ABSTRACT
This paper presents the results of a geomorphological investigation of Mount Akdag landslide complex, located on the Western Taurus range, SW Turkey. The landslide, resulting in the collapse of a 5 km segment of Mount Akdag, covers an area of 9.8 km2 and has a volume of about 3 × 108 m3. The 1:15,000 scale geomorphological map (Main Map) of the Akdag landslide presented here was produced from a combination of field studies and unmanned aerial vehicle-based DSM, including aerial-photo interpretation and geomorphometric analysis of landforms. The map shows contemporary and past geomorphological hillslope processes and landforms associated with the landslide complex. Our new map suggests that the Akdag landslide was a complex rockslide consisting of multiple landslide types that developed through various movements. We found that 43 highly developed and active mass movements on the main body and side slopes of the landslide made a major contribution to the sediment input. The sediment produced in the upstream severely threatens the touristic site of Saklikent Canyon in the lower catchment via an increasing magnitude of torrents. This map will provide important data for local and national administrators regarding monitoring up-to-date mass-wasting processes, understanding the landslide and its related sedimentary dynamics, and implementing risk assessment and necessary precautions.
1. Introduction
Mount Akdag in the Western Taurus range represents a remarkably dynamic terrain in terms of past and recent geomorphological processes. Multiple factors have played a role in the geomorphological formation of Mount Akdag. Among these, karst, glacial, tectonic and gravitational processes are especially important. Particularly in areas above 2000 m elevation, landforms belonging to karst and glacial geomorphology are observed in an interleaved manner. Climate changes in the Quaternary have also had important implications for these polygenetic topographies (CitationBayrakdar, 2012; CitationSarikaya, Ciner, Haybat, & Zreda, 2014). In this respect, the geomorphological evolution of Mount Akdag is quite complex.
The region is highly active in terms of sedimentary dynamics and the geomorphological processes are generally dominated by mass movements. Landslides are observed on almost all the hillslopes of the mountain but their activity is especially prevalent on the southern slope. The complex Akdag landslide that developed on the southern slope is possibly the largest landslide of its type in the Western and Central Taurides. The landslide resulted in the collapse of a 5 km segment of Mount Akdag and covers an area of 9.8 km2. This landslide complex presents significant challenges to geohazard management in the region. The magnitude of geohazard events is increasing due to the delivery of high debris input to the river system by this landslide during the frequent fatal torrents observed in the region. In previous years, there have been many catastrophic torrents in Saklikent Canyon, which is an important touristic site located downstream of the catchment. Thus, slope failures and torrent hazards pose a serious risk to tourists and the local population. In this respect, long-term monitoring of the landslide’s activity and the quantity and transfer of sediment are necessary.
Mapping of the secondary and individual landslides provides a sound basis for future geomorphological investigation of the dynamics and hazard assessment of complex landforms such as Akdag landslide. In order to better assess potential hazards and risks, a number of issues within the mapped area are worthy of further investigation. These include (i) revealing landslide dynamics by monitoring the area using multi-temporal unmanned aerial vehicle (UAV)-based high-resolution digital surface models (DSMs), (ii) performing slope stability analyses taking into account the long-term behavior of the landslide, (iii) quantifying the material generated by the landslide and transferred to the river channel by carrying out runout and entrainment analyses of the released debris input, and (iv) implementing precautionary measures and mitigation studies in consideration of the risks to the downstream catchment.
In this study, we present a 1:15,000 geomorphology map of the Mount Akdag landslide (Main Map), which is in the Western Taurus range, Turkey. By compiling a detailed geomorphological map of the landslide, we aim (1) to contribute to a better understanding of the sedimentary dynamics and landscape evolution of the area through mapping the multiple widespread mass movements and (2) to reduce possible hazards to the public and infrastructure.
