ABSTRACT
On 25 October 2011, the eastern Liguria (Vara Valley and Cinque Terre area) and northwestern Tuscany (Magra Valley) were affected by an extreme rainstorm (almost 600 mm/24 h) that caused floods, thousands of shallow landslides, 13 casualties and damage to villages and infrastructure. This study aims at analysing the main features of the 25 October 2011 shallow landslides occurred in the Pogliaschina Torrent basin (25 km2 wide, Vara Valley), in order to investigate the influence of specific predisposing factors (land use, geological and structural setting, plan and profile curvature, slope angle and aspect) on landslide occurrence. For this purpose, both a landslide inventory map and a geology map (scale 1:10,000) were prepared. In addition, a database including the main geological, geomorphological, structural and land use features of the landslide source areas was implemented. The relationship between landslide source areas and the main predisposing factors was evaluated through spatial and statistical analysis.
1. Introduction
On 25 October 2011 an extreme rainstorm struck eastern Liguria (Vara Valley and Cinque Terre area; ) and northwestern Tuscany (Magra Valley). The rainstorm triggered hundreds of shallow landslides and floods, causing 13 fatalities and severe damage to villages, infrastructure and roads (CitationBartelletti, D’Amato Avanzi, Galanti, Giannecchini, & Mazzali, 2015; CitationBordoni et al., 2015; CitationD’Amato Avanzi, Galanti, Giannecchini, & Bartelletti, 2015; CitationGalanti, Giannecchini, D’Amato Avanzi, Barsanti, & Benvenuto, 2016; CitationGalve, Cevasco, Brandolini, & Soldati, 2015). The rain gauges located at Brugnato, Calice al Cornoviglio (Vara Valley) and Monterosso (Cinque Terre) recorded 424.2, 340.4 and 259.2 mm in 6 h (from 10:00 to 16:00 UTC), and 538.2, 452.8 and 381.8 mm in 24 h, respectively ().
Figure 2. Isohyet map of the 25 October 2011 rainstorm (cumulative rainfall in 24 h). The violet line borders the Pogliaschina T. basin.
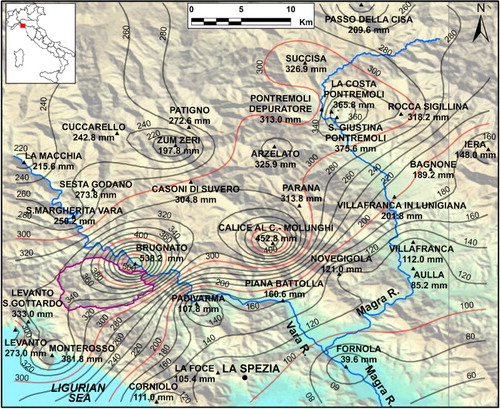
During recent decades, a high number of rainfall-induced landslides occurred in eastern Liguria (e.g. Lavagna and Vara Valleys) and in nearby northwestern Tuscany (Apuan Alps, Garfagnana, Versilia and Serchio River Valley) – as happened on 19 June 1996 (Versilia and Garfagnana, 14 causalities; CitationD’Amato Avanzi, Giannecchini, & Puccinelli, 2004), 6 November 2000 (Versilia and Serchio Valley; CitationD’Amato Avanzi, Giannecchini, & Puccinelli, 2002), 20 November 2000 (Serchio River Valley, 5 causalities; CitationD’Amato Avanzi et al., 2002), 23 September 2003 (Apuan Alps), 31 October 2010 (Massa-Carrara province, northwestern Tuscany, 3 casualties; CitationD’Amato Avanzi, Galanti, Giannecchini, & Puccinelli, 2013), 22–25 December 2009 (Serchio, Magra and Vara Valleys; CitationD’Amato Avanzi et al., 2013) and 22–23 December 2010 (Magra Valley; CitationSacchi, 2012). A recent rainstorm also occurred on 10 November 2014 in Lavagna Valley (CitationCevasco, Pepe, D’Amato Avanzi, & Giannecchini, 2015).
In the Pogliaschina Torrent basin (Vara Valley; ), the 25 October 2011 rainstorm showed very high rainfall intensity (143.4 mm from 13:00 to 14:00 UTC at Brugnato, nearby the Pogliaschina T. basin; CitationD’Amato Avanzi, Galanti, Giannecchini, Mazzali, & Saulle, 2013; CitationPersichillo et al., 2016), inducing hundreds of shallow landslides. Most of them were first-time movements, mainly complex, translational debris slide-flows (CitationCruden & Varnes, 1996). The failure surface was commonly located at the soil-bedrock contact, sometimes involving portions of weathered bedrock. Despite the small volume of material mobilized, this type of landslide is very dangerous due to the following factors: extremely rapid triggering and evolution, difficulty of temporal and spatial prediction, lack of knowledge about triggering mechanisms, high areal frequency and high run-out distance (CitationD’Amato Avanzi et al., 2004; CitationD’Amato Avanzi, Galanti et al., 2013; CitationGiannecchini, Galanti, D’Amato Avanzi, & Barsanti, 2016).
