Abstract
This paper illustrates the methodology and techniques for the compilation of a thematic (engineering) geological map based on detailed mapping of the weathering grade of crystalline rocks occurring in a portion of the Sila Massif close to the San Giovanni in Fiore Village (Calabria, Italy). The map (1:5000 scale), covering an area of about 20 km2, was compiled combining new geological and structural data with the results of a weathering grade field survey. The methodology, used to distinguish and map the weathering grade classes, was performed using qualitative criteria, semi-quantitative tests, and petrographic analysis of weathered rock samples. The Main Map, presented in this paper, aims to provide a useful tool for land-use planning, for geological hazard assessment and engineering perspectives.
1. Introduction
Weathering intensity in rocks results in mineralogical modification of the primary minerals and strong structural and textural changes in the rock fabric, as fractures in both intercrystalline boundaries and intracrystalline contacts (CitationBorrelli, Perri, Critelli, & Gullà, 2014; CitationCritelli, Di Nocera, & Le Pera, 1991; CitationLe Pera, Critelli, & Sorriso-Valvo, 2001; CitationRegmi, Yoshida, Dhital, & Devkota, 2014; CitationScarciglia, Le Pera, & Critelli, 2007). These structural and mineralogical changes are responsible for generating variable thickness of weathered rocks. In regions where crystalline rocks are widespread, slope instability and morphodynamic evolution of slopes are strongly related to both the thickness of weathering profiles and intensity of weathering (CitationBorrelli, Greco, & Gullà, 2007; CitationCalcaterra & Parise, 2010; CitationCascini, Critelli, Di Nocera, Gullà, & Matano, 1992; CitationChigira, 2001; CitationChigira, Mohamad, Sian, & Komoo, 2011; CitationLacerda, 2007; CitationLe Pera & Sorriso-Valvo, 2000a, Citation2000b; CitationRegmi, Yoshida, Dhital, & Devkota, 2013; CitationTurkington, Phillips, & Campbell, 2005).
Traditional geological and lithological maps are not usually efficient to determine and quantify the weathering processes and mapping the rock-weathering profiles in detail, because the characterization of weathering features is not the purpose of geological maps.
The large occurrence of mid-crustal rocks in the Calabria region, southern Italy, experienced intense mechanical and chemical weathering that is responsible for generating moderate-to-deep weathering profiles. In this region, geological maps are detailed in their general lithological and geological relationships, offering sufficient geological information at various scales of detail. However, these maps include little information about the weathering intensity of rocks except some general descriptions in the legend.
The compilation of a weathering grade map, for crystalline rocks, includes the key features, classification and mapping of the weathering intensity at outcrop scale (CitationBorrelli, Cofone, Critelli, Greco, & Gulla’, 2012; CitationBorrelli, Critelli, Gullà, & Muto, in press; CitationBorrelli et al., 2007; CitationCalcaterra & Parise, 2010; CitationCascini et al., 1992; CitationDi Nocera & Matano, 2002; CitationGeological Society Engineering Group Working Party [GSEGWP], 1995; CitationIetto, Perri, & Fortunato, in press; CitationInternational Association of Engineering Geology [IAEG], 1976; CitationMaharaj, 1995; CitationMalomo, Olorunniwo, & Ogunsanwo, 1983). The thematic map, which provides the distinction between different weathering classes of the rocks – characterized by comparable mechanical behaviour – is useful for environmental geology, engineering geology, territorial planning, and management purposes.
Because the weathering grade of the outcropping crystalline rocks reflects their engineering characteristics and performances (CitationBaynes, Dearman, & Irfan, 1978; CitationCascini & Gullà, 1993; CitationCeryan, Tudes, & Ceryan, 2008; CitationDearman & Matula, 1976; CitationGeotechnical Control Office [GCO], 1988; CitationGullà, Aceto, & Borrelli, 2012; CitationIAEG, 1981; CitationIrfan, 1996; CitationIrfan & Dearman, 1978; CitationRegmi et al., 2014), this paper is focused on the subdivision and mapping of the weathering grade, through observation of geologically distinctive characteristics and qualitative and semi-quantitative engineering–geological tests (CitationBorrelli et al., in press; CitationBorrelli et al., 2007; CitationGullà & Matano, 1997).
The weathering grade map was compiled for an area of 20 km2 (), located on the eastern side of the Sila Massif, close to the San Giovanni in Fiore Village (northern Calabria, Italy).
The key results of this study are summarized in the ‘Main Map’ at large scale (1:5000). The map reflects the effects of weathering in an area characterized by active tectonics and fast landscape evolution, providing an essential contribution to the assessment of soil erosion and landslide hazards.
2. Geological features of the Sila Massif
The Sila Massif, a section of the Hercynian orogenic belt of Western Europe (CitationAmodio-Morelli et al., 1976), is one of the key zones of the Calabrian terranes (CitationCritelli, Muto, Tripodi, & Perri, 2013; CitationMessina et al., 1994; CitationTansi, Muto, Critelli, & Iovine, 2007; CitationVan Dijk et al., 2000). Its structure has been formed since the Late Oligocene–Early Miocene, in response to accretionary processes towards the Adriatic foreland and, since the Tortonian, it became a morphostructural high having elevation mostly similar to the present day (CitationBarone, Dominici, Muto, & Critelli, 2008; CitationCritelli et al., 2013; CitationMuto, Spina, Tripodi, & Critelli, 2014; CitationZecchin, Caffau, et al., 2013; CitationZecchin, Civile, et al., 2013). The uppermost crystalline nappe of the Calabrian Arc tectonic edifice is made up of Palaeozoic metamorphic (from low-to-high-grade rocks) and plutonic rocks included in the Sila Unit (CitationMessina et al., 1994).