2. Geographical, geological and climatic setting of study area
The landslide is located on the southern slope of Mount Akdag (36.54°N, 29.57°E, 3016 m a.s.l.) (). This mountainous zone contains the second highest peak (Uyluk, 3014 m a.s.l.) of the Western Taurus range. Landforms belonging to glacial morphology can be observed up to 1900 m a.s.l. as Mount Akdag was exposed to glaciation in the past. Glacial thickness and expansion in the paleo-karst depressions have been widely compared to similar altitudes in nearby glacial mountains (CitationBayrakdar, 2012). As a result, seven flat-bottomed glacial valleys developed on Mount Akdag. According to Optically Stimulated Luminescence dating and cosmogenic 36Cl surface exposure dating of the side and bottom moraines in these valleys, the last glacial maximum was found to correspond to the Würm II (17–21 ka) (CitationBayrakdar, 2012; CitationSarikaya et al., 2014).
Figure 1. Location map of the study area and 3D view of the Akdag landslide area. Location of landslides on eastern and southern slopes of Mount Akdag were identified from CitationDuman, Can, and Emre (2011).
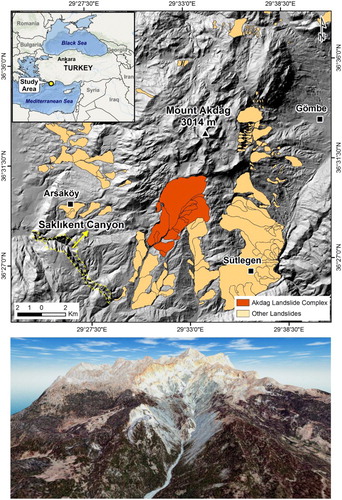
From north to south, Mount Akdag is composed mainly of Jurassic-Cretaceous Lycian allochthonous carbonates on top of the Beydag autochthonous carbonate platform (CitationErsoy, 1990; CitationSenel, 1997). The allochthonous units were emplaced by Alpine Orogenesis during the Late Mesozoic and Cenozoic (CitationGorur & Tuysuz, 2001) (). These allochthonous units are called the Lycian nappes and display a different geo-environmental character from adjacent areas (CitationErsoy, 1990; CitationSenel, 2004). After the end of napping movements in the Mid-Miocene (Langhian), the paleotectonic period ended and the neotectonic period began in the study area (CitationErsoy, 1990; CitationSchildgen et al., 2012).
Figure 2. Geology map of the study area (modified after CitationSenel, 1997).
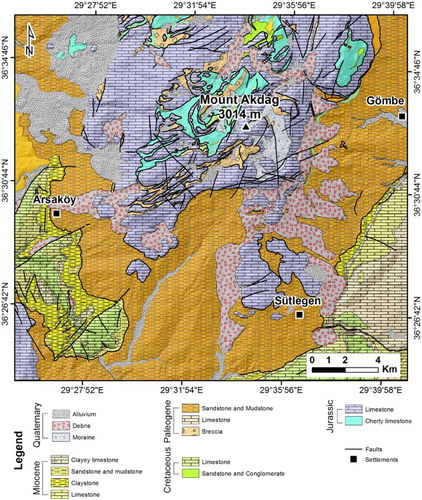
The lithology and structural geology of the area exert a strong influence on the morphology. Mount Akdag, which is mainly composed lithologically of carbonate units, is located in the Taurus range, where karstification was effective before, during and after the glacial period. Karstification in the region continued until the present, despite disruptions in areas where glaciation was effective. There are many dolines and uvalas in these karstic areas which were previously glaciated. Presently, these karst depressions constitute an underground drainage system through the dolines on their floors. Besides the glacial and karst geomorphology, mass movements on hillslopes play an important role in the present geomorphological appearance of Mount Akdag. The mass movements are concentrated particularly on the eastern and southern slopes, causing an asymmetry between the eastern and western hillslopes ().
A Mediterranean climate is observed at lower altitudes of the southern slopes. In contrast, at high elevations and on northern slopes of the mountains, the climate shows a terrestrial transition character (CitationSaris, Hannah, & Eastwood, 2010; CitationTurkes, 1996; CitationTurkes & Erlat, 2005). The main reason for climate differences between south and north is the topographic elevation. The yearly average temperature in mountainous areas above 2500 m a.s.l. is 4°. While the yearly average temperature in areas below 2000 m a.s.l. is 10° in the south and 8° in the north. Yearly precipitation reaches about 1100 mm in areas above 2000 m a.s.l but decreases to 670 mm in areas below 1000 m a.s.l.