Slope stability is generally controlled by a series of interrelated environmental parameters, namely lithology, structure and texture of rock and soil, morphology and the topographic surface (described by attributes such as slope angle, curvature, etc.), hydrological conditions, vegetation, land use and land use practise (CitationVan Westen, 2000; CitationVan Westen, Castellanos, & Kuriakose, 2008). During intense rainfall, some parameters can influence slope stability more than others and can contribute to generate shallow landslides (CitationCevasco, Pepe, & Brandolini, 2014). For this reason, the analysis of the relationship between landslide distribution and environmental parameters not only leads to a better investigation of the landslide mechanisms, but can also offer a basic tool for predicting future landslides (CitationZhou, Lee, Li, & Xu, 2002).
This paper focuses on the spatial distribution of the shallow landslides that occurred in the Pogliaschina T. basin on 25 October 2011 and on linkages with environmental parameters, in order to detect the main combination of parameters that contribute to shallow landslide initiation. With this purpose, both a detailed landslide inventory map related to the 25 October 2011 event and a digital geological map of the Pogliaschina T. basin (1:10,000 scale) were prepared. The landslide inventory and geological maps were stored in a database implemented using a geographical information system (GIS). GIS is an excellent tool in the analysis of landslide distribution in relation to spatial variability of terrain variables (CitationVan Westen, 2000). In this study, the role of each environmental parameter on landslide occurrence was investigated through landslide database queries.
For the proposed methodology, this work represents an important first step in evaluating shallow landslide susceptibility in the Pogliaschina T. basin.
2. Study area
The Pogliaschina T. basin is situated in the Vara Valley (northern Apennines, Italy; ). It is 25 km wide and has a maximum elevation of about 720 m a.s.l., while the valley bottom is at about 95–100 m a.s.l. (). The climate is typically Mediterranean, with dry and hot summers and mild winters. At this latitude rainstorms mostly occur in autumn (October–November; CitationGiannecchini & D’Amato Avanzi, 2012), and the mean annual precipitation is about 1500 mm (CitationMondini et al., 2014). Vegetation is mainly represented by hardwood (mostly chestnut) and coniferous (maritime pine) forests. Agricultural areas include cultivated and abandoned terraces for olive groves and vineyards, particularly common around the villages of Borghetto di Vara, Cassana and L’Ago.
Figure 3. Comparison between the landslide inventory map and 24 h isohyets of the 25 October 2011 rainfall in a 5×5 m DEM. The box shows a zoom of the landslide inventory.
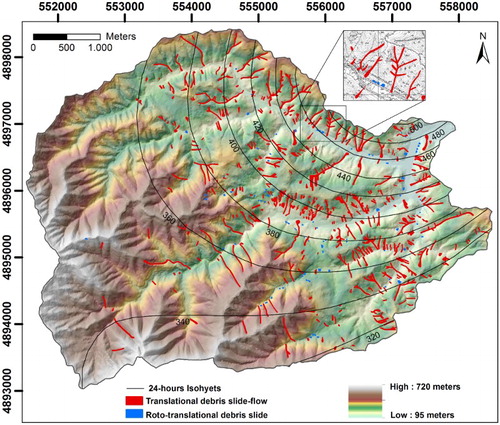
The Vara Valley occupies a morpho-structural depression enclosed between the La Spezia ridge to the west and the M. Picchiara-M. Cornoviglio ridge to the east, produced from two main tectonic phases which formed the northern Apennines (CitationRaggi, 1985). From the Cretaceous to the Eocene, a compression phase was responsible for the development of synclinal and anticlinal folds; from the Upper Miocene an extension phase produced sets of normal faults, intersecting the previous structure (CitationBernini & Lasagna, 1988). The basin is underlain by tectonic units coming from the following paleogeographic domains (CitationPuccinelli, D’Amato Avanzi, & Perilli, 2015b): Internal Ligurian Domain, Sub-Ligurian Domain, Tuscan Domain ().
In the study area, the bedrock is mainly formed of medium and coarse quartz-feldspathic sandstone (Macigno Fm., MAC; Tuscan Nappe Unit) and quartz-feldspathic greywacke sandstone-siltstone (Arenarie di Monte Gottero Fm., GOT; Gottero Unit). These formations underlie 46.9% (west-southwest portion) and 34.1% (northeast-eastern portion) of the whole basin (), respectively.
The geological units cropping out in the basin are listed from the upper to the lower one in and are described on the Main Map.
3. Methods
The cartographic basemap is a geological map (1:10,000 scale; ), derived from geological maps produced by CitationAbbate (1969), CitationAbbate et al. (2005), CitationBortolotti et al. (2011), CitationPuccinelli, D’Amato Avanzi, and Perilli (2015a). The 25 October 2011 landslide inventory map was overlain on the geological map. The landslide inventory map () was obtained by the analysis of post-event aerial photographs, orthophotos, Google Earth images and field survey. The aerial photos were acquired on 28 October 2011, provided by the Liguria Regional Administration, while the orthophotos were acquired on 28 November 2011, taken by the Friuli Venezia Giulia Regional Administration and provided by the Liguria Regional Administration. The aerial survey covers the eastern portion of the basin where many landslides occurred. However, the field survey involved the whole basin and allowed compilation of data for the entire study area. Google Earth images were used to identify morphological changes and to detect landslide scars older than the 25 October event using older imagery.
