Abstract
The geomorphology of a coastal landscape may reveal an indication of past shoreline configurations. The spatial arrangement of the preserved morphologies may further contain indications on the importance of the different key parameters influencing shoreline evolution over millennial timescales, such as palaeo sea-level position, longshore currents, energy gradients, and sediment supply. This paper presents the results and observations of a surveying and mapping study conducted for the island of Samsø at a scale of 1:75,000. The objective of the work is to support the interpretation of core sample data and to extract information on the factors determining the morpho-sedimentary development of moraine-embedded coastal lagoon systems during the Holocene. The map is based on an airborne LiDAR-derived high-resolution digital terrain model that is combined with spatial information on bathymetry, surface geology and other features from archived geodata and maps.
1. Introduction
The evolution of the coastal landscape in central Denmark is, in its broad characteristics, determined by three key variables: fetch, inherited topography, and centennial to millennial-scale sea-level changes (CitationSchou, 1945). The landscape character of the area is profoundly determined by the Pleistocene glaciations that moulded large bodies of unconsolidated sedimentary material into a varied terrain (CitationHoumark-Nielsen, 1987). The marine ingression into the southern Kattegat took place in the mid-Atlantic period (8–7 kyr BP; CitationBjørnsen, Clemmensen, Murray, & Pedersen, 2008; CitationKrog, 1979; CitationPetersen, 1978) when an initial shoreline of islands, lagoons, and fjords evolved. With the ingression, the morphological work of waves, tides, and currents commenced and led to the formation of coastal cliffs, barriers, and beaches. The initially high rates of relative sea-level (RSL) rise culminated in the establishment of a highstand period with minor sea-level fluctuations lasting between approximately 7.3 and 3.7 kyr BP (CitationChristensen, 1995; CitationClemmensen, Nielsen, Bendixen, & Murray, 2012; CitationHede et al., in press; CitationIversen, 1937). Since then, RSL in the area is characterized by a falling trend, due to the continuous glacial isostatic adjustment (GIA) of SW Scandinavia outpacing the declining rates of post-glacial absolute sea-level (ASL) rise in the mid-Atlantic period (CitationFleming et al., 1998; CitationLambeck, Smither, & Johnston, 1998; CitationPåsse & Anderson, 2005; CitationHede et al., in press). This determines that most of central Denmark is characterized by spatially highly variable rates of RSL development since the mid-Holocene (CitationHansen, Aagaard, & Binderup, 2011; CitationMertz, 1924). However, regarding the evolution and present configuration of the coastal landscape, CitationSchou (1949) argues that ‘the different types of Danish moraine coasts have to be interpreted as stages in the development of shoreline simplification and not as coasts of emergence and submergence’ (p. 358), since the erosion of the unconsolidated moraine material and the accumulation in coastal landforms proceeded much faster than the rates of vertical land movement or RSL change. Fetch and wave exposure thus yields first-order controls on the morphological development of moraine coasts over centennial to millennial time scales (CitationCarter & Orford, 1988; CitationSchou, 1945, Citation1949). Both fetch and wave energy exposure varies considerably over short distances due to the complex land–sea distribution of central Denmark ((a); CitationLundqvist, Jansen, Balstroem, & Christiansen, 2006). Favourable conditions for shoreline progradation only exist in areas with a sustained net sediment surplus (CitationBjørnsen et al., 2008; CitationClemmensen, Richardt, & Andersen, 2001; CitationHede, Bendixen, Clemmensen, Kroon, & Nielsen, 2013; CitationKabuth & Kroon, 2014). Most of the central Danish coast is characterized by stability or minor rates of change (CitationKabuth, Kroon, & Petersen, 2013). A retreating mode in shoreline development commonly finds its morphological expression in the presence of moraine cliffs, while elevated strandplains are evidence of a progradational mode in shoreline development over an extended period of time (cf CitationTamura, 2012). A wide range of spatial arrangements of interlocking coastal landforms is possible between these end-member scenarios.
Figure 1. (a) Maximum fetch (CitationLundqvist et al., 2006), ring spacing: 25 km, (b) observed wind data (annual averages, 1989–1998; CitationCappelen & Jørgensen, 1999) and modelled wave parameters around Samsø, and (c) hypothesized evolutionary pathways of the asymmetrical Norby Hede beach-ridge system since the mid-Holocene transgression, based on the LiDAR DTM, geodata, and absolute chronology from CitationHede et al. (in press) and CitationSander et al. (in press).