3. Data and methods
The geomorphological map of Mount Akdag was formed from interpretation of UAV-based high-resolution orthophoto-mosaics and DSM and DSM derivatives, together with detailed field surveys ((a–e)). Airborne digital photographs over the landslide were acquired between 6 and 12 August 2015 using an autonomous UAV system. Images of the study area were obtained from 21 flights with the UAV. A total of 4666 photos were taken. For each flight, a standardized image resolution (∼10 cm) and 80% (lateral) and 75% (longitudinal) overlap ratios were considered. Esri ArcGIS software was used in the production of the geomorphological map which is based on the UTM European 1950 coordinate system and has a scale of 1:15,000. The base images considered for making the maps were UAV-based orthophoto-mosaics and high-resolution (∼1 m) GeoEye-2 images. These images were used to complement missing data in the north-western portion of the source area due to unfavorable weather conditions occurring during the research ((a)). The UAV-based DSM produced for the study area was resampled to 1 m resolution.
Figure 3. (A) Boundary of landslide (transparent orange color) and UAV-based DSM. Thematic maps derived from UAV-obtained DSM analysis of the sample area (yellow rectangle in (A)); (B) 3D view of the NE section of Akdag landslide obtained from UAV-based orthophoto-mosaics and DSM, (C) Hillshade, (D) Slope, and (E) Aspect.
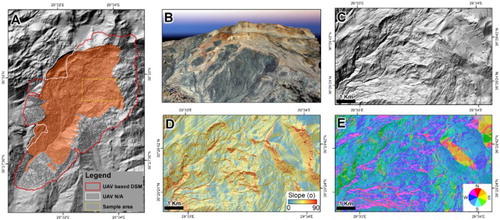
In areas where DSM data were lacking, 10 m contour lines obtained from 1:25,000 scale topographic maps were used. Also, field-based mapping was applied intensively in areas where we could not obtain UAV-based airborne digital photographs. In the interpretation, we made use of diagnostic features such as tone, texture, pattern and shape from the UAV-based orthophoto-mosaics. Additionally, we used topographic slope, aspect and hillshade features, which are first-order terrain derivatives calculated from the DSM, to obtain morphometric data and assist with geomorphological interpretation ((a–e)). Each geomorphological unit was digitized by evaluating all data in ArcGIS.
The digitized geomorphological units were validated by ground data from extensive field observations conducted during three different periods between 2013 and 2015. The rock falls on the main landslide mass, the debris flows and the secondary landslides were mapped in detail during these field studies. In addition, data regarding the main and secondary fracture systems were collected and inserted on the maps. Validation for inaccessible steep areas was conducted using ground photos and oblique photos obtained from a quadcopter drone. The geology map produced by CitationSenel et al. (1989) was used to obtain structural and lithological data for the geomorphology map. This geology map was used for determining the unit boundaries before the Quaternary. The limits of the geomorphological boundaries belonging to Quaternary and the surface geology were established by interpreting remote sensing images and field data.
The mapped geomorphological features were classified according to the primary process systems that shaped this landscape. In this regard, the geomorphological features were grouped in the key according to whether they were generated and dominated by processes associated with ‘Structural’, ‘Gravitational’ and ‘Fluvial’ action. Among these main groups, gravitational landforms were also divided into two subgroups. The first of these was geomorphological subunits related to the Akdag landslide. The other group included landforms not related to the landslide and/or morphological features related to secondary mass movements formed on the main body of the Akdag landslide. In the mapping, the lithology is provided as a separate layer. The lithological units are defined as two separate subgroups, namely, Quaternary deposits and older rock types.
4. Mapping results and discussion
The Akdag landslide is a complex landslide including multiple landslide types that developed through multiple activities. The length of the landslide from the source area to the toe is 4.5 km (). The surface area of the landslide is about 9.8 km2. The landslide formed at the contact points of the Jurassic limestone, Paleogene sandstone and claystone units. It is one of the biggest bedrock landslides known in the West and Central Taurus range. The landslide was primarily affected by structural features such as cracks, fissures and faults; these can be clearly observed in the source area.
Figure 4. Longitudinal cross-section of Akdag landslide. Yellow line on inset map shows the location of the cross-section.