The landslide database was implemented according to the guidelines of the IFFI project (Italian Landslide Inventory; CitationAmanti et al., 2004, Citation2005) and those of the landslide inventory model proposed by the LaMMA Consortium – Environmental Modelling and Monitoring Laboratory for Sustainable Development (CitationConsorzio LaMMA, 2012). Each landslide source area is represented as a georeferenced point, called a PIFF (Landslide Identification Point), placed at the highest point of the landslide crown. Different to the IFFI project, in this study the landslide identification point was placed inside the perimeter of each landslide rather than on its boundary, so that it coincides with the point label feature containing the attribute table that is transferred to the landslide polygon during its construction using Esri ArcGIS.
The attribute table for the landslide source areas includes both general information (number, position, type of movement) and the main environmental parameters (geology, structural setting, land use, slope angle, aspect, plan and profile curvature). The type of movement uses the CitationCruden and Varnes (1996) classification. Geology and land use features were obtained from the geology map of the Pogliaschina T. basin and land use map of the Liguria Region at 1:10,000 scale (CitationRegione Liguria, 2013), respectively.
The morphometric parameters (slope angle, aspect, plan and profile curvature) were derived from the Digital Elevation Model (DEM 5 × 5 m) using Esri ArcGIS. Slope angle (S; change of the maximum slope), aspect (A; direction of steepest slope with respect to North), plan curvature (PLC; intersection between the topographic surface and the XY plane) and profile curvature (PRC; intersection between the topographic surface and the YZ plane parallel to the direction of the maximum slope) maps were obtained for each cell. Plan curvature allows verification of the concavity (PLC < 0) or convexity (PLC > 0) of slopes and consequently indicates the convergence or divergence of superficial and sub-surface flow. Profile curvature describes the change of slope angle affecting flow acceleration and deceleration, which influences erosion and deposition. Positive values indicate that the surface is convex and that flow accelerates, while negative values indicate that the surface is concave and that flow decelerates.
By overlaying geology, land use, slope angle, aspect, plan and profile curvature maps with the landslide inventory map, the response to the variation of such environmental parameters was analysed for each landslide source area. Landslide distribution for each class of the considered parameters was defined, the related landslide index (LI; landslide area in a given feature class/area of that class) was computed, and the results compared. The subdivision of environmental parameters into classes having a LI higher or lower than that of the whole basin (LIB, ratio between the total landslide area and the whole basin area), allows us to verify whether they are correlated with landslide occurrence. Moreover, the LI analysis allows identification of parameters which are more or less susceptible to landsliding. If a parameter includes classes showing a great difference in LI, it is a significant parameter in shallow landslide assessment; otherwise, if a parameter has similar values of LI, it is not well related with the landslide inventory and consequently does not play an important role in landslide susceptibility.
4. Results
The 2011 heavy rainstorm triggered hundreds of shallow landslides. Their main features are now summarized and related to the most significant environmental parameters.
4.1. Landslide features
658 shallow landslides were triggered during the 25 October 2011 rainstorm in the Pogliaschina T. basin (). 569 of them were classified as complex, translational debris slide-flows (CitationCruden & Varnes, 1996; (a and b)). Most of these were first-time superficial (0.3–2 m thick) and linear (width/length ratio 0.03–0.5) landslides, mainly involving coarse-grained soil and sometimes portions of bedrock.
Figure 5. Examples of complex, translational debris slide-flows (a), source area of debris slide-flow (b) and roto-translational slide (c).
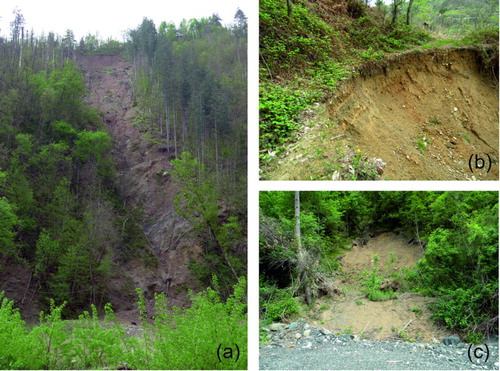
The other 89 landslides fall into the rotational debris slide class (CitationCruden & Varnes, 1996; (c)), with a small size and a width/length ratio > 1. They were principally located upstream of road cuts, where the anthropogenic modification of the slopes may have influenced the failure (CitationD’Amato Avanzi et al., 2013; CitationGiannecchini, Galanti, & D’Amato Avanzi, 2012).
In the study area, the 2011 landslide density is 26 landslides per km2, with LIB of 3.0% (dashed line in –). 70.5% of complex, translational debris slide-flows intersected the hydrological network and increased the debris and wood transport of the Pogliaschina Torrent. Considering a mean soil thickness of 1.5 m involved in landsliding, and a total landslide area of about 783,000 m2, the total volume of material mobilized by the landslides was estimated at 1,174,500 m3.
Figure 6. Percentage of landslide and LIG for the different geological classes. (ACC = Argille e calcari di Canetolo Fm., APA = Argille a palombini Fm., GOT = Monte Gottero Fm.; LVG = Val Lavagna Fm., MAC = Macigno Fm., MAI = Maiolica Fm., SRN = Serpentiniti Fm., STO = Scaglia toscana Fm.). The dashed black line shows the LIB.