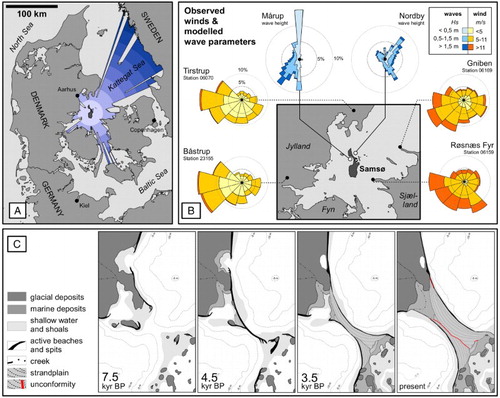
The island of Samsø, Denmark ((a) and 1(b)), was chosen as a case example for the mapping of coastal geomorphological features indicative of past shoreline configurations and process dynamics. The spatial distribution and orientation of, for example, elevated strandlines or inactive moraine cliffs facilitate an intuitive reconstruction of past stages in the evolution of the coastal landscape over the mid and late Holocene ((c)). The reconstruction of local shoreline evolution is commonly based on the analysis of historical maps as an important source of spatial data (CitationFruergaard, Andersen, Johannessen, Nielsen, & Pejrup, 2013; CitationSchou, 1945; CitationStéphan, Suanez, & Fichaut, 2012), whereas the presented map is primarily a Geographical Information System (GIS)-based analysis and processing of digital terrain model (DTM) data of the present topography of the terrestrial and marine environments. Archived maps and orthophotos are used to additionally support the interpretation. Detailed ground-truthing was conducted during field surveys at key localities.
The purpose of this study is to locate adequate on-shore study sites for the detailed investigation of coastal lagoons and beach ridges in order to reconstruct Holocene RSL changes (CitationSander et al., in press; CitationHede et al., in press). The mapping provides crucial information for the strategic preparation of field campaigns as well as for substantiating the interpretation of field data and laboratory results. The input data used for the mapping allow a reproduction of the presented approach for any given site of interest within the area of data coverage (i.e. the Danish national territory) or wherever similar databases are available.
2. Physical characteristics of the field site and the Holocene sea-level development
Two main factors determine the development of Holocene RSL in south-western Scandinavia: (1) post-glacial ASL and (2) GIA. The end of the last Pleistocene glaciation caused a drastic rise in sea level due to the release of large amounts of melt water into the world's oceans (CitationLambeck & Chappell, 2001). At the same time, a slow vertical displacement of the Earth's crust in previously glaciated areas was initiated by the unloading of the weight of the ice-masses with the deglaciation of the continental ice sheets (CitationPåsse & Anderson, 2005). The balance between the rates of ASL change and GIA determines the local development of RSL through time. The southern Kattegat area experienced a period of rapid sea-level rise between 8 and 7 kyr BP (CitationKrog, 1979; CitationMörner, 1976; CitationSander et al., in press) followed by a highstand period in the mid-Holocene (CitationBjørnsen et al., 2008; CitationChristensen, 1995; CitationHede, 2003), when RSL on Samsø was approximately 2.5–3 m higher than present (CitationMertz, 1924). Since then, RSL has been falling at rates comparable to the present rates of vertical isostatic movement (CitationHansen et al., 2011; CitationHede et al., in press).
Samsø is located in the protected inner part of the southern Kattegat Sea. The island formed on a lobate terminal moraine of late Pleistocene age (CitationHoumark-Nielsen, 2011; CitationKjær, Houmark-Nielsen, & Richardt, 2003), and the accessible parent materials for the formation of coastal morphological features are most importantly unsorted tills (diamicton) and proglacial outwash deposits composed of gravels, sands, and mud (CitationHoumark-Nielsen, 1987). The ice-marginal position of Samsø further determines an undulating topography with marked changes in elevation over short distances.