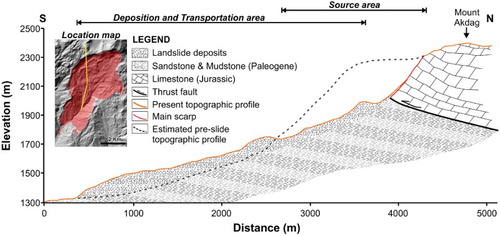
The multi-phase Akdag landslide was assessed geomorphologically together with younger landslides. Due to the development of many secondary mass movements over the main landslide body, Mount Akdag displays an intricate character. Two distinct mass movement sectors were identified within the Akdag landslide complex and are shown on the map. While the first is the main Akdag landslide, the second is composed of relatively young slope failures formed after the main landslide. The Akdag landslide has a very steep (72°) scarp with 300 m length ((a)). Many rock falls occurred on this main scarp, which corresponds to a thrust fault zone. Apart from the main scarp, one secondary scarp unit was also mapped in the landslide. These scarps are at the same elevation and located in the middle of the landslide body. The height and slope of these scarps belonging to the secondary slides are 60 m and 32°, respectively. A wide counterslope surface formed between the main and secondary scarps. In contrast to the general southern slope trends, this surface has an average 8° north-facing slope. All landslides below the counterslope level are highly active.
Figure 5. (A) Source area and main scarp of Akdag landslide. (B) Rill and gully channels developed on bodies of secondary slides and earthflows over dark gray clays. (C) Example of new landslide occurrence on the body of former slides.
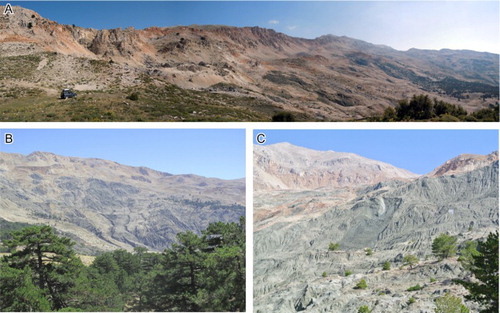
Not only is there a dense distribution of cracks and fissures in this area but springs are also apparent. Rill and gully channels developed on the earthflow bodies over the dark gray clays and fine sandy matrix levels of the Akdag landslide ((b)). Besides the old landslides, this type of terrain has areas where small-scale new slides and flows developed ((c)). The accumulation section of the Akdag landslide overspread a former mountainous valley system, which is thought to have had a narrow floor. The maximum width of the accumulation area, which expands from the tip of the landslide toe to the north east, is 1.12 km. The coarse debris and boulders in the accumulation area are dominantly limestone. The diameter ranges from a few meters to several tens of meters. Dimensions of the blocks decrease in size and height away from the source scarp. Materials from these boulders are transferred by Kara Creek ((a)). Due to the presence of varying-size blocks, cobbles and pebbles, the gradient and energy of this mountain catchment is high ((b)). The frequency of flash floods is high in Kara Creek, whose stream-bed narrows toward Saklikent Canyon. The transfer of material generated by the landslide generally increases in the spring and beginning of summer due to the sudden melting of snow.
Figure 6. (A) Upper part of Kara Creek, the conduit by which material is transferred downstream from the Akdag landslide complex. (B) Massive aggradation on the upper section of Kara Creek.
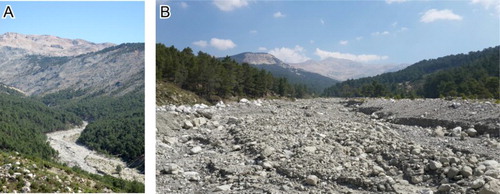
Forty-three different types (CitationCruden & Varnes, 1996) and sized secondary slope failures were mapped in the main body and flanks of the Akdag landslide. The type of these landslides was identified as slides, rockfalls and earth and debris flows. The landslides on the main body of the Akdag landslide are located just in front of the counter slope and their movement direction is toward the southern slopes. Transverse and radial cracks with distances of 10–290 m developed in the secondary slides situated on the main body of the landslide. The density of these cracks and fissures is high in the western part. The slope failures that developed outside the main body of the landslide are located in the accumulation area and on the side slopes of Kara creek. These landslides are generally of the slide type. In contrast to the landslides that formed on the main body, these are not secondary but individual slides. The sliding surface depth is higher especially on the western side slopes (>15 m) and the landslides formed here are mainly constituted of deep-seated slides. These slope movements are younger compared to ones that formed in the main landslide and are still very active. Besides structural factors, toe erosion also plays an important role in the development of these mass movements to the south of the main landslide.