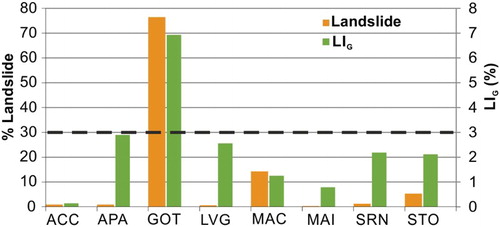
4.2. Landslides and bedrock type
Shallow landslides mainly involved soils covering the GOT and MAC formations. These very similar formations respectively underlie 46.9% (west-southwestern portion) and 34.1% (northeastern portion) of the whole basin (), where 76.6% and 14.2% of the 25 October 2011 landslides respectively occurred. The landslide index in relation to the basin geology (LIG, ratio between the landslide area in each formation and the total area underlain by the same formation) of the GOT and MAC Fms. area resulted 6.9% and 1.3%, respectively (). Despite the similarity of such formations, the smaller value of LIG obtained for MAC Fm. (, ) could be attributable to the relatively less intense rainfall that hit the area where this formation crops out (340–380 mm in 24 h, SW area; ). In contrast, the higher LIG value for GOT Fm. is probably linked to the high rainfall intensity (340–500 mm in 24 h, NE area) recorded in the GOT area (). Except for the GOT Fm., all geological formations showed an LIG lower than the LI B ().
Table 1. Statistical analysis of the relationship between environmental parameters subdivided into classes and the 25 October 2011 shallow landslides.
The correlation between landslide distribution and bedrock attitude highlights that 40.1%, 32.2% and 27.2% of landslides occurred where the rock layers dip obliquely, upslope and downslope, respectively. This result is consistent with the prevalent oblique orientation of slopes in accordance with the main trending tectonic structures (NW–SE direction). Finally, it should be remarked that the largest landslides activated where the rock layers are dipping downward, as also observed by CitationD’Amato Avanzi et al. (2004) in the southern Apuan Alps.
4.3. Landslides and land use
In order to compare shallow landslide distribution and land use, the Pogliaschina T. basin was subdivided into three main land use categories: woodland (92.8% of the basin), agricultural (5.6%) and urban (1.6%) areas. 83.3%, 14.9% and 1.8% of the 2011 shallow landslides fall into these three categories, respectively. The woodland area was further subdivided into four classes: chestnuts (45.6% of the whole area), pine woodlands (21.5%), mixed hardwoods and conifers (mainly chestnuts and pines, 16.8%) and other types (9.1%). 30.2%, 20.4% and 16.4% of the shallow landslides fall into the first three classes, respectively (). Chestnut (Castanea sativa) and pine (mainly Pinus pinaster) woodlands are in a poor condition, due to low maintenance and heavy parasite infestation: chestnuts and pines are attacked by the chestnut gall wasp (Dryocosmus kuriphilus) and by the cochineal (Matsucoccus feytaudi), respectively. Landslide density is greater in areas of heavy infested pine woodlands than in less infested areas. Thus, the M. feytaudi infestation probably induces a negative influence on slope stability, weakening the trees and reducing root reinforcement of the soil. Further studies on this topic are however necessary and of great importance in order to confirm this hypothesis and to plan countermeasures.
Agricultural areas, made of dry stone wall terraces or grassy embankments, include vineyards (0.6% of the basin area), olive groves (0.9%), abandoned olive groves (0.1%), mixed vineyards and olive groves (0.4%) and meadows (3.6%). Although agricultural areas cover only 5.6% of the Pogliaschina T. basin, 14.9% of the shallow landslides occurred in this land use class (). In general, the landslide index for land use (LILU, ratio between the landslide area in each land use class and the total land use class area) of the agricultural areas is higher than that of other land use classes (). The LILU of mixed vineyards and olive groves, abandoned olive groves, vineyards and olive groves are 16.2%, 14.1%, 13.7% and 6.6% (), respectively. Otherwise, chestnuts, mixed hardwoods and conifers, and pine woodlands are characterized by low LILU values: respectively of 2.1%, 3.4% and 3.8% ().
The great variability of the LILU values for each class indicates that land use as significant landslide predisposing factor. In particular, terraced agricultural areas are more prone to landsliding than woodlands. This could be explained by considering that sub-horizontal surfaces of terraces generally hinder runoff in favour of water infiltration. Therefore, during intense rainfall and in the absence of maintenance, the soil of a terraced area can become saturated more easily than on a natural slope, so causing instability (CitationCevasco, Brandolini, Scopesi, & Rellini, 2013; CitationCrosta, Dal Negro, & Frattini, 2003; CitationTarolli, Preti, & Romano, 2014).
Significant differences in terms of LILU were found between cultivated and abandoned terraced areas: the abandoned olive groves showed a double LILU than the cultivated ones (, ), probably attributable to a lack of or poor maintenance of the terraced areas. Landslide susceptibility of the abandoned terraced areas higher than that of the cultivated ones is consistent with the observations of CitationCevasco et al. (2014) in the Vernazza Basin (Cinque Terre area) during the same meteorological event.