From a tectonic perspective, the Sila Massif is characterized by a structural style mainly dominated by the intersection of regional fault systems trending N–S and NW–SE (CitationBorrelli et al., in press; CitationCorbi et al., 2009; CitationSpina, Tondi, & Mazzoli, 2011; CitationVan Dijk et al., 2000).
Since the Late Miocene, the basement rocks of the Sila Massif experienced intense weathering processes resulting in weathered landforms and deep weathering profiles (CitationBorrelli, Perri, Critelli, & Gullà, 2012; CitationBorrelli et al., 2014; CitationGuzzetta, 1974; CitationLe Pera et al., 2001; CitationLe Pera & Sorriso-Valvo, 2000a, Citation2000b; CitationMatano & Di Nocera, 1999; CitationPerri, Scarciglia, Apollaro, & Marini, in press; CitationScarciglia, in press). The intense erosion, activated by the Pleistocene uplift which prevailed over the Quaternary weathering processes, did not completely remove the exposed deeply weathered mantle (CitationCalcaterra & Parise, 2010; CitationMatano & Di Nocera, 1999; CitationMolin, Pazzaglia, & Dramis, 2004; CitationScarciglia, Le Pera, & Critelli, 2005; CitationScarciglia et al., 2007; CitationScarciglia, Le Pera, Vecchio, & Critelli, 2005).
3. Methods
In the first stage, geological and structural investigations were carried out through aerial photo interpretation and field surveys at 1:5000 scale, both to investigate and verify the morphological evidence related to tectonics, and to identify the rock types outcropping in the study area.
In the second stage, the study focused on the weathering grade survey (CitationBorrelli et al., in press; CitationDi Nocera & Matano, 2002; CitationGullà & Matano, 1997) by means of a procedure composed of five phases: Phase 1, photo-interpretative analysis; Phase 2, weathering surveys; Phase 3, detailed weathering grade survey of the most significant cut slopes; Phase 4, petrographic analysis of weathered rock samples; Phase 5, analysis of the collected data and production of the weathering grade map.
Phase 1 distinguished the plutonic rocks into two macro-classes of weathering (CitationBorrelli, Cofone, & Gulla’, 2012): areas affected by soils formed by in situ weathering (residual soils) or transported soils (colluvial and detrital soils); areas affected by crystalline bedrock outcrop (with typical mechanical behaviour of weak or hard rock). The main geo-environmental features used in this preliminary analysis were described in CitationBorrelli et al. (in press).
In Phase 2, the field weathering survey of the area along road cuts, river valleys, and exposed sections was carried out via specific codified procedures to distinguish the different outcropping classes of weathering. The criteria, simplified for large areas, were suggested by CitationGCO (1988), CitationCascini et al. (1992), CitationGSE-GWPR (1995), and CitationBorrelli et al. (in press), that subdivide the weathering grade into six classes (): class VI (residual and colluvial soils and detrital weathered material); class V (completely weathered rock); class IV (highly weathered rock); class III (moderately weathered rock); class II (slightly weathered rock); class I (fresh rock). During the weathering field surveys, the main engineering–geological features of the different classes were obtained through the visual recognition of mineral alteration, rock and soil ratio, presence of original texture and structure, joint staining, and degree of discolouration. In particular, for a quick identification (CitationBorrelli et al., in press), the rock strength can only be assessed by a geological hammer (e.g. sound emitted from the rock when it is struck with the hammer, hammer head effect, hammer point effect, hammer breaking), and trying to break rock fragments – length about 15 cm – with the hands.
Figure 2. Reference descriptions for the weathering classes (modified from CitationBorrelli et al., in press).
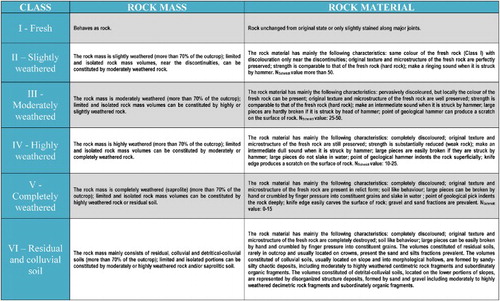
In order to collect more data on the thickness and geometrical characteristics of the different weathering horizons, in Phase 3 of the analysis, a detailed survey of weathering profiles was performed on 58 cut slopes. The weathering profiles were studied following the methodology proposed by CitationGullà and Matano (1997) which considers the use of both qualitative (e.g. rock colour, discolouration processes, samples broken by hands and hammer) and semi-quantitative criteria (e.g. Schmidt Hammer tests). The observations and the measures carried out to define the weathering grade on each cut slope were reported in the storage form shown in .
Figure 3. Data collection from observations and measurements at the checkpoints for each cut slope surveyed (modified from CitationGullà & Matano, 1997).