The southern Kattegat Sea has an average present mean tidal range of ∼0.3 m, though strong seasonal deviations in water level occur regularly of the order of less than 1 m due to meteorological conditions (CitationHansen, 1999; CitationKroon, Kabuth, & Westh, 2013; CitationRasmussen, 1968). Predominant winds fluctuate between south-easterly and westerly directions (150–300°) ((b); CitationCappelen & Jørgensen, 1999). Model hindcast data on wave height and direction for two node points close to Samsø’s northern shore indicate a highly uneven exposure to wave energy as a function of fetch ((a and b)). Given the low tidal energy, waves become the most important energy source influencing erosion, sediment transport, and deposition on Samsø (cf CitationBoyd, Dalrymple, & Zaitlin, 1992; CitationDavis & Hayes, 1984).
3. Data
The surface morphology of the terrestrial portion of Samsø was analysed based on high-resolution DTM data obtained from a national airborne Light Detection and Ranging (LiDAR) survey and is available through the Danish Geodata Agency (CitationGeodatastyrelsen, 2014a). The resulting grid has a resolution of 1.6 m with a vertical accuracy of ±0.1 m (CitationRosenkranz & Frederiksen, 2011). Elevations relate to the current Danish ordnance datum DVR90 (Danish Vertical Reference, 1990). The interpretation of the DTM was supported by digital orthophotos (Danmarks Digitale Orthofoto, DDOland; ) and maps to identify surface features (e.g. location of roads, drainage channels, etc.). Information on the topography of the currently submerged areas was retrieved from a digital bathymetrical model (DBM) available for the inner Danish waters from Danmarks Miljøundersøgelser – Areal Informations Systemet (DMU-AIS; Areal Information System of the National Environmental Research Institute of Denmark). The DBM is a compilation of data from different generations of hydrographic surveys available at a 10 m grid resolution. The accuracy of the data is variable, and data quality may deteriorate towards shallower coastal waters (CitationNielsen et al., 2000). Water depths are provided in metres in relation to the outdated ordnance datum, DNN (Dansk Normalnul, Danish ordnance datum). The offset between DVR90 and DNN results from historical land uplift and historical sea-level changes and is negligible on Samsø (−0.013 m; CitationMiljøministeriet, 2005). It is thus disregarded for this study due to the chosen scale of observation as well as due to the resolution and vertical accuracy of the original input data. Information on surface geology is obtained from a digital geological map sheet at a 1:25,000 scale (CitationJakobsen, Hermansen, & Tougaard, 2011), which is interpreted along with the original map and mapping report for Samsø (CitationMadsen, 1897). Additional topographic information was available from digitized historical maps (; CitationGeodatastyrelsen, 2014a). All analyses and the visualization of the geodata are conducted in a GIS (Esri ArcMap 10.1), and coastal landforms are mapped manually and supported by basic digital terrain analysis (e.g. slope).
Table 1. Overview of used geodata.
4. Coastal landforms
4.1. Lagoons
The existence of basins in the Pleistocene moraine topography promotes the formation of coastal lagoons. These are in consequence predominantly encountered on Samsø’s southern island, where more undulating terrain conditions prevail as a result of the location of the southern island on a young terminal moraine, while the northern island is dominated by a basal till and meltwater gravels. Lagoons are here defined as near-coastal basins that received marine sedimentation at some point during the Holocene (cf CitationTagliapietra, Sigovini, & Ghirardini, 2009). Today, only one active lagoon is encountered on Samsø (Stavns Fjord; CitationRasmussen, 1968). On the DTM, inactive (i.e. sediment filled or artificially drained) lagoons can be defined and detected by their relatively flat topography in relation to the surrounding moraine elevations as well as by their low absolute elevation in relation to fossil moraine cliffs (). The inactive lagoons are delimited by barriers towards the open marine environment ((d)), and tidal channel morphologies may occasionally be visible ((d)). Several side basins of Stavns Fjord are artificially drained, and dikes and channels can be detected on the DTM and on the orthophotos. The soils of inactive lagoons are in most cases reclaimed for agriculture, and their original morphology is altered by ploughing and the installation of infrastructure, which renders the definition of exact strandlines within the lagoons impossible.