4.4. Landslides and morphometric parameters
The shallow landslide distribution was also compared with four fundamental morphometric parameters: aspect, slope angle, plan curvature and profile curvature, obtaining the LI for each morphometric parameter. With regards to aspect, most landslides occurred on the southeast (21.9%) and south (19.0%) facing slopes ((a) and (a)). Few landslides involved north (11.9%) and northeast (7.1%) facing slopes ((a)). This distribution is not directly attributable to aspect, but is probably indirectly influenced by other parameters, such as slope angle and land use. Indeed, the highest landslide susceptibility of the south facing slopes may be related to their steepness and the diffusion of terraced areas ((a)).
Only landslide indexes (LIA) for the SE (4.4%), SW (4.3%), W (3.7%) and S (3.4%) classes are higher than the LIB. The difference between these LIA and the LIA for the remaining aspect classes is not high, likely highlighting a poor correlation between aspect and landslide distribution. Therefore, as already observed for the Sicily Region (Italy) by CitationManzo, Tofani, Segoni, Battistini, and Catani (2013), the influence of aspect may be negligible in a landslide susceptibility assessment.
In order to investigate the influence of slope angle on landslide occurrence, the study basin was subdivided into nine slope angle classes (≤15°, 16–20°, 21–25°, 26–30°, 31–35°, 36–40°, 41–45°, 46–50°, ≥51°). The slope angle distribution in these classes is largely homogeneous, although the class 31–35° is notably different ((b)). This homogeneity is reflected in the shallow landslide distribution, with the 31–35° class including the highest percentage of source areas (24.3%; (b)). Only 6.3% of source areas fall into the slope angle classes ≤15° and ≥51°, demonstrating that landslides rarely occur on gentle slopes (smaller shear stress) and on very steep slopes (soil and debris are thin or rocky outcrops prevail).
The landslide index for slope angle (LIS) classes of 16–20°, 21–25°, 26–30°, 31–35° and 36–40° are 4.2%, 3.4%, 3.3%, 3.9% and 3.0% ((b)), respectively. The other classes, which have LIS<3.0% ((b)), show a poor correlation with the landslide distribution.
The relationship between shallow landslides and plan and profile curvature was also analysed. Concave slopes (PLC < 0) include 39.2% of landslides and their landslide index (LIPLC<0) is 5.1% ((c)). The LIPLC=0 (2.1%) and LIPLC>0 (2.2%) values are lower than the LIB. Consequently, the slopes with PLC = 0 and PLC > 0 are less susceptible to landsliding than those with PLC < 0.
The landslide distribution in the three profile curvature classes (PRC < 0, PRC = 0, PRC > 0) is homogenous ((d); ), although the class PRC > 0 (convex slopes; 34.6%) is slightly higher. The PRC < 0, PRC = 0 and PRC > 0 classes have similar LIPRC values: 3.4%, 2.7% and 3.0% ((d); ), respectively, highlighting a poor correlation of this parameter with landsliding.
Ultimately, the areas most prone to shallow landslide initiation are located in the upper part of the slopes and are characterized by a concave morphology. Comparable results were obtained by several authors (CitationCampbell, 1975; CitationD’Amato Avanzi et al., 2004; CitationDietrich, Reneau, & Wilson, 1987; CitationReneau & Dietrich, 1987; CitationSmith, 1988), which asserted that concave slopes are more susceptible to shallow landsliding, because of running water concentration and the build-up of pore pressure. In this study, the analysis and comparison of landslide source areas and types of movement with the plan curvature parameter highlighted that about 41% of debris slide-flows originated on concave slopes, while only 29% of debris slides were generated in the same category.
5. Conclusions
On 25 October 2011 a heavy rainstorm (almost 600 mm in 24 h) hit eastern Liguria and northwestern Tuscany, triggering hundreds of shallow landslides in the Pogliaschina T. basin. 658 landslides were subsequently detected, mapped and analysed during this work and are presented on the Main Map.
A landslide database including the geological–geomorphological and land use features of landslide source areas was also implemented, with the aim of analysing the influence of some terrain factors (geology, structural setting, land use, aspect, slope angle and plan and profile curvature) on landslide occurrence.
The bedrock lithology appears to be an important factor in the localization of shallow landslide source areas. In fact, 90.7% of shallow landslides were triggered on soil covering arenaceous formations (GOT and MAC Fms.). In particular, GOT Fm. showed the highest LIG (6.9%). This value may be affected by the high values of rainfall (340–500 mm in 24 h) recorded on the areas underlain by GOT Fm. The structural setting of bedrock seems to influence both the landslide distribution and size. The largest landslides occurred where bedrock layering dips downslope.
The comparison between landslide inventory and land use indicates that terraced agricultural areas are more prone to landsliding than woodland. In fact, although the majority of landslides (83.3%) involved the latter, the highest LILU values were found for vineyards and mixed vineyards and olive groves (13.7–16.2%). The degree of maintenance of terraced agricultural areas seems to play an important role on landslide susceptibility: the LILU of the abandoned olive groves (14.1%) is about double than that of cultivated olive groves (6.6%).
The correlation between shallow landslides and slope morphology of the source areas indicates that the failures mainly initiated on concave slopes (39.2% of landslides; LIPLC<0 = 5.1%).