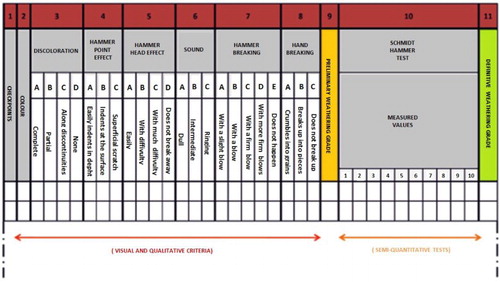
In Phase 4, in order to characterize the weathering grade at micro-scale, seven representative samples of weathered granitoid rocks were collected and thin sectioned for petrographic analyses. The weathering grade in the thin sections was determined by the micropetrographic index suggested by CitationIrfan and Dearman (1978). The authors assumed that the microscopic determinations include the percentage of altered and unaltered minerals, microcracks, and voids.
In Phase 5, the overall analysis of the data, managed within a geographic information system, allowed the preparation of the weathering grade map following the procedure suggested by CitationBorrelli et al. (in press).
4. Results
4.1. Geomorphology and geotectonic
The morphology of the study area is very complex and strongly controlled by tectonics (CitationMolin et al., 2004). Geomorphic evidence of Quaternary fault activity in the area includes features preserved in the landscape as the result of surface deformation and subsequent erosional or depositional processes. Geomorphic features include fault scarps, trapezoidal facets, straight ridges, saddles and aligned saddles, offset or deflected drainage, enclosed depressions, side-hill benches, and linear drainage or troughs.
From 800 to 1300 m above sea level (), the area mostly consists of mountain ridges and mountain valleys (CitationLuca, Robustelli, Conforti, & Fabbricatore, 2011). Three main morphological units can be distinguished: summit surfaces, slopes, and valleys. The summit surfaces have generally rounded morphology and represent the remnants of an ancient low relief landscape almost totally removed by erosion. The slopes are characterized by high gradient values (ranging from 20° to 60°, ) and deep incisions down to the granitoid basement, where there is evidence of widespread erosion and mass wasting. The valleys are mostly characterized by sedimentation (e.g. alluvial deposits related to the Arvo and Neto rivers), with local alternating erosional processes.
From a geological perspective, the Palaeozoic crystalline lithologies outcropping in the study area (for more than 95%) are represented by granitoid rocks belonging to the Sila Unit (CitationMessina et al., 1994) (). Based on field observations and petrographic evidence, granitoid rocks are mainly represented by granite and granodiorite; locally, they are intruded by pegmatite and aplite dikes. Mineralogy of granitoid rocks includes K-feldspar, quartz, plagioclase, biotite, chlorite, and muscovite. These rocks are coarse-grained and white in colour when they are unaltered; pink or reddish when intensely weathered.
Figure 5. Geo-structural map of the study area: (a) panoramic view of the San Giovanni in Fiore town; (b) Upper Pliocene–Middle Pleistocene clastic deposits; (c) granitoid rocks (Palaeozoic). Notes: (1) alluvial deposits; (2) Upper Pliocene–Middle Pleistocene alternating sands and conglomerates; (3) granitoid rocks with mainly granitoid composition (Palaeozoic); (4) normal fault; (5) left-lateral transcurrent fault reactivated as normal fault; (6) fault with undetermined kinematics; (7) low-angle thrust.
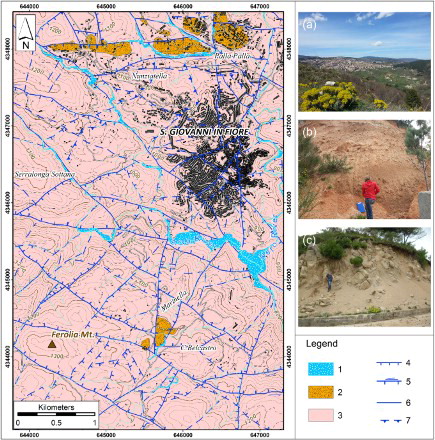
In the northern portion of the study area (), unconformably overlying granitoid rocks rest the Upper Pliocene–Middle Pleistocene clastic deposits including brown-reddish interstratified conglomerates and sands; the succession is related to the proximal facies of the Pliocene-Quaternary Crotone Basin fill (CitationZecchin et al., 2012). Finally, Holocene alluvial deposits are present in the study area along the main rivers ().
From a tectonic perspective, the three main tectonic structures exhibit N–S, NW–SE, and NE–SW trends (). In particular, the N–S trending faults () represent the most recent high-angle extensional faults, having fresh morphological evidence ((a)). This system includes an array of normal faults, predominantly dipping towards the east. However, fault planes show different slip-directions and pitch, sometimes superimposed; the relative chronology of the kinematic indicators suggests that right transcurrent movements and oblique transpressive slip ((b) and (c)) postdate the last normal slip ((d)).
Figure 6. Examples of faults surveyed in the study area at the macro- and meso-scale: (a) panoramic view of the right slope of the Arvo river and some faults related to the main fault systems; (b) N–S transpressive faults; (c) N–S right-lateral transpressive faults; (d) last kinematics on the N–S fault plane in the Pleistocene conglomerates; (e) left-lateral striae on the NW–SE fault plane; (f) ancient overthrust; (g) NW–SE fault zone with associated thick fault gouge.
