Abstract
This paper presents and describes a glaciological and geomorphological map of Glacier Noir and Glacier Blanc, French Alps. Glacier Noir is a debris-covered glacier and is adjacent to Glacier Blanc, a clean-ice (debris-free) glacier. The glaciological and geomorphological evolution of Glacier Blanc is well known, but the evolution of Glacier Noir is poorly understood, as is the case for many debris-covered glaciers globally, despite their importance in a number of mountain ranges around the world (e.g. European and Southern Alps, the Himalayas and the Rockies). The accompanying map was created by manually digitising aerial ortho-images and historical georeferenced photographs from 1952 to 2013. The main glacial and geomorphological features of both glaciers were mapped, including debris cover, crevasses, moraines, hummocky terrain and scree areas. Hydrological features (supra- and pro-glacial streams and meltwater ponds) were also mapped. The map illustrates the key differences between Glacier Noir and Glacier Blanc, and is important for understanding future glaciological and geomorphological changes.
1. Introduction
Mountain glaciers are currently contributing ∼27% of the observed global sea-level rise with a large uncertainty of more than 20% (CitationJacob, Wahr, Pfeffer, & Swenson, 2012). Although the contribution of debris-free or clean-ice glaciers is well known, debris-covered glaciers and their contribution are still poorly understood. Debris-covered or debris-mantled glaciers are those where part of the surface of the ablation area is covered by a layer of rock debris including dust, ash and boulders of various sizes (CitationCogley et al., 2011; CitationHambrey et al., 2008; CitationSingh, Singh, & Haritashya, 2011).
Debris-covered glaciers represent ∼5% of all mountain glaciers worldwide (CitationWGMS & NSIDC, [1989] 2012) and the rate of sea-level rise attributed to them differs from clean-ice glaciers due to the insulating effect of the debris layer (CitationReznichenko, Davies, Shulmeister, & Mcsaveney, 2010). A better understanding of long-term glaciological processes on debris-covered glaciers is needed to reduce the uncertainty of their contribution to global sea level.
The debris layer on debris-covered glaciers derives from a number of sources, most notably valley-side rockfalls (CitationDeline & Kirkbride, 2009). These rockfalls can be significant at the glacier-scale, such as is the case for the Black Rapids Glacier (CitationShugar, Rabus, Clague, & Capps, 2012) and the Sherman Glacier (CitationMarangunic, 1972). These rock avalanches form specific deposits characterised by the regular thickness of the debris layer and angular grains (CitationHewitt, 2009). Other sources of debris include collapsing lateral moraines (CitationHambrey & Ehrmann, 2004) and debris elevated from subglacial and englacial positions to supraglacial positions (CitationGoodsell, Hambrey, & Glasser, 2005). The debris from these latter sources is more heterogeneous and may contain a mix of sub-angular to sub-rounded grains.
The supply of surface debris to the glacier's terminus has great control over the geomorphological processes occurring on and adjacent to that glacier (CitationReznichenko, Davies, & Alexander, 2011) and often results in the formation of very large geomorphological features, such as the Waiho Loop moraine in the Southern Alps, New Zealand (CitationTovar, Shulmeister, & Davies, 2008). From a glaciological perspective, the elevation of the snout of a debris-covered glacier would be lower than that of a similar clean-ice glacier. Specific glaciological and geomorphological dynamics of a debris-covered glacier are beginning to be considered in the interpretation of glaciated landscapes and landforms (CitationCarrasco, Pedraza, Dominguez-Villar, Willenbring, & Villa, 2013; CitationReznichenko, Davies, Shulmeister, & Winkler, 2012). Accurate interpretation and attribution of features to debris-covered glaciers can lead to re-interpretation of palaeo-climatic conditions contributing to their formation (CitationShulmeister, Davies, Evans, Hyatt, & Tovar, 2009; CitationVacco, Alley, & Pollard, 2010).
Here, a detailed Main Map is presented in order to provide the basis for investigating the geomorphological context of, and relationships between, a debris-covered glacier (Glacier Noir) and an adjacent and morphometrically similar clean-ice glacier (Glacier Blanc). This map will also help the re-interpretation of palaeo-landforms where debris-covered glaciers may have contributed to their formation.
