker, & G. Garzon (Eds.), Flood and megaflood deposits: Recent and ancient (pp. 85–97). International Association of Sedimentologists. Special Publication No. 32.
- Fleisher, P. J. (1986). Dead ice sinks and moats: Environments of stagnant ice deposition. Geology, 14, 39–42. doi: 10.1130/0091-7613(1986)14<39:DSAMEO>2.0.CO;2
- Forman, S. L., Mann, D. H., & Miller, G. H. (1987). Late Weichselian and Holocene relative sea level history of Broggerhalvoya, Spitsbergen. Quaternary Research, 27, 41–50. doi: 10.1016/0033-5894(87)90048-2
- Hattestrand, C., & Stroeven, A. P. (2002). A relict landscape in the centre of Fennoscandian glaciation: geomorphological evidence of minimal Quaternary glacial erosion. Geomorphology, 44, 127–143. doi: 10.1016/S0169-555X(01)00149-0
- Howarth, P. J., & Welch, R. (1969a). Breiðamerkurjökull, South-east Iceland, August 1945. 1:30,000 scale map, University of Glasgow.
- Howarth, P. J., & Welch, R. (1969b). Breiðamerkurjökull, South-east Iceland, August 1965. 1:30,000 scale map, University of Glasgow.
- Hubbard, A., Bradwell, T., Golledge, N., Hall, A., Patton, H., Sugden, D., Cooper, R., & Stoker, M. (2009). Dynamic cycles, ice-streams and their impact on the extent, chronology and deglaciation of the British–Irish Ice Sheet. Quaternary Science Reviews, 28, 758–776. doi: 10.1016/j.quascirev.2008.12.026
- Kirkbride, M. P. (2000). Ice marginal geomorphology and Holocene expansion of debris-covered Tasman Glacier, New Zealand. In M. Nakawo, C. Raymond, & A. Fountain (Eds.), Debris-covered glaciers (pp. 211–217). IAHS Publication 264.
- Krüger, J. (1993). Moraine-ridge formation along a stationary ice front in Iceland. Boreas, 22, 101–109. doi: 10.1111/j.1502-3885.1993.tb00169.x
- Krüger, J. (1994). Glacial processes, sediments, landforms and stratigraphy in the terminus region of Myrdalsjökull, Iceland. Folia Geographica Danica, 21, 1–233.
- Krüger, J. (1995). Origin, chronology and climatological significance of annual moraine ridges at Myrdalsjökull, Iceland. The Holocene, 5, 420–427. doi: 10.1177/095968369500500404
- Landvik, J. Y., Ingolfsson, O., Mienert, J., Lehman, S. J., Solheim, A., Elverhoi, A., & Ottesen, D. (2005). Rethinking Late Weichselian ice sheet dynamics in coastal NW Svalbard. Boreas, 34, 7–24. doi: 10.1080/03009480510012809
- Maizels, J. K. (1992). Boulder ring structures produced during jokulhlaup flows: Origin and hydraulic significance. Geografiska Annaler, 74A, 21–33. doi: 10.2307/521467
- Maizels, J. (1997). Jokulhlaup deposits in proglacial areas. Quaternary Science Reviews, 16, 793–819.
- McCarroll, D. (2006). Average glacial conditions and the landscape of Snowdonia. In P. G. Knight (Ed.), Glacier science and environmental change (pp. 266–268). Oxford: Blackwell.
- McKinzey, K. M., Orwin, J. F., & Bradwell, T. (2004) Re-dating the moraines at Skalafellsjökull and Heinabergsjökull using different lichenometric methods: Implications for the timing of the Icelandic Little Ice Age maximum. Geografiska Annaler, 86A, 319–335. doi: 10.1111/j.0435-3676.2004.00235.x
- McKinzey, K. M., Orwin, J. F., & Bradwell, T. (2005) A revised chronology of key Vatnajökull (Iceland) outlet glaciers during the Little Ice Age. Annals of Glaciology, 42, 171–179. doi: 10.3189/172756405781812817
- Porter, S. C. (1989). Some geological implications of average Quaternary glacial conditions. Quaternary Research, 32, 245–261. doi: 10.1016/0033-5894(89)90092-6
- Price, R. J. (1969). Moraines, sandar, kames and eskers near Breiðamerkurjökull, Iceland. Transactions of the Institute of British Geographers, 46, 17–43. doi: 10.2307/621406
- Price, R. J. (1970). Moraines at Fjallsjökull, Iceland. Arctic and Alpine Research, 2, 27–42. doi: 10.2307/1550139
- Price, R. J. (1971). The development and destruction of a sandur, Breiðamerkurjökull, Iceland. Arctic and Alpine Research, 3, 225–237. doi: 10.2307/1550195
- Russell, A. J., Roberts, M. J., Fay, H., Marren, P. M., Cassidy, N. J., Tweed, F. S., & Harris, T. (2006). Icelandic jökulhlaup impacts: Implications for ice-sheet hydrology, sediment transfer and geomorphology. Geomorphology, 75, 33–640. doi: 10.1016/j.geomorph.2005.05.018
- Sharp, M. J. (1984). Annual moraine ridges at Skalafellsjökull, south-east Iceland. Journal of Glaciology, 30, 82–93.
- Sharp, M. J. (1985). “Crevasse-fill“ ridges: A landform type characteristic of surging glaciers? Geografiska Annaler, 67A, 213–220.
- Spedding, N., & Evans, D. J. A. (2002). Sediments and landforms at Kvíárjökull, southeast Iceland: A reappraisal of the glaciated valley landsystem. Sedimentary Geology, 149, 21–42. doi: 10.1016/S0037-0738(01)00242-1
- Thorarinsson, S. (1939). The ice dammed lakes of Iceland with particular reference to their values as indicators of glacial oscillation. Geografiska Annaler, 21, 216–242. doi: 10.2307/520011