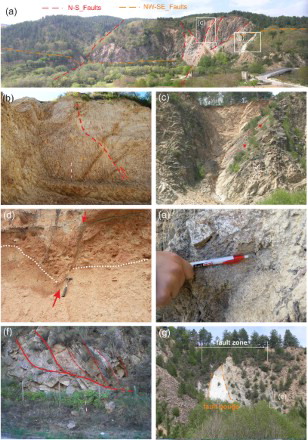
The NW–SE fault system, at macroscale, is well defined and widely diffused (). The system is represented by sub-vertical, NE-dipping fault planes with superimposed kinematic indicators. At the meso-scale, the NW–SE faults are characterized by left-lateral-strike-slip movement ((e)), even if the overprinting of dip-slip pitch suggests their reactivation as normal faults during the last tectonic event.
The NE–SW fault system is also widely represented along the study area (); it shows overprinted kinematic indicators and its inferred chronology suggests a transcurrent or oblique-slip movements superimposed by dip-slip normal kinematics.
The compressive tectonic structures (), poorly evident morphologically, have been surveyed only at the meso-scale. The planes with steeper inclination and oblique pitch are related to the transpressional deformation of the area (cut system), while the subhorizontal planes are related to the more ancient deformation phase, responsible for the development of duplex thrust stack structures ((f)).
Thick argillified fault gauges are sometimes present along the major fault planes ((g)).
4.2. Weathering features
The map of the weathering grade of granitoid rocks () allows a general comprehensive overview of the effects, intensity and distribution of weathering classes strongly controlled by the main fault systems. With regard to the spatial distribution of the weathering grade, four weathering classes (from class VI to class III) were distinguished in the field.
Figure 7. Weathering grade and geotectonic map of the study area. Notes: (1) alluvial deposits; (2) conglomerates and relative covers; (3) class VI; (4); class V; (5) class IV; (6) class III; (7) normal fault; (8) left-lateral transcurrent fault reactivated as normal fault; (9) fault with undetermined kinematics; (10) uncertain thrust; (11) studied weathering profiles; (12) samples collected for the petrographic analyses.
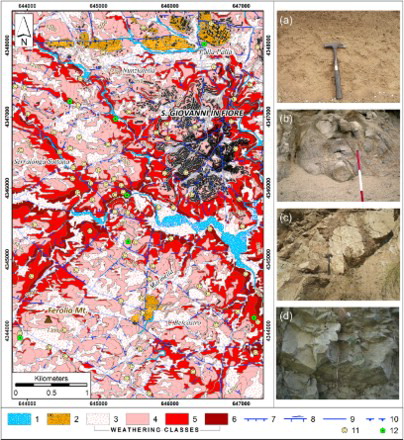
In particular, class VI is present as isolated outcrops both on the top of relief and in the mid-lower parts of the slopes (). It covers about 33% of the surveyed area so divided: (i) residual soils, with not surveyable thickness at the map scale, are mainly present at the summit areas as relicts of palaeosurfaces ((a)); (ii) colluvial soils are mainly present in morphological hollows, where the thickness can reach several metres; and (iii) slope debris, ranging from metres to decametres in thickness, is distributed along the slopes and at the valley bottom.
Class V is located in the upper portion of the slopes, mainly above 1000 m a.s.l. ((b)); it covers about 40% of the surveyed area. The rock masses are completely weathered (more than 70% of the outcrop); limited and isolated rock mass volumes can be constituted by highly weathered rock or residual soil (respectively, classes IV and VI).
Class IV outcrops mainly along the middle portions of the slopes and along the lower portions of the minor stream incisions ((c)); it covers about 22% of the surveyed area. The rock masses are highly weathered (more than 70% of the outcrop); limited and isolated rock mass volumes can be constituted by moderately or completely weathered rock (respectively, classes III and V).
Class III outcrops predominantly both along the lower portions of the slopes and the major stream incisions (e.g. Arvo river and tributary streams) ((d)); it covers about 5% of the surveyed area. The rock masses are moderately weathered (more than 70% of the outcrop); limited and isolated rock mass volumes can be constituted by highly or slightly weathered rock (respectively, classes IV and II).
The weathering profiles were reconstructed along the main cut slopes observed and are shown in . Generally, the studied profiles ((d)–(g)) are formed by rocky masses varying from moderately weathered rocks (along the cut slopes of the stream incisions 783–930 m a.s.l.) to completely weathered rocks (along the cut slopes of the highest reliefs, above 1000 m a.s.l.). Corestones of classes III and IV, outcropping both on the ground surface and on the cut slopes immersed in class V, are common in the upper portions of the weathering profile (above 1100 m a.s.l) ((a) and 8(b)). Residual soils (class VI) are locally observed at the highest points and are a few centimetres thick. Colluvial and detritical-colluvial soils (class VI) are widespread, random, having variable thickness from metres to decametres, and are related to active morphodynamic processes.
Figure 8. Schematic weathering profile reconstructed by the cut slopes survey: (a and b) corestones outcropping on the ground surface near Mt. Ferolia and near Marinella area, above 1100 m a.s.l.; (c) examples of aplitic dike of class III immersed in class V, generally widespread above 1000 m a.s.l.; (d) typical features of the cut slopes observed between 1360 and 1050 m a.s.l; (e–g) typical features of the cut slopes observed (e) from 1050 to 1000 m a.s.l, (f) from 1000 to 930 m a.s.l, (g) from 930 to 783 m a.s.l.