In comparison with the very steep main scarp and average height of >250 m for the landslide and counterslope surfaces, the presence of the secondary landslides that developed in the main body of the landslide and in the two main scarps can typically be explained by relatively rapid movements related to multiple deep-seated gravitational processes (CitationCrosta, 1996; CitationCruden & Varnes, 1996; CitationFrodella, Morelli, Fidolini, Pazzi, & Fanti, 2014; CitationGeertsema, Clague, Schwab, & Evans, 2006; CitationHancox & Perrin, 2009; CitationHungr, Leroueil, & Picarelli, 2014; CitationZaruba & Mencl, 1982). Apart from the triggering mechanism, the internal structures of the hillslope and the geological setting primarily control the formation of this type of large gravitational slope deformation (CitationAmbrosi & Crosta, 2006; CitationBorrelli, Antronico, Gullà, & Sorriso-Valvo, 2014; CitationCrosta, Imposimato, & Roddeman, 2003; CitationHungr, Evans, & Hazzard, 1999; CitationKorup, 2005; CitationSacchini, Faccini, Ferraris, Firpo, & Angelini, 2016; CitationStrom & Korup, 2006; CitationWeidinger & Korup, 2009; CitationWeidinger et al., 2014). The correspondence of the Akdag landslide scarp to the thrust fault that forms the lithologic border of the Lycian nappes suggests that structural factors played a significant role in the formation of the landslide.
In addition to structural geological factors, the widespread distribution of karstic depressions and lithological differences has also had an influence on the development of the Akdag landslide. There are many uvalas and dolines in the carbonate units on the upper part of the scarp. The relatively permeable Jurassic limestone units provide water to the underlying, tectonically discordant and impermeable Paleogene sandstone and mudstone lithological units through the karstic depressions and fracture systems. We do not have sufficient information about the age and therefore the triggering factors of the landslide. However, considering that snow depth reaches 15–17 m in the karstic depressions in the upper parts of the landslide, we suggest that the percolation of water from the snow-melt to the underlying impermeable clayey units possibly influenced the formation of the landslide. In addition, the presence of active faults within a 20 km radius of the landslide points to seismic shaking together with high pore water pressure as a possible triggering factor for the landslide.
5. Conclusions
We presented here a 1:15,000 scale map that illustrates the Mount Akdag landslide complex. The geomorphological map was prepared by means of a combination of detailed field surveys, UAV-based high-resolution DSM and DSM products (hillshade, topographic slope and aspect layers) and geographic information system analysis, revealing the complex nature of the Akdag landslide complex, characterized by a very steep head scarp, dissected counterslopes and secondary and individual mass movements. Many cracks and fissures mapped in the landslide and behind the scarp, together with the fresh morphology of the secondary and individual mass movements, suggest that the activity level of the landslide is high.
The sinuous morphology of the main scarp of the landslide and the correspondence between the concave parts of the scarp with rockfall and secondary slides show that the landslide has a retrogressive character. Moreover, the landslide exhibits an enlarging tendency due to the individual landslides that have occurred on the side slopes to the south. These characteristics and the large amount of debris due to it being a mountainous catchment area increase the frequency of high magnitude fatal torrents that affect the touristic Saklikent Canyon located in the lower catchment. The heavy rainfall that triggered a torrent causing the death of two tourists and injuring nine more in July 2014 is only one example of the risks that the landslide poses. Thus, the geomorphological map produced by this study has an important role to play in monitoring the current mass-wasting processes that have developed over the landslide, understanding the landslide and its related sedimentary dynamics, implementing hazard and risk assessment of the landslide, and the taking of necessary measures by local and national administrations.