Moreover, the landslide source areas were mainly located on southeast (21.9%) and south (19.0%) facing slopes. This is not attributable directly to aspect, but is probably related to the indirect influence of slope angle and land use, since south and southeast facing slopes are very steep and covered by terraced areas.
Although it was possible to appreciate some differences in landslide distribution, slope angle, aspect and profile curvature are not strongly associated with landslide occurrence, as no significant variation of LI was observed. Otherwise, geology, land use and plan curvature showed an important correlation with shallow landslides, as they have a great difference of LI among the classes.
In conclusion, this paper (and Main Map) provides basic information on the main predisposing factors controlling shallow landslide occurrence. This work can represent a useful tool for landslide susceptibility and hazard assessment in the study area. Statistical methods for landslide susceptibility mapping of the study area are currently being performed.
Further investigations will be performed in order to quantify the geotechnical and hydrogeological parameters (cohesion, friction angle, hydraulic conductivity) of soils involved in landsliding, which play a key role in slope stability (CitationD’Amato Avanzi, Galanti, Giannecchini, Lo Presti, & Puccinelli, 2013) and will be used in physically based models to produce landslide susceptibility maps of the study area. The comparison between susceptibility maps obtained using statistical and physically based methods will contribute to an understanding of the features of shallow landslide source areas. Moreover, rainfall thresholds for shallow landslide initiation are under study and will help to improve forecasting and warning systems. Finally, all these studies will help to identify risk scenarios for shallow landslide occurrence in the study area
Software
Georeferencing and digitization, landslide database, statistical analysis, geomorphological and geological maps were entirely developed using Esri ArcGIS® 10. Map Layout and final editing were performed using CorelDraw®X6.
The influence of geological-morphological and land use settings on shallow landslides in the Pogliaschina T. basin (northern Apennines, Italy).pdf
Download PDF (6.5 MB)Acknowledgements
We are grateful to the Liguria Regional Administration for providing the topographic raster maps, post-event aerial photographs and orthophotos, 5×5 digital elevation model and land use map. We also thank the Reviewers for their insightful comments and suggestions that greatly improved this paper.
Disclosure statement
No potential conflict of interest was reported by the authors.
ORCID
C. Bartelletti http://orcid.org/0000-0001-9045-3625
R. Giannecchini http://orcid.org/0000-0003-0447-3086
G. D’Amato Avanzi http://orcid.org/0000-0002-7502-1637
Y. Galanti http://orcid.org/0000-0002-3346-515X
Additional information
Funding
References
- Abbate, E. (1969). Geologia delle Cinque Terre e dell’entroterra di Levanto (Liguria Orientale). Memorie della Società Geologica Italiana, 8, 923–1014 [in Italian].
- Abbate, E., Fanucci, F., Benvenuti, M., Bruni, P., Cipriani, N., Falorni, P., … Vannucchi, P. (2005). Carta Geologica d'Italia alla scala 1:50.000, Foglio 248 La Spezia. Serv. Geol. d’Italia. Roma [in Italian].
- Amanti, M., Bertolini, G., Cara, P., Chiessi, V., De Nardo, M. T., Martini, M. G., … Ventura, R. (2004). Allegato 2 – Il database cartografico. Serv. Geol. d’Italia, Roma, pp. 7 [in Italian].
- Amanti, M., Bertolini, G., Cara, P., Chiessi, V., De Nardo, M. T., Martini, M. G., … Ventura, R. (2005). Allegato 3 – Il database alfanumerico. Serv. Geol. d’Italia, Roma, pp. 62 [in Italian].
- Bartelletti, C., D’Amato Avanzi, G., Galanti, Y., Giannecchini, R., & Mazzali, A. (2015). Assessing shallow landslide susceptibility by using the SHALSTAB model in Eastern Liguria (Italy). Rendiconti Online - Società Geologica Italiana, 35, 17–20. doi: 10.3301/ROL.2015.53
- Bernini, M., & Lasagna, S. (1988). Rilevamento geologico e analisi strutturale del bacino dell’alta Val di Magra tra M. Orsaro e Pontremoli (Appennino settentrionale). Atti della Società Toscana di Scienze Naturali, Memorie, Serie A, 95, 139–183 [in Italian].
- Bordoni, M., Persichillo, M. G., Meisina, C., Cevasco, A., Giannecchini, R., D’Amato Avanzi, G., … Zizioli, D. (2015). Developing and testing a data-driven methodology for shallow landslide susceptibility assessment: Preliminary results.Rendiconti Online - Società Geologica Italiana, 35, 25–28. doi: 10.3301/ROL.2015.55
- Bortolotti, V., Principi, G., Abbate, E., Chiari, M., Aiello, I. W., Mannori, G., … Pandeli, E. (2011). Carta Geologica d’Italia alla scala 1:50.000, Foglio 232 Sestri Levante. Serv. Geol. d’Italia. Roma [in Italian].
- Campbell, R. H. (1975). Soil slips, debris flows and rainstorms in the Santa Monica Mountains and Vicinity, Southern California (U.S. Geological Survey Professional Paper 851).