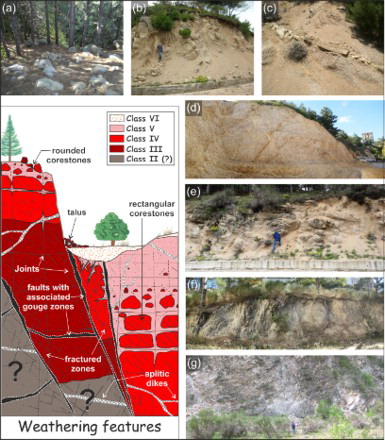
Faults and fractured zones are commonly present on the cut slopes in which sharp contacts between different weathering classes are observed. Indeed, the main faults are characterized by thick fault gauge, made of completely weathered granitoids with soil-like behaviour ((g)).
The combination of tectonic and climate variation (between the Late Miocene and Pleistocene) plays an important role in the development of granitoid weathering profiles of the area (CitationBorrelli et al., 2014; CitationCritelli et al., 1991; CitationGuzzetta, 1974; CitationIetto & Ietto, 2004; CitationMatano & Di Nocera, 1999; CitationMongelli, Cullers, Dinelli, & Rottura, 1998). In particular, the typical weathering profile is generally simple (CitationBrand & Phillipson, 1985) and characterized by a progressive gradual transition from moderately weathered rock (class III) to completely weathered rock (class V), with weathered horizons locally displaced by tectonism (see weathering features in ). Moreover, according to different authors (CitationBorrelli et al., 2014; CitationMatano & Di Nocera, 1999; CitationScarciglia, in press; CitationScarciglia et al., 2005), the weathered rock masses have been estimated to be at least 60 m in thickness.
4.3. Petrographic assessment of weathered rock samples
The granitoid rocks have a subhedral granular texture; they mainly consist of K-feldspar, plagioclase, quartz, biotite, and minor constituents of chlorite, muscovite with accessory epidote, apatite, and titanite.
The micropetrographic index Ip (CitationIrfan & Dearman, 1978) ranges from 0.6 to 7.9 related to different weathered stages. In particular, the lowest Ip values are typical of completely weathered rocks (class V) while the highest are related to moderately weathered rocks (class III). The transition from class III to class V () consists of: (i) the sericitization of plagioclase from core to rim (for the plagioclase with an anorthitic composition) and from rim to core (for the plagioclase with an albitic composition) and, along preferential lines and pitting on mineral surfaces; (ii) the neoformation of unidentified clay minerals within both plagioclase and K-feldspar, and partial clay neoformation and exfoliation of biotite along rims and lamellae; and (iii) the transformation of biotite and secondary (deuteric) chlorite to fine-grained micaceous products, clay minerals and Fe-oxides are frequently observed and cause the expansion along cleavage planes, grain boundaries, and partial decomposition. Generally, quartz remains unaffected by chemical alteration or shows minor modifications.
Figure 9. Photomicrographs of weathering stages for the studied granitoid: (a and b) moderately weathered sample (class III); (c and d) highly weathered sample (class IV); (e and f) completely weathered sample (class V). K-feld, K-feldspar; Plg, plagioclase; Chl, chlorite; Bt, biotite; Qtz, quartz; Cm, clay minerals; Fe ox, Fe oxides.
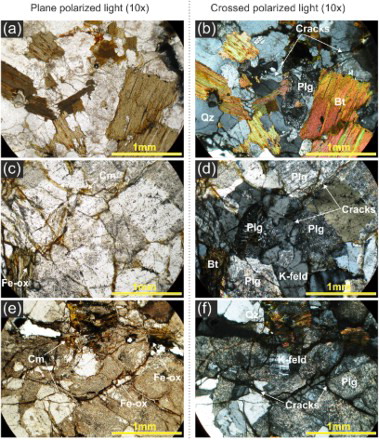
Starting from class III, some intragranular and intergranular small cracks, which tend to expand and propagate towards the class V, can be observed (). In the advanced stage of weathering (completely weathered samples), plagioclases are completely decomposed, and potash feldspars are gritty as a result of partial alteration ((e) and 9(f)); the structure is strongly weakened due to opening up of grain boundaries and intense micro-fracturing, but the fabric is still intact ((f)).
5. Conclusions
This paper presents a geotectonic and weathering grade map of the San Giovanni area (Sila Massif, Calabria). The map provides, at detailed scale, both a general and comprehensive overview of effects, intensity, and spatial distribution of the weathering profile and the effect of the constraint generated by tectonics.
The proposed methodology represents a typical example of interdisciplinary study based on geo-structural investigations, weathering grade surveys, and petrographic analyses.
The weathering grade survey is focused on geological observations integrated with the assessment of engineering properties of the rocks by simple qualitative and semi-quantitative index tests. Field surveys show that the granitoid rocks outcropping in the study area experienced intense weathering action. The results of this process are evident in the diffusion of highly to completely weathered rocks, while, moderately weathered rocks outcrop only along major stream incisions. Moreover, residual soils are rarely observed, while colluvial and detrital soils are widespread due to active morphodynamic processes.
A useful contribution to the weathering grade surveys is represented by the analyses of the cut slopes, which presented information on the typical features of the weathering profiles, such as the geometry and thickness of the weathering classes. Consequently, the weathering profiles have been estimated to be at least 60 m in thickness. Despite chemical weathering being the dominant process, fracturing and faulting are also key factors both in the development of deep weathering and in the spatial geometric relationship between the weathered horizons.
The mechanical changes observed from moderately to completely weathered rocks are generally associated with both an increase of weathering products at micro-scale (e.g. sericite, clay minerals, Fe-oxides, etc.), and the progressive formation of voids and fractures.