Figure 2. DTM representations and orthophotos of selected field sites. (a) Prograded beach ridges on the exposed side of cliffed and wave-abraded moraine elevations and the formation of salt marshes on the protected side. (b) Aeolian drapings and small dunes covering the extensive beach-ridge system of central Samsø. (c) Salt marshes and remnants of moraine elevations of two islands within Stavns Fjord. (d) A sub-recent coastal lagoon with tidal channel morphology is delimited by an elongated spit connecting moraine cliffs and elevated shoals to the west, while its eastern boundary is delimited by dikes constructed in AD1877 for reclamation of land.
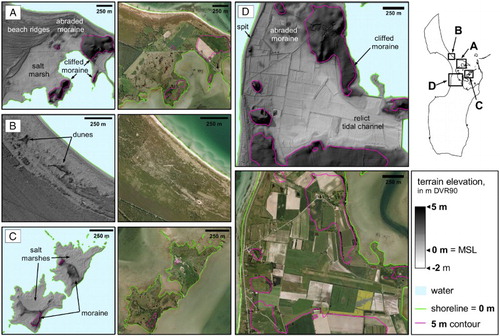
4.2. Beaches and beach-ridge systems
All of the exposed coasts of Samsø are fringed by beaches ((a) and 1(b), (a) and 3(b)). The characteristics of these beaches vary considerably with regard to their width, grain-size composition, and consequently their dip angle and morphology. Most beaches associated with active or sub-recently active cliffs are composed of pebble- and cobble-sized material with minor gravel and sand fractions. In sediment-surplus locations like the major beach-ridge system in central Samsø, mixed-sediment beaches occur with sands and gravels as major components. Here, the shoreline is slightly convex in its course and has been prograding over historical time scales (CitationKabuth et al., 2013). The orientation of an individual beach-ridge crest provides a clear indication of a shoreline position in the past, and the morphology of prograded beach-ridge successions may thus be interpreted as time series of coastal evolution with a relative chronology (CitationTamura, 2012; CitationTaylor & Stone, 1996). Unconformities, in the form of truncation or erosion of older ridges by younger ones with a different orientation, may allude to a punctuation of the sedimentary record caused by changes in sediment budget, hydrodynamic conditions, or sea-level forcing (CitationClemmensen, Murray, & Nielsen, 2012; CitationTaylor & Stone, 1996). While the mapping of beach ridges in the LiDAR DTM can be a straight-forward operation, aeolian reworking or deposition of wind-blown material may interfere with a complete and accurate reconstruction of marine deposits and morphologies. This applies particularly where single ridges or smaller beach-ridge systems occur. Individual ridges (spits) are found in several locations within the strandplain and appear to be associated with the erosion of local sediment sources, and thus leave an indication of the direction of longshore transport at the time of their formation (CitationCarter & Orford, 1988; CitationStéphan et al., 2012).
Figure 3. (a) Slim beach and active cliff retreat on Samsø’s northern shore; (b) vegetated moraine bluff with pebbly beach ridges, Ballebjerg, Northern Samsø; (c) seaweed-covered boulders at −2 m water depth ∼300 m offshore from Samsø’s western shore; and (d) boulders on a high marsh covering abraded moraine material at Hesselholm Made, central Samsø.
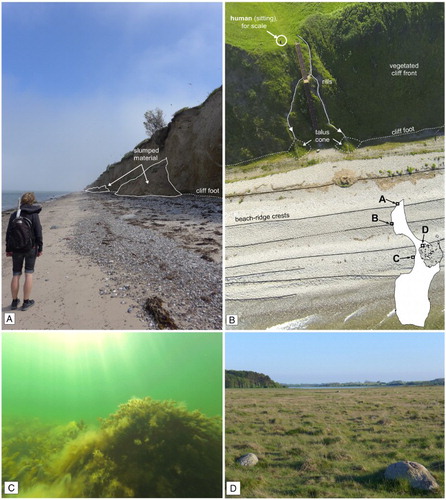
4.3. Moraine cliffs
Moraine cliffs form by wave erosion during high water events with waves, and active cliffs are usually associated with slim beaches or boulder lags ((a); cf CitationGreenwood & Orford, 2008). Modern cliffs are primarily found on the exposed stretches of Samsø’s shoreline and the existence of cliff sections located in protected positions or enclosed by elevated marine deposits thus suggests a higher exposure in the past ((b)). Many sub-recently active cliffs are characterized by gravitational mass movements in the form of slumping or sliding and occur locally in combination with rill erosion (cf CitationSchou, 1949; (a)). A sharp transition between the cliff foot and the foreshore is not preserved at the surface in fossil cliff sections, but can by approximation be identified by the marked change in slope between the cliff and the foreshore ((a) and (d)). Cliffs indicate periods of coastal retreat of unknown duration or rate and are found between the present high-water line and the mid-Holocene maximum RSL (CitationMertz, 1924).