2. Study site
Located in the Haute Vallée de St Pierre in the ‘Écrins’ National Park (Parc National des Écrins) in the French Alps (), Glacier Noir is a 4.5 km long debris-covered glacier with a surface area of 3.8 km². In contrast, the surface of adjacent Glacier Blanc is debris-free. Both glaciers were confluent in the Pré de Madame Carle field during the Little Ice Age (LIA, sixteenth to mid-nineteenth century, [CitationMann, 2002]). Pré de Madame Carle was a grazing field before it was transformed into an outwash plain by the advance of the combined glacier during the LIA (CitationLetreguilly & Reynaud, 1989).
Figure 1. Overview map presenting the position of the study site (red rectangle) in ‘Écrins’ national park (solid black line). Background map: IGN ©SCANREGIONAL. Inset: location (red marker) of the study site in the European Alps. Background Image: ©NASA.
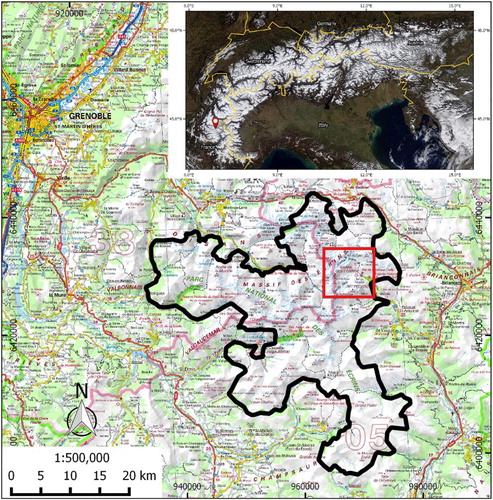
Glacier Noir (44°54′58″ N, 6°23′03″ E) has an elevation range of 2200–3600 m and comprises a main trunk (2200–2900 m in elevation) of 1.1 km² (2.6 km long), orientated WSW-ENE with a single tributary (2500–3600 m in elevation) of 2.7 km² (3.2 km long), orientated SSW-NNE. The tributary is now an independent glacier – named here Glacier Noir Sud – having separated from the main glacier between 2009 and 2013.
Glacier Blanc (44°56′25″ N, 6°22′42″ E) has an elevation range of 2500–4000 m and is 4.8 km² (5.5 km long), being orientated SW-NE in its upper section (3050–4000 m in elevation), which is relatively flat and then NW-SE in the steep crevassed area approaching its terminus (2500–3050 m in elevation). This main trunk is fed by six individual accumulation basins (cirques).
Both glaciers have attracted previous glaciological research, with Glacier Blanc being more widely studied (CitationAllix, 1922, Citation1929; CitationLetreguilly & Reynaud, 1989; CitationRabatel, Dedieu, & Reynaud, 2002; CitationRabatel, Dedieu, Thibert, Letreguilly, & Vincent, 2008; CitationRabatel, Letreguilly, Dedieu, & Eckert, 2013; CitationReynaud & Vincent, 2000, Citation 2002; CitationThibert, Faure, & Vincent, 2005; CitationVivian, 1967a) than Glacier Noir (CitationAllix, 1922, Citation1929; CitationCossart, Fort, Jomelli, & Grancher, 2006; CitationMount & Stott, 2008; CitationStott & Mount, 2007; CitationVivian, 1967b). The most recent studies have focused on sediment transport in the proglacial stream at Glacier Noir and on the variation of the equilibrium line altitude (ELA) at Glacier Blanc and its determination using optical remote sensing.
3. Data and methods
3.1. Data sources
Mapping was conducted by manually digitising aerial ortho-images (six RGB tiles of 5 km by 5 km with a 50 cm resolution) using QGIS software (Section 3.2). The National Institute of Geographic and Forestry Information (IGN) provided the ortho-images. These images are part of the French national database, © BDORTHO, and were taken during summer 2013.
The topography is derived from the IGN topographical map (Meije-Pelvoux 3436 ET), which is included in the database SCAN25. The scale of the map is 1:25,000.
The dates of formation of the moraines are from various sources:
A public engagement booklet edited by the ‘Écrins’ National Park (CitationÉcrins, 2005) on the glaciers present in the park.
Unpublished historical and archive documents owned by the ‘Écrins’ National Park.
Archived ortho-images and georeferenced aerial photographs extracted from the historical IGN database. This database is the compilation of previous versions of the © BDORTHO, grouping aerial scenes from 1952 to 2009.