- Cevasco, A., Brandolini, P., Scopesi, C., & Rellini, I. (2013). Relationships between geo-hydrological processes induced by heavy rainfall and land-use: The case of 25 October 2011 in the Vernazza catchment (Cinque Terre, NW Italy). Journal of Maps, 9, 289–298. doi: 10.1080/17445647.2013.780188
- Cevasco, A., Diodato, N., Revellino, P., Fiorillo, F., Grelle, G., & Guadagno, F. M. (2015). Storminess and geo-hydrological events affecting small coastal basins in a terraced Mediterranean environment. Science of the Total Environment, 532, 208–219. doi: 10.1016/j.scitotenv.2015.06.017
- Cevasco, A., Pepe, G., & Brandolini, P. (2014). The influences of geological and land use settings on shallow landslides triggered by an intense rainfall event in a coastal terraced environment. Bulletin of Engineering Geology and the Environment, 73, 859–875. doi: 10.1007/s10064-013-0544-x
- Cevasco, A., Pepe, G., D’Amato Avanzi, G., & Giannecchini, R. (2015). A study on the November 2014 intense rainfall and the related landslides in the lower Lavagna valley (eastern Liguria).Rendiconti Online - Società Geologica Italiana, 35, 66–69. doi: 10.3301/ROL.2015.65
- Consorzio LaMMA. (2012). Studio sulla stabilità dei versanti e individuazione degli elementi di maggior rischio di dissesto attraverso il confronto della cartografia regionale di stabilità dei versanti e dei depositi superficiali con la banca dati delle aree instabili ricavata dall’analisi dei piani strutturali comunali – completamento su tutto il territorio regionale. Technical Report, May 2012, 26 pp.
- Crosta, G. B., Dal Negro, P., & Frattini, P. (2003). Soil slips and debris flows on terraced slopes. Natural Hazards and Earth System Sciences, 3, 31–42. doi: 10.5194/nhess-3-31-2003
- Cruden, D. M., & Varnes, D. J. (1996). Landslide type and processes. In A. K. Turner, & R. L. Schuster (Eds.), Landslides: Investigation and mitigation (pp. 36–75). Special Report 247, Transportation Research Board, National Research Council. Washington: National Academy Press.
- D’Amato Avanzi, G., Galanti, Y., Giannecchini, R., & Bartelletti, C. (2015). Shallow landslides triggered by the 25 October 2011 extreme rainfall in eastern Liguria (Italy). In G. Lollino, D. Giordan, G. B. Crosta, J. Corominas, R. Azzam, J. Wasowski, & N. Sciarra (Eds.), Engineering geology for society and territory (Vol. 2, pp. 515–519). Cham: Springer International Publishing.
- D’Amato Avanzi, G., Galanti, Y., Giannecchini, R., Duchi, S., Lo Presti, D., & Marchetti, D. (2013). DP test in geotechnical characterization of shallow landslides source area: Results and perspectives. In C. Margottini, P. Canuti & K. Sassa (Eds.), Landslide science and practice: Landslide inventory and susceptibility and hazard zoning (Vol. 1, pp. 249–255). Berlin: Springer-Verlag.
- D’Amato Avanzi, G., Galanti, Y., Giannecchini, R., Lo Presti, D., & Puccinelli, A. (2013). Estimation of soil properties of shallow landslide source areas by dynamic penetration tests: First outcomes from Northern Tuscany (Italy). Bulletin of Engineering Geology and the Environment, 72, 609–624. doi: 10.1007/s10064-013-0535-y
- D’Amato Avanzi, G., Galanti, Y., Giannecchini, R., Mazzali, A., & Saulle, G. (2013). Remarks on the 25 October 2011 rainstorm in eastern Liguria and northwestern Tuscany (Italy) and the related landslides. Rendiconti Online - Società Geologica Italiana, 24, 76–78.
- D’Amato Avanzi, G., Galanti, Y., Giannecchini, R., & Puccinelli, A. (2013). Fragility of territory and infrastructures resulting from rainstorms in Northern Tuscany (Italy). In C. Margottini, P. Canuti & K. Sassa (Eds.), Landslide science and practice: Risk assessment, management and mitigation (Vol. 6, pp. 239–246). Berlin: Springer-Verlag.
- D’Amato Avanzi, G., Giannecchini, R., & Puccinelli, A. (2002). I movimenti franosi del novembre 2000 nella provincia di Lucca: osservazioni preliminari. In Il dissesto idrogeologico: inventario e prospettive (Vol. 181, pp. 365–377). Roma: Accademia Nazionale dei Lincei. [in Italian].
- D’Amato Avanzi, G., Giannecchini, R., & Puccinelli, A. (2004). The influence of the geological, geomorphological settings on shallow landslides. An example in a temperate climate environment: The June 19,1996 event in north-western Tuscany (Italy). Engineering Geology, 73, 215–228. doi: 10.1016/j.enggeo.2004.01.005
- Dietrich, W. E., Reneau, S. L., & Wilson, C. J. (1987). Overview: “zero-order basins” and problems of drainage density, sediment transport and hillslope morphology. Paper presented at the proceedings of international symposium on erosion and sedimentation in the Pacific Rim, 3–7 August 1987, Corvallis, Oregon. IAHS pub, 165, 27–37.