4.4. Abraded moraine and boulders
Distinct areas with (abraded) moraine topography are found both onshore and offshore Samsø. In shallow water, these are characterized by deviations of the bathymetric contour lines and the local presence of boulder reefs ((c), Main Map). The boulder lags are evidence for the nearly complete erosion of smaller moraine elevations resulting from the work of waves. On land, the abraded moraine surfaces can be identified as small, flat to convex elevations ((a, c, d) and 3(d)). The flooding and wave abrasion of these features is supported by their position below the elevation of the foot of fossil cliffs and the local height of beach-ridge crests. The positions of individual boulders or boulder assemblages were included in (historical) topographical maps (LMBB from 1863, DTK from 1961; cf ). The local presence of boulders correlated well with other indications of shoreline retreat, though the exact location and presence of boulders has to be treated with caution, as the original mapping is likely to be incomplete and boulders cannot be detected independently from the available data sets.
4.5. Aeolian landforms
Aeolian sandsheets are difficult to define or to delimit based on the DTM alone. Some features covering the beach-ridge systems can be identified as aeolian landforms ((b)), while areas covered by thicker wind-blown sandsheets identified in corings (up to >1 m thick; CitationSander et al., in press) do not become apparent in the LiDAR DTM. Difficulties arise from the alteration of the surface morphology by agriculture and other anthropogenic use. The geological map sheet for Samsø indicates a few smaller areas of aeolian deposition in proximity to the modern shoreline (Main Map: surface geology). Aeolian landforms are not indicated in the presented map, due to the mapping uncertainties and their supposedly minor importance as a process of shoreline evolution on Samsø at the provided scale of observation.
5. Conclusions
The record of coastal landforms preserved on Samsø can, in a straightforward and problem-oriented fashion, be used to acquire knowledge about the palaeogeographical configurations of the coastal landscape as well as the changes that occurred during the area's marine stage. The extensive body of available geodata can be put to use for this purpose in environments where Holocene coastal and marine deposits exist. Most preserved landforms can be mapped based on the LiDAR DTM alone, which is the major source of information for this study and can be employed at a high degree of confidence. Similarly to glacial landforms (CitationLivingstone et al., 2013; CitationSmith, Rose, & Booth, 2006), coastal geomorphological features such as cliffs, spits, or beach ridges preserve a directional component and thus contain information about exposure and the direction of sediment transport (CitationStéphan et al., 2012; CitationTamura, 2012). Substantial information about the subtidal environment is more difficult to obtain due to the lower quality of the base data. Modern intertidal and terrestrial analogues are however useful to support an adequate interpretation and can be supported by the high-resolution DTM. Additional data from orthophotos and maps have been shown to be useful to identify, for example, areas of anthropogenic alteration, infrastructure, vegetation patterns, or rates of shoreline dynamics and thus helped to improve the quality of the landform interpretation.
Software
All data were available as ArcGIS layer files from an internal faculty server and were thus for practical reasons processed in Esri ArcMap 10.1. All used tools and methods are generic, which determine a high degree of reproducibility with other GIS applications. Individual data frames were clipped and cropped in GIMP 2.8.6 and the final map layout was prepared in Apache OpenOffice 4.0.1 Draw.
Acknowledgements
I would like to thank Alina Kabuth for providing access to her data set on historical shoreline changes in Denmark and Lilith Gimbel for support during field work. All data were accessed through the geodata server at the Department of Geosciences and Natural Resource Management, University of Copenhagen, and I would like to acknowledge the work of Bjarne Fog for maintenance and software support.