The archived ortho-images were also used for the photo-interpretation of moraines, which are sensitive to the position of shadows (CitationOtto & Smith, 2013).
The interpretation of the ortho-images was verified and refined by direct field observation between mid-August and mid-September 2014, particularly where the ortho-images have shadowed areas or other areas where misinterpretation is possible. All ground-based photographs presented in this article and on the map were taken during the same period.
3.2. Software and digitising tools
All mapping and digitising was conducted using the geographic information system QGIS (CitationQGIS, 2014). Multiple versions of QGIS have been used (see Software Section below) as well as the updated versions of the following plugins:
autoSaver plugin, for automatic saves of the work in progress.
Digitizing Tools plugin, for additional digitising options.
GdalTools plugin, for elevation data extraction.
Georeferencer GDAL plugin, for the georeferencing of the aerial images.
GPS Tools plugin, for the import of field data.
Multipart Split plugin, for better management of multiple features in the same layer.
The map was designed using QGIS. The ground-based photographs presented on the map were modified using Adobe Illustrator CS2.
The digitisation of the ortho-images was conducted within a scale range of 1:1000–1:10,000, allowing a global view of each feature across the study site's large altitudinal range.
3.3. Map design
3.3.1. General principles
The mapped features are divided into four themes with additional background data: glaciological, geomorphological, hydrological and anthropogenic. The different colour schemes used are theme dependent. Glaciological features are depicted using only black and white colours. Geomorphological features are depicted in brown to yellow colours. In addition, vegetated features are presented in dark green. Hydrological features (ponds and streams) are depicted using different hues of blue. Although not essential to the map's principal purpose, anthropogenic features which provide important context (e.g. buildings) are depicted in grey. To bring contrast to the map, the background contour lines are depicted in light green.
3.3.2. Specific digitising cases
Moraines have been digitised only as moraine ridges. Ridges are the best indicators of the position of a moraine and so help to develop understanding of the retreat history of glaciers. Moraine extent has not been digitised to not overload the map. From field observations, crevasses and crevasse traces represent the large majority of structural features on Glacier Blanc and Glacier Noir. However, due to ortho-image resolution and the heavily disturbed area in the curve of Glacier Blanc, the recognition of foliations and/or lineations was particularly difficult, and consequently, some may have been digitised as crevasses.
In addition to digitising active and relict meltwater ponds, their areas of topographic influence (see Section 4.3.1) was also mapped as separate features because of their importance in the melting of debris-covered glaciers (CitationSakai, Takeuchi, Fujita, & Nakawo, 2000).
4. Description of the mapped features
4.1. Glaciological features
4.1.1. Glacier outlines
Glaciers were identified using the following definition: ‘mass of ice presenting active flow pattern', which is a simplified version of the GLIMS definition (CitationRau, Mauz, Vogt, Khalsa, & Raup, 2005). This definition was used as a guide to outline digitisation of both glaciers, although defining the lateral and frontal boundaries was easier for Glacier Blanc (i.e. between clean ice and proglacial debris) than for the ablation area of Glacier Noir, where the debris cover makes the identification of the glacier limit () and flow patterns more difficult (CitationCogley et al., 2011; CitationPaul et al., 2013).
4.1.2. Debris cover
For this map, we defined debris cover as where no clean ice is visible. The precise limits of debris-covered areas are difficult to define because of the continuous variations in debris concentration that are encountered in the field. In addition, the debris cover must have been persistent, that is, appearing in images separated by at least one year. By these criteria, no debris cover was mapped on Glacier Blanc because the debris-covered areas are temporary and localised, and are rapidly buried by snow in the accumulation area, or removed from the surface through crevasses in the ablation area.
4.1.3. Crevasses
Crevasses form when the extensional strain exceeds a critical threshold (CitationVaughan, 1993), resulting in fields of fractures with distinctive lengths and orientations. A fractured area is particularly visible on the lower section of Glacier Blanc where the glacier changes direction and becomes steeper.
On Glacier Noir, most of the crevasses are filled by debris that only leave traces of the crevasses visible on the surface. These crevasse traces create only low relief perturbations and are consequently not visible by direct observation in the field.
4.1.4. Nunataks and bare-rock areas
Nunataks are areas of glaciers where the bedrock is exposed (CitationSingh et al., 2011). Nunataks and other bare-rock areas are mainly present on the south-facing side of Glacier Blanc. The locations of these rock exposures vary, as they are dependent on the ice thickness and ice flow. Consequently, the features mapped are only those present when the aerial images were acquired in 2013.