The obtained results summarized in the Main Map highlight the validity of the proposed approach, demonstrating the reliability of rapid techniques in the identification of homogenous areas – affected by the same weathering grade (i.e. weathering class) – with a comparable mechanical behaviour.
This kind of thematic map represents a useful tool for territorial planning and engineering–geological and environmental purposes, providing a useful contribution for mass wasting and soil erosion hazard assessment.
Software
The weathering grade map and related layout (, , , and ) were carried out using ESRI ArcGIS 10.0. Corel Draw X6 was used for compiling , , , , and .
Acknowledgements
This work was carried out under the Commessa TA.P05.012 ‘Tipizzazione di eventi naturali e antropici ad elevato impatto sociale ed economico’ of the CNR – Department ‘Scienze del sistema Terra e Tecnologie per l'Ambiente’. The authors thank Dr John Abraham, Dr Massimo Conforti, and Dr Francesco Muto for providing constructive comments, which have contributed to the improvement of the manuscript and map.
References
- Amodio-Morelli, L., Bonardi, G., Colonna, V., Dietrich, D., Giunta, G., Ippolito, F., … Zuppetta, A. (1976). The Calabrian–Peloritan Arc in the Apennine-Maghrebide orogen. Memorie della Società Geologica Italiana, 17, 1–60.
- Barone, M., Dominici, R., Muto, F., & Critelli, S. (2008). Detrital modes in a late Miocene wedge-top basin, northeastern Calabria, Italy: Compositional record of wedge-top partitioning. Journal of Sedimentary Research, 78, 693–711. doi: 10.2110/jsr.2008.071
- Baynes, F. J., Dearman, W. R., & Irfan, T. Y. (1978). Practical assessment of grade in a weathered granite. Bulletin of the International Association of Engineering Geology, 18, 101–109. doi: 10.1007/BF02635355
- Borrelli, L., Cofone, G., Critelli, S., Greco, S., & Gulla’, G. (2012). Carta del grado di alterazione e categorie di frane nell'alta valle del Fiume Trionto (Calabria, Italia). Rendiconti online della Società Geologica Italiana, 21, 537–539.
- Borrelli, L., Cofone, G., & Gulla’, G. (2012). Procedura speditiva per la redazione di una carta del grado di alterazione a scala regionale. Rendiconti online della Società Geologica Italiana, 21, 528–530.
- Borrelli, L., Critelli, S., Gullà, G., & Muto, F. (in press). Weathering grade and geotectonics of the western-central Mucone River basin (Calabria, Italy). Journal of Maps. doi:10.1080/17445647.2014.933719
- Borrelli, L., Greco, R., & Gullà, G. (2007). Weathering grade of rock masses as a predisposing factor to slope instabilities: Reconnaissance and control procedures. Geomorphology, 87, 158–175. doi: 10.1016/j.geomorph.2006.03.031
- Borrelli, L., Perri, F., Critelli, S., & Gullà, G. (2012). Minero-petrographical features of weathering profiles in Calabria, southern Italy. Catena, 92, 196–207. doi: 10.1016/j.catena.2012.01.003
- Borrelli, L., Perri, F., Critelli, S., & Gullà, G. (2014). Characterization of granitoid and gneissic weathering profiles of the Mucone River basin (Calabria, southern Italy). Catena, 113, 325–340. doi: 10.1016/j.catena.2013.08.014
- Brand, E. W., & Phillipson, H. B. (1985). Sampling and testing of residual soils: A review of international practice. Technical Committee on Sampling and Testing of Residual Soils, International Society for Soil Mechanics and Foundation Engineering. Hong Kong: Scorpion Press.
- Calcaterra, D., & Parise, M. (2010). Weathering in the crystalline rocks of Calabria, Italy, and relationships to landslides. In D. Calcaterra & M. Parise (Eds.), Weathering as a predisposing factor to slope movements (pp. 105–130). London: Geological Society.
- Cascini, L., Critelli, S., Di Nocera, S., Gullà, G., & Matano, F. (1992). Grado di alterazione e franosità negli gneiss del Massiccio silano: L'area di S. Pietro in Guarano (CS). Geologia Applicata e Idrogeologia, 27, 49–76.
- Cascini, L., & Gullà, G. (1993). Caratterizzazione fisico-meccanica dei terreni prodotti dall'alterazione di rocce gneissiche. Rivista Italiana di Geotecnica, 2, 125–147.
- Ceryan, S., Tudes, S., & Ceryan, N. (2008). Influence of weathering on the engineering properties of Harsit granitic rocks (NE Turkey). Bulletin of Engineering Geology and Environment, 67, 97–104. doi: 10.1007/s10064-007-0115-0
- Chigira, M. (2001). Micro-sheeting of granite and its relationship with landsliding specifically after the heavy rainstorm in June 1999, Hiroshima Prefecture, Japan. Engineering Geology, 59, 219–231. doi: 10.1016/S0013-7952(00)00075-2
- Chigira, M., Mohamad, Z., Sian, L. C., & Komoo, I. (2011). Landslides in weathered granitic rocks in Japan and Malaysia. Bulletin of the Geological Society of Malaysia, 57, 1–6.
- Corbi, F., Fubelli, G., Lucà, F., Muto, F., Pelle, T., Robustelli, G., … Dramis, F. (2009). Vertical movements in the Ionian margin of the Sila Massif (Calabria, Italy). Bollettino Società Geologica Italiana, 128, 731–738.