Software
The UAV data were collected using a SenseFly eBee. The DSM was generated using Postflight Terra 3D software. The geomorphological maps presented in this study were digitized and produced using Esri ArcGIS (10.1). Map layout and final editing were performed using Adobe Illustrator CS5.
Geomorphology of the Mount Akdag Landslide, Western Taurus Range (SW Turkey).pdf
Download PDF (4.2 MB)Acknowledgements
T. G. wishes to thank the Turkish Academy of Sciences for their support within the framework of the Distinguished Young Scientist Award Program (TÜBA-GEBIP). The authors thank Luigi Borrelli, Mihai Micu, and Chandra Jayasuriya for their instructive and thought-provoking comments on an earlier version of this manuscript and Mr G. H. Lee for proof-reading the final version.
Disclosure statement
No potential conflict of interest was reported by the authors.
ORCID
Tolga Görüm http://orcid.org/0000-0001-9407-7946
Ugur Avdan http://orcid.org/0000-0001-7873-9874
Additional information
Funding
References
- Ambrosi, C., & Crosta, G. B. (2006). Large sackung along major tectonic features in the Central Italian Alps. Engineering Geology, 83(1), 183–200. doi: 10.1016/j.enggeo.2005.06.031
- Bayrakdar, C. (2012). Akdag Kütlesi’nde (Batı Toroslar) Karstlaşma-Buzul ilişkisinin Jeomorfolojik Analizi (Unpublished PhD thesis). Istanbul University, Istanbul, p. 201. [in Turkish].
- Borrelli, L., Antronico, L., Gullà, G., & Sorriso-Valvo, G. M. (2014). Geology, geomorphology and dynamics of the 15 February 2010 Maierato landslide (Calabria, Italy). Geomorphology, 208, 50–73. doi: 10.1016/j.geomorph.2013.11.015
- Crosta, G. (1996). Landslide, spreading, deep seated gravitational deformation: Analysis, examples, problems and proposals. Geografia fisica e dinamica quaternaria, 19(2), 297–313.
- Crosta, G. B., Imposimato, S., & Roddeman, D. G. (2003). Numerical modelling of large landslides stability and runout. Natural Hazards and Earth System Science, 3(6), 523–538. doi: 10.5194/nhess-3-523-2003
- Cruden, D. M., & Varnes, D. J. (1996). Landslide types and processes. In A. K. Turner & R. L. Shuster (Eds.), Landslides: Investigation and mitigation. Transportation Research Board, Special Report 247, 36–75.
- Duman, T. Y., Can, T., & Emre, O. (2011). 1: 1,500,000 scaled Turkish landslide inventory map. Mineral Research & Exploration General Directorate Special Publication Series-27, Ankara, Turkey. p. 1.
- Ersoy, S. (1990). Batı Toros (Likya) naplarının yapısal öğelerinin ve evriminin analizi. Jeoloji Mühendisliği, 37, 5–16 [in Turkish].
- Frodella, W., Morelli, S., Fidolini, F., Pazzi, V., & Fanti, R. (2014). Geomorphology of the Rotolon landslide (Veneto Region, Italy). Journal of Maps, 10(3), 394–401. doi: 10.1080/17445647.2013.869666
- Geertsema, M., Clague, J. J., Schwab, J. W., & Evans, S. G. (2006). An overview of recent large catastrophic landslides in northern British Columbia, Canada. Engineering Geology, 83(1), 120–143. doi: 10.1016/j.enggeo.2005.06.028
- Gorur, N., & Tuysuz, O. (2001). Cretaceous to Miocene palaeogeographic evolution of Turkey: Implications for hydrocarbon potential. Journal of Petroleum Geology, 24, 119–146. doi: 10.1111/j.1747-5457.2001.tb00664.x
- Hancox, G. T., & Perrin, N. D. (2009). Green Lake Landslide and other giant and very large postglacial landslides in Fiordland, New Zealand. Quaternary Science Reviews, 28(11), 1020–1036. doi: 10.1016/j.quascirev.2008.08.017