4.2. Geomorphological features
These features are all related to the former presence of a glacier.
4.2.1. Moraines
Moraines are landforms built by the deposition by glaciers of glacial sediments (CitationSingh et al., 2011). There are many types of moraines (CitationBennett & Glasser, 2009); around Glacier Noir and Glacier Blanc, these are mostly frontal and lateral moraines and were mapped accordingly.
During the LIA, Glacier Noir and Glacier Blanc had a common terminus and produced a large moraine, like many other alpine glaciers. This LIA frontal moraine has been partially washed away by the proglacial stream, and currently, the only large LIA moraine intact is the lateral moraine of Glacier Noir. This moraine is recognisable because of its large size compared to the surrounding moraines ().
4.2.2. Gullies
Gullies are formed in areas of unconsolidated sediment where the runoff from rain and snowmelt creates micro-valleys. In the study site, most of the gullies are on the ice-proximal flanks of moraines.
The process of gullying is an active phenomenon () and was observed during heavy rainfall events during the fieldwork period. This process contributes widely to the erosion of moraines.
4.2.3. Scree areas
According to CitationSingh et al. (2011), scree material (also called debris) is ‘Unconsolidated sediment, larger than 1 mm, of angular or rounded angular fragments of boulders (clasts), predominantly originating from physical weathering’. Scree areas are steep zones of scree material. All the active scree areas around Glacier Noir and Glacier Blanc face SW to SE. Scree clast size is variable, ranging from pebble to boulder-size.
Three types of scree areas were mapped:
Active scree areas where traces of rock falls are visible and where regular rock falls have been observed in the field. They are mainly located around the Glacier Noir catchment.
Stabilised scree areas without traces of active rock falls located on the eastern side of the terminus of Glacier Blanc and above the outwash plain.
Vegetated scree areas near the entrance of Haute Vallée de St-Pierre.
presents the geological context for scree production. Production appears to be independent of lithology (gneiss or granite) and to be primarily driven by slope orientation (CitationNagai, Fujita, Nuimura, & Sakai, 2013).
Figure 5. Geological map of the study site with superimposed scree areas. Geological variations (mainly gneiss except for Glacier Noir Sud with granite) in the study area cannot explain the origin of the scree areas. Slope orientation is the main factor in the scree production. Adapted from Bureau de Recherche Géologiques et Minières (BRGM) maps 0822N and 0823N.
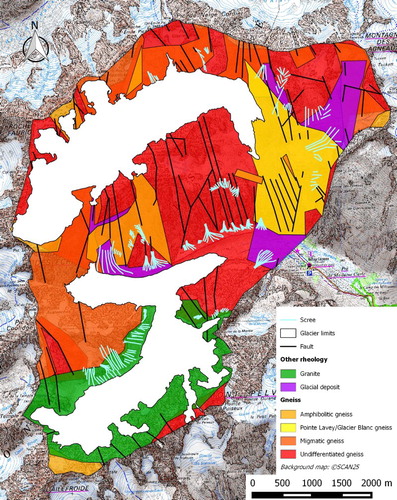
4.2.4. Hummocky terrain
On the map, hummocky terrain () designates an assemblage of debris and glacial sediment pits and mounds including small, possibly ice-cored, moraines (CitationSingh et al., 2011).
Figure 6. Hummocky terrain in the proglacial area of Glacier Blanc. The hummocky moraines (green) are easily eroded by the proglacial stream. The frontal moraine (white) marks the lower limit of this hummocky area.
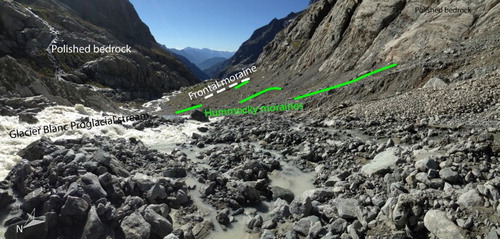
The hummocky terrain is located in the proglacial area of both glaciers and in a former lower accumulation cirque of Glacier Noir Sud. Like gullies, these areas are particularly active and their morphology evolves closely with the variation of the proglacial streams, especially during heavy rainfall events.