ORCID
Lasse Sander http://orcid.org/0000-0003-1066-7952
Mikkel Fruergaard http://orcid.org/0000-0002-8575-157X
Additional information
Funding
References
- Bjørnsen, M., Clemmensen, L. B., Murray, A., & Pedersen, K. (2008). New evidence of the Littorina transgressions in the Kattegat: Optically Stimulated Luminescence dating of a beach ridge system on Anholt, Denmark. Boreas, 37, 157–168. doi:10.1111/j.1502-3885.2007.00008.x
- Boyd, R., Dalrymple, R., & Zaitlin, B. A. (1992). Classification of clastic coastal depositional environments. Sedimentary Geology, 80, 139–150. doi:10.1016/0037-0738(92)90037-R
- Cappelen, J., & Jørgensen, B. (1999). Observed wind speed and direction in Denmark – With climatological standard normals, 1961–90 (Technical Report No. 99-13). Copenhagen: Danish Meteorological Institute.
- Carter, R. W. G., & Orford, J. D. (1988). Conceptual model of coarse clastic barrier formation from multiple sediment sources. Geographical Review, 78, 221–239. doi:10.2307/214179
- Christensen, C. (1995). The littorina transgressions in Denmark. In A. Fischer (Ed.), Man and sea in the Mesolithic: Coastal settlement above and below present sea-level (pp. 15–22). Oxford: Oxbow Books.
- Clemmensen, L. B., Murray, A. S., & Nielsen, L. (2012). Quantitative constraints on the sea-level fall that terminated the Littorina Sea Stage, southern Scandinavia. Quaternary Science Reviews, 40, 54–63. doi:10.1016/j.quascirev.2012.03.001
- Clemmensen, L. B., Nielsen, L., Bendixen, M., & Murray, A. (2012). Morphology and sedimentary architecture of a beach-ridge system (Anholt, the Kattegat Sea): A record of punctuated coastal progradation and sea-level change over the past ∼1000 years. Boreas, 41, 422–434. doi:10.1111/j.1502-3885.2012.00250.x
- Clemmensen, L. B., Richardt, N., & Andersen, C. (2001). Holocene sea-level variation and spit development: Data from Skagen Odde, Denmark. The Holocene, 11, 323–331. doi:10.1191/095968301667877044
- Davis, R. A., Jr., & Hayes, M. O. (1984). What is a wave-dominated coast? Marine Geology, 60, 313–329. doi:10.1016/0025-3227(84)90155-5
- Fleming, K., Johnston, P., Zwartz, D., Yokoyama, Y., Lambeck, K., & Chappell, J. (1998). Refining the eustatic sea-level curve since the last glacial maximum using far-and intermediate-field sites. Earth and Planetary Science Letters, 163, 327–342. doi:10.1016/S0012-821X(98)00198-8
- Fruergaard, M., Andersen, T. J., Johannessen, P. N., Nielsen, L. H., & Pejrup, M. (2013). Major coastal impact induced by a 1000-year storm event. Scientific reports, 3. doi:10.1038/srep01051
- Geodatastyrelsen. (2014a). Geodata-info. Retrieved from http://www.geodata-info.dk
- Geodatastyrelsen. (2014b). Kortforsyningen. Retrieved from http://download.kortforsyningen.dk/
- Greenwood, R. O., & Orford, J. D. (2008). Temporal patterns and processes of retreat of drumlin coastal cliffs – Strangford Lough, Northern Ireland. Geomorphology, 94(1), 153–169. doi:10.1016/j.geomorph.2007.05.004
- Hansen, D. (Ed.). (1999). Vandmiljøet i Stavns Fjord og Nordby Bugt [The aquatic environment in Stavns Fjord and Norby Bay]. Højbjerg: Århus Amt, Natur og Miljø.
- Hansen, J. M., Aagaard, T., & Binderup, M. (2011). Absolute sea levels and isostatic changes of the eastern North Sea to central Baltic region during the last 900 years. Boreas, 41, 180–208. doi:10.1111/j.1502-3885.2011.00229.x
- Hede, M. U., Bendixen, M., Clemmensen, L. B., Kroon, A., & Nielsen, L. (2013). Joint interpretation of beach-ridge architecture and coastal topography show the validity of sea-level markers observed in ground-penetrating radar data. The Holocene, 23, 1238–1246. doi:10.1177/0959683613484618
- Hede, M. U., Sander, L., Nielsen, L., Clemmensen, L. B., Kroon, A., & Pejrup, M. (in press). Changes in Holocene relative sea-level and coastal morphology in relation to vertical land movement – a study of a raised beach-ridge system on Samsø, southwest Scandinavia. The Holocene.