- Critelli, S., Di Nocera, S., & Le Pera, E. (1991). Approccio metodologico per la valutazione petrografica del grado di alterazione degli gneiss del Massiccio Silano (Calabria settentrionale). Geologia Applicata e Idrogeologia, 26, 41–70.
- Critelli, S., Muto, F., Tripodi, V., & Perri, F. (2013). Link between thrust tectonics and sedimentation processes of stratigraphic sequences from the southern Apennines foreland basin system, Italy. Rendiconti Online della Società Geologica Italiana, 25, 21–42.
- Dearman, W. R., & Matula, M. (1976). Environmental aspects of engineering geological mapping. Bulletin of the International Association of Engineering Geology, 13, 141–146. doi: 10.1007/BF02634779
- Di Nocera, S., & Matano, F. (2002). Weathering surveys in geomorphology: Mapping examples from Sila Massif (Calabria, Southern Italy). In R. J. Allison (Ed.), Applied geomorphology: Theory and practice (pp. 205–225). Chichester: Wiley.
- Geological Society Engineering Group Working Party. (1995). The description and classification of weathered rocks for engineering purposes. Quarterly Journal of Engineering Geology and Hydrogeology, 28, 207–242. doi: 10.1144/GSL.QJEGH.1995.028.P3.02
- Geotechnical Control Office. (1988). Guide to rock and soil descriptions. Hong Kong: Civil Engineering Services Department.
- Gullà, G., Aceto, L., & Borrelli, L. (2012). Terreni di alterazione da rocce cristalline. Rendiconti online della Società Geologica Italiana, 21, 548–550.
- Gullà, G., & Matano, F. (1997). Surveys of weathering profile on gneiss cutslopes in Northern Calabria, Italy. Proceedings of the international symposium on engineering geology and the environment, IAEG, Athens, pp. 133–138.
- Guzzetta, G. (1974). Ancient tropical weathering in Calabria. Nature, 251, 302–303. doi: 10.1038/251302a0
- Ietto, A., & Ietto, F. (2004). Age and history of the weathering of granitoids in southern Calabria. Geografia Fisica e Dinamica Quaternaria, 27, 37–45.
- Ietto, F., Perri, F., & Fortunato, G. (in press). Lateral spreading phenomena and weathering processes from the Tropea area (Calabria, southern Italy). Environmental Earth Sciences. doi:10.1007/s12665-014-3745-0
- International Association of Engineering Geology. (1976). Engineering geological maps: A guide to their preparation. Paris: Unesco Press.
- International Association of Engineering Geology. (1981). Rock and soil description for engineering geological mapping. Bulletin of the International Association of Engineering Geology, 24, 235–274. doi: 10.1007/BF02595273
- Irfan, T. Y. (1996). Mineralogy, fabric properties and classification of weathered granites in Hong Kong. Quarterly Journal of Engineering Geology and Hydrogeology, 29, 5–35. doi: 10.1144/GSL.QJEGH.1996.029.P1.02
- Irfan, T. Y., & Dearman, W. R. (1978). Engineering classification and index properties of a weathered granite. Bulletin of the International Association of Engineering Geology, 17, 79–90. doi: 10.1007/BF02634696
- Lacerda, W. A. (2007). Landslide initiation in saprolite and colluvium in southern Brazil: Field and laboratory observations. Geomorphology, 87, 104–119. doi: 10.1016/j.geomorph.2006.03.037
- Le Pera, E., Critelli, S., & Sorriso-Valvo, M. (2001). Weathering of gneiss in Calabria, Southern Italy. Catena, 42, 1–15. doi: 10.1016/S0341-8162(00)00117-X
- Le Pera, E., & Sorriso-Valvo, M. (2000a). Weathering and morphogenesis in a Mediterranean climate, Calabria, Italy. Geomorphology, 34, 251–270. doi: 10.1016/S0169-555X(00)00012-X
- Le Pera, E., & Sorriso-Valvo, M. (2000b). Weathering, erosion and sediment composition in a high-gradient river, Calabria, Italy. Earth Surface Processes and Landforms, 25, 277–292. doi: 10.1002/(SICI)1096-9837(200003)25:3<277::AID-ESP79>3.0.CO;2-Z
- Luca, F., Robustelli, G., Conforti, M., & Fabbricatore, D. (2011). Geomorphological map of the Crotone province (Calabria, South Italy). Journal of Maps, 7, 375–390. doi: 10.4113/jom.2011.1190
- Maharaj, R. J. (1995). Engineering-geological mapping of tropical soils for land-use planning and geotechnical purposes: A case study from Jamaica, West Indies. Engineering Geology, 40, 243–286. doi: 10.1016/0013-7952(95)00054-2
- Malomo, S., Olorunniwo, M. A., & Ogunsanwo, O. (1983). Engineering geological mapping in terrains of tropical weathering – An example from Abuja, Nigeria. Engineering Geology, 19, 133–148. doi: 10.1016/0013-7952(83)90031-5
- Matano, F., & Di Nocera, S. (1999). Weathering patterns in the Sila Massif (northern Calabria, Italy). Italian Journal of Quaternary Sciences, 12, 141–148.
- Messina, A., Russo, S., Borghi, A., Colonna, V., Compagnoni, R., Caggianelli, A., … Piccarreta, G. (1994). Il Massiccio della Sila Settore settentrionale dell'Arco Calabro-Peloritano. Bollettino della Società Geologica Italiana, 113, 539–586.