4.2.5. Bedrock with incised channels
Large areas of bedrock (gneiss) with incised channels are visible in front of Glacier Blanc, exposed as the glacier receded. Field observations confirm that some of the channels have subglacial origins and are probably Nye channels. Nye channels (or N-Channels) are subglacial channels directly carved into bedrock by meltwater discharge (CitationNye, 1973). Most of the visible channels are now abandoned except for those occupied by the glacier's main proglacial streams.
4.2.6. Outwash plain
An outwash plain is a large flat area covered with well-sorted glaciofluvial sediment. Braided rivers often develop widely in outwash plains, for example in Iceland where they are called ‘sandur’ because of the predominance of sand- and gravel-sized sediment across such plains. The proglacial streams of Glacier Noir and Glacier Blanc converge in the upper part of the outwash plain to form a dynamic braided stream system as shown in at two different dates.
Figure 7. Outwash plain of Glacier Noir and Glacier Blanc. As a consequence of the heavy rainfall event of 26th August 2014, the proglacial stream shifted from the northern edge (left hand side image) of the outwash plain to the southern edge (right hand side image), illustrating this highly dynamic environment.
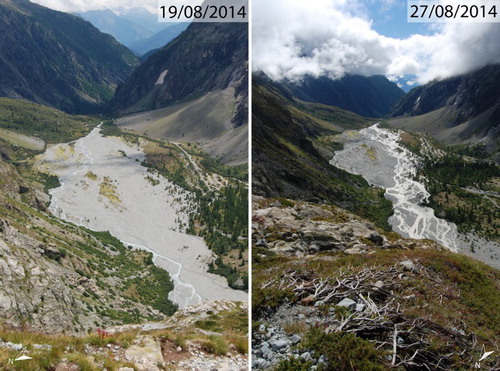
4.3. Hydrological features
4.3.1. Meltwater ponds
Meltwater ponds are depressions on the ice surface that are filled with water released by the melt of snow and ice. Numerous, often large, supraglacial meltwater ponds are a common feature on debris-covered glaciers. Indeed, such ponds form the basis of one key classification of the morphological evolution of debris-covered glaciers (CitationBenn et al., 2012).
Meltwater ponds form by differential melting between debris-covered and clean-ice areas. Ablation of the latter is faster than the former, creating a depression – here called the area of topographic influence – where water can be stored. This process involves a positive feedback loop where the edge of the depression becomes steeper and so less debris-covered, inducing further melting and consequently steepening of the side. This feedback loop gradually expands the area of topographic influence of meltwater ponds.
However, these meltwater ponds are eventually drained supraglacially via a channel or englacially via crevasses. The drainage process creates relict/trace ponds () where the difference between the pond itself and the area of topographic influence is still visible.
4.3.2. Streams
Streams on the study site are produced by the melt of glaciers. They are found in two different positions: on the surface (supraglacial streams) and in front (proglacial streams) of both glaciers. Due to the dynamics (water discharge, deposition of sediment) and ephemeral nature of proglacial streams, especially in the outwash plain, only principal active channels were mapped, illustrating the situation at the time the aerial images were acquired.
Supraglacial streams could only be observed on the debris-covered surface of Glacier Noir. Most of the mapped streams were restricted to the ablation area. No visual expression of supraglacial streams was found on aerial images of Glacier Blanc despite their presence in the field (). Therefore, supraglacial streams were not mapped on Glacier Blanc.
Figure 9. Various meltwater channels in the study area. (a) Active meltwater channel just below the accumulation area of Glacier Noir. (b) Trace of meltwater channels in the ablation area of Glacier Noir. (c) Active meltwater channels on Glacier Blanc highlighted by pink dye. Note the difference in scale between these images.
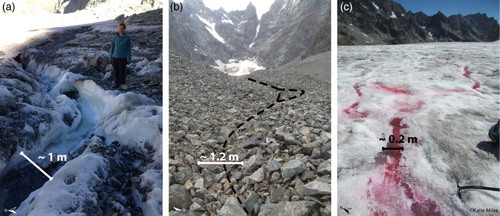
4.4. Anthropogenic features and elevation data
The Glacier Noir and Glacier Blanc site is a tourist attraction in the ‘Écrins’ National Park and so buildings (three refuges, one visitor centre and public restroom facilities), roads and hiking trails were additionally mapped to provide context.