- Hede, S. (2003). Prehistoric settlements and Holocene relative sea-level changes in north-west Sjælland, Denmark. Bulletin of the Geological Society of Denmark, 50, 141–149. Retrieved from http://2dgf.dk/xpdf/bull50-2-141-149.pdf
- Houmark-Nielsen, M. (1987). Pleistocene stratigraphy and glacial history of the central part of Denmark. Bulletin of the Geological Society of Denmark, 36, 1–189. Retrieved from http://2dgf.dk/xpdf/bull36_01-02-1-189.pdf
- Houmark-Nielsen, M. (2011). Pleistocene glaciations in Denmark: A closer look at chronology, ice dynamics and landforms. Developments in Quaternary Sciences, 15, 47–58. doi:10.1016/B978-0-444-53447-7.00005-2
- Iversen, J. (1937). Undersøgelser over Litorinatransgressioner i Danmark [Investigations into the Littorina transgressions in Denmark]. Meddelelser fra Dansk Geologisk Forening, 9, 223–232. Retrieved from http://2dgf.dk/xpdf/iversen1937_ocr.pdf
- Jakobsen, P. R., Hermansen, B., & Tougaard, L. (2011). Danmarks digitale jordartskort 1:25.000 [Digital soil map for Denmark 1:25.000] (Report 2011/40). Copenhagen: Danmarks og Grønlands Geologiske Undersøgelse (GEUS). Retrieved from http://www.geus.dk/DK/nature-climate/land/PublishingImages/dk_digitale_jordartskort_v_3_1.pdf
- Kabuth, A. K., & Kroon, A. (2014). Wave energy fluxes and multidecadal shoreline changes in two coastal embayments in Denmark. Ocean Dynamics, 64, 741–754. doi:10.1007/s10236-014-0709-6
- Kabuth, A. K., Kroon, A., & Petersen, J. B. (2013). Multidecadal shoreline changes in Denmark. Journal of Coastal Research. doi:10.2112/JCOASTRES-D-13-00139.1
- Kjær, K. H., Houmark-Nielsen, M., & Richardt, N. (2003). Ice-flow patterns and dispersal of erratics at the southwestern margin of the last Scandinavian Ice Sheet: Signature of palaeo-ice streams. Boreas, 32, 130–148. doi:10.1111/j.1502-3885.2003.tb01434.x
- Krog, H. (1979). The quaternary history of the Baltic. Denmark. In V. Gudelis & L.-K. König (Eds.), The quaternary history of the Baltic (pp. 207–217). Stockholm: Almqvist and Wiksell International.
- Kroon, A., Kabuth, A. K., & Westh, S. (2013). Morphologic evolution of a storm surge barrier system. Journal of Coastal Research, Special Issue No. 65, 529–534. doi:10.2112/SI65-090.1
- Lambeck, K., & Chappell, J. (2001). Sea level change through the last glacial cycle. Science, 292, 679–686. doi:10.1126/science.1059549
- Lambeck, K., Smither, C., & Johnston, P. (1998). Sea-level change, glacial rebound and mantle viscosity for northern Europe. Geophysical Journal International, 134, 102–144. doi:10.1046/j.1365-246x.1998.00541.x
- Livingstone, S. J., Cofaigh, C. Ó., Stokes, C. R., Hillenbrand, C. D., Vieli, A., & Jamieson, S. S. (2013). Glacial geomorphology of Marguerite Bay palaeo-ice stream, western Antarctic Peninsula. Journal of Maps, 9, 558–572. doi:10.1080/17445647.2013.829411
- Lundqvist, D., Jansen, D., Balstroem, T., & Christiansen, C. (2006). A GIS-based method to determine maximum fetch applied to the North Sea–Baltic Sea transition. Journal of Coastal Research, 223, 640–644. doi:10.2112/03-0125.1
- Madsen, V. (1897). Beskrivelse til geologisk kort over Danmark (i målestok 1:100.000): Kortbladet Samsø [Description of the geological map of Denmark (1:100.000 scale): Map sheet Samsø]. Copenhagen: Danmarks Geologiske Undersøgelse.