- Molin, P., Pazzaglia, F. J., & Dramis, F. (2004). Geomorphic expression of active tectonics in a rapidly deforming forearc, Sila Massif, Calabria, southern Italy. American Journal of Science, 304, 559–589. doi: 10.2475/ajs.304.7.559
- Mongelli, G., Cullers, R. L., Dinelli, E., & Rottura, A. (1998). Elemental mobility during the weathering of exposed lower crust: The kinzigitic paragneisses from the Serre, Calabria, Southern Italy. Terra Nova, 10, 190–195. doi: 10.1046/j.1365-3121.1998.104188.x
- Muto, F., Spina, V., Tripodi, V., & Critelli, S. (2014). Neogene tectonostratigraphic evolution of allochthonous terranes in the eastern Calabrian Foreland (Southern Italy). Italian Journal of Geosciences, 133, 455–473. doi: 10.3301/IJG.2014.23
- Perri, F., Scarciglia, F., Apollaro, C., & Marini, L. (in press). Characterization of granitoid profiles in the Sila Massif (Calabria, southern Italy) and reconstruction of weathering processes by mineralogy, chemistry, and reaction path modelling. Journal of Soils and Sediments. doi:10.1007/s11368-014-0856-x
- Regmi, A. D., Yoshida, K., Dhital, M. R., & Devkota, K. (2013). Effect of rock weathering, clay mineralogy, and geological structures in the formation of large landslide, a case study from Dumre Besei landslide, Lesser Himalaya Nepal. Landslides, 10, 1–13. doi: 10.1007/s10346-011-0311-7
- Regmi, A. D., Yoshida, K., Dhital, M. R., & Pradhan, B. (2014). Weathering and mineralogical variation in gneissic rocks and their effect in Sangrumba Landslide, East Nepal. Environmental Earth Sciences, 71, 2711–2727. doi: 10.1007/s12665-013-2649-8
- Scarciglia, F. (in press). Weathering and exhumation history of the Sila Massif upland plateaus, southern Italy: A geomorphological and pedological perspective. Journal of Soils and Sediments. doi:10.1007/s11368-014-0923-3
- Scarciglia, F., Le Pera, E., & Critelli, S. (2005). Weathering and pedogenesis in the Sila Grande Massif (Calabria, South Italy): From field scale to micromorphology. Catena, 61, 1–29. doi: 10.1016/j.catena.2005.02.001
- Scarciglia, F., Le Pera, E., & Critelli, S. (2007). The onset of sedimentary cycle in a mid-latitude upland environment: Weathering, pedogenesis and geomorphic processes on plutonic rocks (Sila Massif, Calabria). In J. Arribas, S. Critelli, & M. Johnsson (Eds.), Sedimentary provenance: Petrographic and geochemical perspectives (pp. 149–166). Boulder: The Geological Society of America.
- Scarciglia, F., Le Pera, E., Vecchio, G., & Critelli, S. (2005). The interplay of geomorphic processes and soil development in an upland environment, Calabria, South Italy. Geomorphology, 64, 1–23. doi: 10.1016/j.geomorph.2004.04.005
- Spina, V., Tondi, E., & Mazzoli, S. (2011). Complex basin development in a wrench-dominated back-arc area: Tectonic evolution of the Crati Basin, Calabria, Italy. Journal of Geodynamics, 51, 90–109. doi: 10.1016/j.jog.2010.05.003
- Tansi, C., Muto, F., Critelli, S., & Iovine, G. (2007). Neogene-Quaternary strike-slip tectonics in the central Calabrian Arc (Southern Italy). Journal of Geodynamics, 43, 393–414. doi: 10.1016/j.jog.2006.10.006
- Turkington, A. V., Phillips, J. D., & Campbell, S. W. (2005). Weathering and landscape evolution. Geomorphology, 67, 1–6. doi: 10.1016/j.geomorph.2004.08.013
- Van Dijk, J. P., Bello, M., Brancaleoni, G. P., Cantarella, G., Costa, V., Frixa, A., … Zerilli, A. (2000). A regional structural model for the northern sector of the Calabria Arc (Southern Italy). Tectonophysics, 324, 267–320. doi: 10.1016/S0040-1951(00)00139-6
- Zecchin, M., Caffau, M., Civile, D., Critelli, S., Di Stefano, A., Maniscalco, R., … Roda, C. (2012). The Plio-Pleistocene evolution of the crotone basin (Southern Italy): Interplay between sedimentation, tectonics and eustasy in the frame of Calabrian Arc migration. Earth-Science Reviews, 115, 273–303. doi: 10.1016/j.earscirev.2012.10.005
- Zecchin, M., Caffau, M., Di Stefano, A., Maniscalco, R., Lenaz, D., Civile, D., … Critelli, S. (2013). The Messinian succession of the Crotone basin (southern Italy) II: Facies architecture and stratal surfaces across the Miocene–Pliocene boundary. Marine and Petroleum Geology, 48, 474–492. doi: 10.1016/j.marpetgeo.2013.08.013
- Zecchin, M., Civile, D., Caffau, M., Muto, F., Di Stefano, A., Maniscalco, R., & Critelli, S. (2013). The Messinian succession of the Crotone basin (southern Italy) I: Stratigraphic architecture reconstructed by seismic and well data. Marine and Petroleum Geology, 48, 455–473. doi: 10.1016/j.marpetgeo.2013.08.014