Contour lines from the IGN 1998 digital elevation model (DEM) were added as background information. To clarify the topographic context of the map, arête lines were added on to the DEM as well as some altitude points.
5. Conclusion
We describe here a new glaciological and geomorphological map of Glacier Noir and Glacier Blanc in the French Alps. The mapped features were divided into four different themes (glaciological, geomorphological, hydrological and anthropogenic) to facilitate the understanding of the map and future studies and comparisons. However, these four themes interact closely. Glacier Noir and Glacier Blanc are the main agents of sediment transport and deposition, creating a range of geomorphological features, from sand layers in the proglacial area to LIA moraines. The streams are, on the contrary, the main agents of erosion on the surface of Glacier Noir, acting to transfer sediment of the debris layer from the top of the glacier to the terminus, as well as eroding the proglacial terrain of both glaciers to create an outwash plain further downstream. Meltwater ponds are the perfect example of the interaction of glacial (melting of debris-free ice cliffs), geomorphological (back wasting of debris from the layer) and hydrological (storage and drainage of significant quantities of water) processes. Finally, anthropogenic features such as roads and bridges modify erosional/depositional patterns in a complex way, especially in the outwash plain.
Understanding these processes and their interactions is part of a larger research project on the impact of variations in supraglacial debris cover on glacier evolution and dynamic response to climatic forcing.
Software
Database development and map production were performed using QGIS 2.2, 2.4, 2.6, and 2.6.1. Additional QGIS plugins used include autoSaver, Digitizing Tools, GdalTools, Georeferencer GDAL, GPS Tools and Multipart Split. Figures on the map were edited using Adobe Illustrator CS2.
Glaciological and geomorphological map of Glacier Noir and Glacier Blanc, French Alps
Download PDF (13.3 MB)Acknowledgements
We would like to thank Stephen Jennings for his useful comments on the map and Patricia Gongal for her helpful comments on the manuscript.
Disclosure statement
No potential conflict of interest was reported by the authors.
ORCID
Pierre Lardeux http://orcid.org/0000-0001-7860-3053
Neil Glasser http://orcid.org/0000-0002-8245-2670
Bryn Hubbard http://orcid.org/0000-0002-3565-3875
Additional information
Funding
References
- Allix, A. (1922). Les glaciers des Alpes françaises en 1921. Revue de géographie alpine, 10, 325–333. doi: 10.3406/rga.1922.1697
- Allix, A. (1929). Observations glaciologiques faites en dauphiné jusqu'en 1924. Les Études rhodaniennes, 5, 185–186.
- Benn, D. I., Bolch, T., Hands, K., Gulley, J., Luckman, A., Nicholson, L. I., … Wiseman, S. (2012). Response of debris-covered glaciers in the Mount Everest region to recent warming, and implications for outburst flood hazards. Earth-Science Reviews, 114, 156–174. doi: 10.1016/j.earscirev.2012.03.008
- Bennett, M. M., & Glasser, N. F. (2009). Glacial geology: Ice sheets and landforms. Chichester: John Wiley & Sons.
- Carrasco, R. M., Pedraza, J., Dominguez-Villar, D., Willenbring, J. K., & Villa, J. (2013). Supraglacial debris supply in the Cuerpo de Hombre paleoglacier (Spanish Central System): Reconstruction and interpretation of a rock avalanche event. Geografiska Annaler Series a-Physical Geography, 95, 211–226. doi: 10.1111/geoa.12010
- Cogley, J. G., Hock, R., Rasmussen, L. A., Arendt, A. A., Bauder, A., Braithwaite, R. J., … Zemp, M. (2011). Glossary of glacier mass balance and related terms. (IHP-VII Technical Documents in Hydrology No. 86, IACS Contribution No. 2). Paris: UNESCO-IHP.
- Cossart, E., Fort, M., Jomelli, V., & Grancher, D. (2006). Les variations glaciaires en Haute-Durance (Briançonnais, Hautes-Alpes) depuis la fin du xix e siècle: mise au point d'après les documents d'archives et la lichénométrie. Quaternaire, 17, 75–92. doi: 10.4000/quaternaire.694
- Deline, P., & Kirkbride, M. P. (2009). Rock avalanches on a glacier and morainic complex in Haut Val Ferret (Mont Blanc Massif, Italy). Geomorphology, 103, 80–92. doi: 10.1016/j.geomorph.2007.10.020
- Ecrins, P. N. D. (2005). Territoire Écrins – Les Cahiers Thematiques du Parc National: Les glaciers. In: Écrins, P. N. D. (ed.).