- Mertz, E. L. (1924). Oversigt over de sen- og postglaciale niveauændringer i Danmark [Survey of the late- and postglacial isostatic changes in Denmark]. Copenhagen: Danmarks Geologiske Undersøgelse.
- Miljøministeriet. (2005). Vejledning om højdesystemet [Guidelines for the ordnance datum]. Retrieved from http://www.gst.dk/media/gst/65263/Vejledning%20om%20h%C3%B8jdesystemet.pdf
- Mörner, N.-A. (1976). Eustatic changes during the last 8,000 years in view of radiocarbon calibration and new information from the Kattegat region and other northwestern European coastal areas. Palaeogeography, Palaeoclimatology, Palaeoecology, 19, 63–85. doi:10.1016/0031-0182(76)90042-0
- Nielsen, K. M., Stjernholm, B. Ø., Olsen, D. I., Müller-Wohlfeil, I. L., Madsen, A., Kjeldgaard, G., … Larsen, H. (2000). Areal informations systemet—AIS [Area Information System – AIS]. Copenhagen: Miljø- og Energiministeriet, Danmarks Miljøundersøgelser. Retrieved from http://www2.dmu.dk/1_Viden/2_Miljoe-tilstand/3_samfund/AIS/2_Rapport/ais_rapport.pdf
- Påsse, T., & Anderson, L. (2005). Shore-level displacement in Fennoscandia calculated from empirical data. Journal of the Geological Society of Sweden (GFF), 127, 253–268. doi:10.1080/11035890501274253
- Petersen, K. S. (1978). Den postglaciale transgression og molluskfaunaen I Tude Å-området, Store Bælt, Danmark. In Geological Survey of Denmark (Ed.). Yearbook 1977 (pp. 39–52). Copenhagen: Danmarks Geologiske Undersøgelse.
- Rasmussen, E. (1968). Stavns Fjord – et østdansk tidevandsområde [Stavns Fjord – an East Danish tidal area]. Geografisk Tidsskrift, 67, 70–92. Retrieved from http://img.kb.dk/tidsskriftdk/pdf/gto/gto_0067-PDF/gto_0067_97721.pdf
- Rosenkranz, B., & Frederiksen, P. (2011). Quality assessment of the Danish Elevation Model (DK-DEM) (Technical Report No. 12). Copenhagen: National Survey and Cadastre. Retrieved from ftp://ftp.kms.dk/download/Technical_Reports/KMS_Technical_Report_12.pdf
- Sander, L., Fruergaard, M., Koch, J., Johannessen, P. N., & Pejrup, M. (in press). Sedimentary constraints on Holocene relative sea-level and coastal evolution derived from coastal lagoon deposits: Samsø, Denmark. Boreas.
- Schou, A. (1945). Det Marine Forland [The marine foreland]. Copenhagen: Hagerups Forlag.
- Schou, A. (1949). Danish coastal cliffs in glacial deposits. Geografiska Annaler, 31, 357–364. doi:10.2307/520378
- Smith, M. J., Rose, J., & Booth, S. (2006). Geomorphological mapping of glacial landforms from remotely sensed data: An evaluation of the principal data sources and an assessment of their quality. Geomorphology, 76, 148–165. doi:10.1016/j.geomorph.2005.11.001
- Stéphan, P., Suanez, S., & Fichaut, B. (2012). Long-term morphodynamic evolution of the Sillon de Talbert gravel barrier spit, Brittany, France. Shore & Beach, 80, 19–36. Retrieved from http://pagesperso.univ-brest.fr/~suanez/Pg_publications/PUB_pdf/2012Shore&Beach.pdf
- Tagliapietra, D., Sigovini, M., & Ghirardini, A. V. (2009). A review of terms and definitions to categorise estuaries, lagoons and associated environments. Marine and Freshwater Research, 60, 497–509. doi:10.1071/MF08088
- Tamura, T. (2012). Beach ridges and prograded beach deposits as palaeoenvironment records. Earth-Science Reviews, 114, 279–297. doi:10.1016/j.earscirev.2012.06.004
- Taylor, M., & Stone, G. W. (1996). Beach-ridges: A review. Journal of Coastal Research, 12, 612–621. Retrieved from http://www.jstor.org/stable/4298509