- Hungr, O., Evans, S. G., & Hazzard, J. (1999). Magnitude and frequency of rock falls and rock slides along the main transportation corridors of southwestern British Columbia. Canadian Geotechnical Journal, 36(2), 224–238. doi: 10.1139/t98-106
- Hungr, O., Leroueil, S., & Picarelli, L. (2014). The Varnes classification of landslide types, an update. Landslides, 11(2), 167–194. doi: 10.1007/s10346-013-0436-y
- Korup, O. (2005). Large landslides and their effect on sediment flux in South Westland, New Zealand. Earth Surface Processes and Landforms, 30(3), 305–323. doi: 10.1002/esp.1143
- Sacchini, A., Faccini, F., Ferraris, F., Firpo, M., & Angelini, S. (2016). Large-scale landslide and deep-seated gravitational slope deformation of the Upper Scrivia Valley (Northern Apennine, Italy). Journal of Maps, 12(2), 344–358. doi: 10.1080/17445647.2015.1021393
- Sarikaya, M. A., Ciner, A., Haybat, H., & Zreda, M. (2014). An early advance of glaciers on Mount Akdağ, SW Turkey, before the global Last Glacial Maximum; insights from cosmogenic nuclides and glacier modeling. Quaternary Science Reviews, 88, 96–109. doi: 10.1016/j.quascirev.2014.01.016
- Saris, F., Hannah, D. M., & Eastwood, W. J. (2010). Spatial variability of precipitation regimes over Turkey. Hydrological Sciences Journal – Journal des Sciences Hydrologiques, 55(2), 234–249. doi: 10.1080/02626660903546142
- Schildgen, T. F., Cosentino, D., Bookhagen, B., Niedermann, S., Yıldırım, C., Echtler, H., … Strecker, M. R. (2012). Multi-phased uplift of the southern margin of the Central Anatolian plateau, Turkey: A record of tectonic and upper mantle processes. Earth and Planetary Science Letters, 317–318, 85–95. doi: 10.1016/j.epsl.2011.12.003
- Senel, M. (1997). 1/100.000 scale Turkish Geology Maps, Fethiye L-9 sheet. Mineral Research & Exploration General Directorate Press, No: 3. Turkey. p. 20.
- Senel, M. (2004). Stratigraphic and structural features of the Yesilbarak Nappe in Western Taurus range and its comparison with the similar units in SE Anatolia and North Cyprus. Bulletin of the Mineral Research and Exploration, 128(128), 1–26.
- Senel, M., Selcuk, H., Bilgin, Z. R., Sen, A. M., Karaman, T., Dincer, M. A., ... Bilgi, C. (1989). Gelogy of the Cameli (Denizli), Yesilova (Burdur), Elmali (Antalya) and near vicinity. Mineral Research & Exploration General Directorate Un-published Geology Reports, Ankara, Turkey. p. 110.
- Strom, A. L., & Korup, O. (2006). Extremely large rockslides and rock avalanches in the Tien Shan Mountains, Kyrgyzstan. Landslides, 3(2), 125–136. doi: 10.1007/s10346-005-0027-7
- Turkes, M. (1996). Spatial and temporal analysis of annual rainfall variations in Turkey. International Journal of Climatology, 16(9), 1057–1076. doi: 10.1002/(SICI)1097-0088(199609)16:9<1057::AID-JOC75>3.0.CO;2-D
- Turkes, M., & Erlat, E. (2005). Climatological responses of winter precipitation in Turkey to variability of the North Atlantic Oscillation during the period 1930–2001. Theoretical and Applied Climatology, 81(1–2), 45–69. doi: 10.1007/s00704-004-0084-1
- Weidinger, J. T., & Korup, O. (2009). Frictionite as evidence for a large Late Quaternary rockslide near Kanchenjunga, Sikkim Himalayas, India – Implications for extreme events in mountain relief destruction. Geomorphology, 103(1), 57–65. doi: 10.1016/j.geomorph.2007.10.021
- Weidinger, J. T., Korup, O., Munack, H., Altenberger, U., Dunning, S. A., Tippelt, G., & Lottermoser, W. (2014). Giant rockslides from the inside. Earth and Planetary Science Letters, 389, 62–73. doi: 10.1016/j.epsl.2013.12.017
- Zaruba, Q., & Mencl, V. (1982). Landslides and their control. Amsterdam: Elsevier, p. 324.