- Goodsell, B., Hambrey, M. J., & Glasser, N. F. (2005). Debris transport in a temperate valley glacier: Haut Glacier d'Arolla, Valais, Switzerland. Journal of Glaciology, 51, 139–146. doi: 10.3189/172756505781829647
- Hambrey, M. J., & Ehrmann, W. (2004). Modification of sediment characteristics during glacial transport in high-alpine catchments: Mount cook area, New Zealand. Boreas, 33, 300–318. doi: 10.1080/03009480410001965
- Hambrey, M. J., Quincey, D. J., Glasser, N. F., Reynolds, J. M., Richardson, S. J., & Clemmens, S. (2008). Sedimentological, geomorphological and dynamic context of debris-mantled glaciers, Mount Everest (Sagarmatha) region, Nepal. Quaternary Science Reviews, 27, 2361–2389. doi: 10.1016/j.quascirev.2008.08.010
- Hewitt, K. (2009). Rock avalanches that travel onto glaciers and related developments, Karakoram Himalaya, Inner Asia. Geomorphology, 103, 66–79. doi: 10.1016/j.geomorph.2007.10.017
- Jacob, T., Wahr, J., Pfeffer, W. T., & Swenson, S. (2012). Recent contributions of glaciers and ice caps to sea level rise. Nature, 482, 514–518. doi: 10.1038/nature10847
- Letreguilly, A., & Reynaud, L. (1989). Past and forecast fluctuations of glacier Blanc (French Alps). Annals of Glaciology, 13, 159–163.
- Mann, M. E. (2002). Little ice age. Encyclopedia of global environmental change. Chichester: John Wiley & Sons.
- Marangunic, C. (1972). Effects of a landslide on Sherman glacier. Columbus, OH: Institute of Polar Studies.
- Mount, N., & Stott, T. (2008). A discrete Bayesian network to investigate suspended sediment concentrations in an Alpine proglacial zone. Hydrological Processes, 22, 3772–3784. doi: 10.1002/hyp.6981
- Nagai, H., Fujita, K., Nuimura, T., & Sakai, A. (2013). Southwest-facing slopes control the formation of debris-covered glaciers in the Bhutan Himalaya. The Cryosphere, 7, 1303–1314. doi: 10.5194/tc-7-1303-2013
- Nye, J. F. (1973). Water at the bed of a glacier. Hydrology of Glaciers. Cambridge: IASH Publisher.
- Otto, J.-C., & Smith, M. J. (2013). Geomorphological mapping. In L. Clarke (Ed.), Geomorphological techniques (Online Edition). London: British Society for Geomorphology.
- Paul, F., Barrand, N. E., Baumann, S., Berthier, E., Bolch, T., Casey, K., … Winsvold, S. (2013). On the accuracy of glacier outlines derived from remote-sensing data. Annals of Glaciology, 54, 171–182. doi: 10.3189/2013AoG63A296
- Qgis, D. T. (2014). QGIS Geographic Information System [Online]. http://www.qgis.org/ Open Source Geospatial Foundation Project. Retrieved June 9, 2015, from http://qgis.osgeo.org.
- Rabatel, A., Dedieu, J. P., & Reynaud, L. (2002). Reconstitution des fluctuations du bilan de masse du Glacier Blanc (Massif des Écrins, France) entre 1985 et 2000, par télédétection optique (imagerie Spot et Landsat). La Houille Blanche, 6, 64–71. doi: 10.1051/lhb/2002086
- Rabatel, A., Dedieu, J. P., Thibert, E., Letreguilly, A., & Vincent, C. (2008). 25 years (1981–2005) of equilibrium-line altitude and mass-balance reconstruction on Glacier Blanc, French Alps, using remote-sensing methods and meteorological data. Journal of Glaciology, 54, 307–314. doi: 10.3189/002214308784886063
- Rabatel, A., Letreguilly, A., Dedieu, J. P., & Eckert, N. (2013). Changes in glacier equilibrium-line altitude in the western Alps from 1984 to 2010: evaluation by remote sensing and modeling of the morpho-topographic and climate controls. The Cryosphere, 7, 1455–1471. doi: 10.5194/tc-7-1455-2013
- Rau, F., Mauz, F., Vogt, S., Khalsa, S., & Raup, B. (2005). Illustrated GLIMS glacier classification manual, Version 1.0. GLIMS Regional Centre, ‘Antarctic Peninsula’, GLIMS (Global Land Ice Measurement from Space), NSIDC.