- Galanti, Y., Giannecchini, R., D’Amato Avanzi, G., Barsanti, M., & Benvenuto, G. (2016). Rainfall thresholds for triggering shallow landslides in Vara Valley (Liguria, Italy). In S. Aversa, L. Cascini, L. Picarelli, & C. Scavia (Eds.), Landslides and engineering slopes. Experience, theory and practice (Vol. 2, pp. 943–950). Rome: Associazione Geotecnica Italiana.
- Galve, J. P., Cevasco, A., Brandolini, P., & Soldati, M. (2015). Assessment of shallow landslide risk mitigation measures based on land use planning through probabilistic modelling. Landslides, 12, 101–114. doi: 10.1007/s10346-014-0478-9
- Giannecchini, R., & D’Amato Avanzi, G. (2012). Historical research as a tool in estimating the flood/landslide hazard in a typical small alpine-like area: The example of the Versilia River basin (Apuan Alps, Italy). Physics and Chemistry of the Earth, Parts A/B/C, 49, 32–43. doi: 10.1016/j.pce.2011.12.005
- Giannecchini, R., Galanti, Y., & D’Amato Avanzi, G. (2012). Critical rainfall thresholds for triggering shallow landslides in the Serchio River Valley (Tuscany, Italy). Natural Hazards and Earth System Science, 12, 829–842. doi: 10.5194/nhess-12-829-2012
- Giannecchini, R., Galanti, Y., D’Amato Avanzi, G., & Barsanti, M. (2016). Probabilistic rainfall thresholds for triggering debris flows in a human-modified landscape. Geomorphology, 257, 94–107. doi: 10.1016/j.geomorph.2015.12.012
- Manzo, G., Tofani, V., Segoni, S., Battistini, A., & Catani, F. (2013). GIS techniques for regional scale landslide susceptibility assessment: The Sicily (Italy) case study. International Journal of Geographical Information Science, 27(7), 1433–1452. doi: 10.1080/13658816.2012.693614
- Mondini, A. C., Viero, A., Cavalli, M., Marchi, L., Herrera, G., & Guzzetti, F. (2014). Comparison of event landslide inventories: The Pogliaschina catchment test case, Italy. Natural Hazards and Earth System Science, 14, 1749–1759. doi: 10.5194/nhess-14-1749-2014
- Persichillo, M. P., Bordoni, M., Meisina, C., Bartelletti, C., Barsanti, M., Giannecchini, R., … Galve, J. P. (2016). Shallow landslides susceptibility assessment in different environments. Geomatics, Natural Hazards and Risk. doi:10.1080/19475705.2016.1265011
- Puccinelli, A., D’Amato Avanzi, G., & Perilli, N. (2015a). Carta Geologica d’Italia alla scala 1:50.000, Foglio 233 Pontremoli. Serv. Geol. d’Italia. Roma [in Italian].
- Puccinelli, A., D’Amato Avanzi, G., & Perilli, N. (2015b). Note illustrative della Carta Geologica d’Italia alla scala 1:50.000, Foglio 233 Pontremoli. Serv. Geol. d’Italia. Roma, pp. 127 [in Italian].
- Raggi, G. (1985). Neotettonica ed evoluzione paleogeografica plio-pleistocenica del bacino del Fiume Magra. Memorie della Società Geologica Italiana, 30, 35–62 [in Italian].
- Regione Liguria. (2013). Carta di uso del suolo scala 1:10000 - edizione 2012 [in Italian].
- Reneau, S. L., & Dietrich, W. E. (1987). The importance of hollows in debris flow studies; examples from Marin County, California. Reviews in Engineering Geology, 7, 165–180. doi: 10.1130/REG7-p165
- Sacchi, A. J. (2012). Analisi meteorologica degli eventi alluvionali eccezionali tra l’alta toscana e l’estremo levante ligure dal 2009 al 2011. Atti Della Società Toscana di Scienze Naturali, Memorie, Serie A, 117–119, 75–88.
- Smith, T. C. (1988). A method for mapping relative susceptibility to debris flows with an example from San Mateo County. In S. D. Ellen, & G. F. Wieczorek (Eds.), Landslides, floods, and marine effects of the storm of January 3-5, 1982, in the San Francisco Bay Region, California (Vol. 1434, pp. 185–203). Washington: U.S. Geological Survey Professional Paper.
- Tarolli, P., Preti, F., & Romano, N. (2014). Terraced landscapes: From an old best practice to a potential hazard for soil degradation due to land abandonment. Anthropocene, 6, 10–25. doi: 10.1016/j.ancene.2014.03.002
- Van Westen, C. J. (2000). The modelling of landslide hazards using GIS. Surveys in Geophysics, 21, 241–255. doi: 10.1023/A:1006794127521
- Van Westen, C. J., Castellanos, E., & Kuriakose, S. L. (2008). Spatial data for landslide susceptibility, hazard, and vulnerability assessment: An overview. Engineering Geology, 102(3), 112–131. doi: 10.1016/j.enggeo.2008.03.010
- Zhou, C. H., Lee, C. F., Li, J., & Xu, Z. W. (2002). On the spatial relationship between landslides and causative factors on Lantau Island, Hong Kong. Geomorphology, 43, 197–207. doi: 10.1016/S0169-555X(01)00130-1