- Reynaud, L., & Vincent, C. (2000). Relevés de fluctuations sur quelques glaciers des Alpes françaises. La Houille Blanche, 5, 79–86. doi: 10.1051/lhb/2000052
- Reynaud, L., & Vincent, C. (2002). Histoire des fluctuations des glaciers en remontant le Petit Age de Glace. La Houille blanche, 8, 16–19. doi: 10.1051/lhb/2002099
- Reznichenko, N., Davies, T., Shulmeister, J., & Mcsaveney, M. (2010). Effects of debris on ice-surface melting rates: an experimental study. Journal of Glaciology, 56, 384–394. doi: 10.3189/002214310792447725
- Reznichenko, N. V., Davies, T. R. H., & Alexander, D. J. (2011). Effects of rock avalanches on glacier behaviour and moraine formation. Geomorphology, 132, 327–338. doi: 10.1016/j.geomorph.2011.05.019
- Reznichenko, N. V., Davies, T. R. H., Shulmeister, J., & Winkler, S. (2012). Influence of rock avalanches upon the formation of moraines and their subsequent palaeoclimatic interpretation: a critical appraisal. Zeitschrift Fur Geomorphologie, 56, 37–54. doi: 10.1127/0372-8854/2012/S-00079
- Sakai, A., Takeuchi, N., Fujita, K., & Nakawo, M. (2000, September 13–15). Role of supraglacial ponds in the ablation process of a debris-covered glacier in the Nepal Himalayas. Debris-covered Glaciers: Proceedings of an International Workshop Held at the University of Washington in Seattle, Washington, USA, IAHS, pp. 119–132.
- Shugar, D. H., Rabus, B. T., Clague, J. J., & Capps, D. M. (2012). The response of black rapids Glacier, Alaska, to the Denali earthquake rock avalanches. Journal of Geophysical Research-Earth Surface, 117, 1–14. doi: 10.1029/2011JF002011
- Shulmeister, J., Davies, T. R., Evans, D. J. A., Hyatt, O. M., & Tovar, D. S. (2009). Catastrophic landslides, glacier behaviour and moraine formation – a view from an active plate margin. Quaternary Science Reviews, 28, 1085–1096. doi: 10.1016/j.quascirev.2008.11.015
- Singh, V. P., Singh, P., & Haritashya, U. K. (2011). Encyclopedia of snow, ice and glaciers. Dordrecht: Springer.
- Stott, T., & Mount, N. (2007). Alpine proglacial suspended sediment dynamics in warm and cool ablation seasons: Implications for global warming. Journal of Hydrology, 332, 259–270. doi: 10.1016/j.jhydrol.2006.07.001
- Thibert, E., Faure, J., & Vincent, C. (2005). Bilans de masse du Glacier Blanc entre 1952, 1981 et 2002 obtenus par modèles numériques de terrain. La Houille Blanche, 2, 72–78. doi: 10.1051/lhb:200502010
- Tovar, D. S., Shulmeister, J., & Davies, T. R. (2008). Evidence for a landslide origin of New Zealand's waiho Loop moraine. Nature Geoscience, 1, 524–526. doi: 10.1038/ngeo249
- Vacco, D. A., Alley, R. B., & Pollard, D. (2010). Glacial advance and stagnation caused by rock avalanches. Earth and Planetary Science Letters, 294, 123–130. doi: 10.1016/j.epsl.2010.03.019
- Vaughan, D. G. (1993). Relating the occurrence of crevasses to surface strain rates. Journal of Glaciology, 39(132), 255–266.
- Vivian, R. (1967a). Le glacier Blanc. Revue de géographie alpine, 55, 729–732. doi: 10.3406/rga.1967.3351
- Vivian, R. (1967b). Le glacier Noir. Revue de géographie alpine, 55, 733–736. doi: 10.3406/rga.1967.3352
- WGMS & NSIDC. ([1989] 2012). World glacier inventory. Compiled and made available by the World Glacier Monitoring Service, Zurich, Switzerland, and the National Snow and Ice Data Center, Boulder, CO.