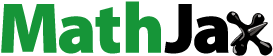
ABSTRACT
A geomorphic assessment of the Middle Fork John Day Watershed, Oregon, USA, was used to generate a hierarchical, map-based understanding of watershed impairments and potential opportunities for improvements. Specifically, we (1) assessed river diversity (character and behavior) and patterns of reach types (and their controls); (2) evaluated the geomorphic condition of the streams; (3) interpreted their geomorphic recovery potential; and (4) synthesized the above into a hypothetical, strategic management plan. Collectively, these maps can set bounds and provide realistic guidance for river rehabilitation, design and implementation efforts. Fifteen distinct reach types were identified, two-thirds of which are found along perennial streams. On the basis of a variety of geo-indicators, approximately two-thirds of all perennial stream reaches were found to be in ‘good’ geomorphic condition, whereas one-third had departed to ‘moderate’ and ‘poor’ condition. Departures from ‘good’ condition were primarily related to riparian vegetation removal, conversion of floodplain to agricultural land uses (farming and grazing), logging, and channel bed dredge mining for gold. Encouragingly, the majority of reaches classified as being in moderate geomorphic condition were found to have high recovery potential. While our geomorphic assessment has practical utility for informing physically realistic expectation management for efforts like salmonid habitat restoration, the maps themselves are the key vehicle for communicating and visualizing among stakeholders.
1. Introduction
Geomorphic mapping of channel patterns and reach types over entire drainage networks sets the stage for restoration and conservation planning (CitationBeechie & Imaki, 2014). In particular, efforts to recover threatened and endangered populations of anadromous salmon (Oncorhynchus spp.) and steelhead (O. mykiss) across the U.S. Pacific Northwest rely heavily on stream restoration intended to mitigate or reverse human impacts (CitationMontgomery, 2004). Those impacts, commonly referred to as the four H’s – hatchery practices, hydropower dams, harvest, and habitat loss/degradation – have spurred intense efforts to quantify the status and trends of fish populations (and their habitats), as well as to identify management actions that might improve population viability (CitationMann & Plummer, 2000; CitationRucklehaus, Levin, Johnson, & Kareiva, 2002; CitationWheaton et al., 2017). Within the Interior Columbia Basin in particular (see Plate 1), biological opinions issued by the National Marine Fisheries Service (NMFS), under the National Oceanic and Atmospheric Administration (NOAA), developed population recovery plans that lean heavily on tributary habitat restoration (CitationNMFS, 2008). Accordingly, a myriad of river restoration efforts have been implemented across subwatersheds (e.g. CitationHolburn, Piety, Lyon, McAffee, & Callahan, 2008; CitationReclamation, 2010). Many of these interventions are opportunistic, pursued at a reach scale without knowledge of the watershed context of geomorphic condition and recovery potential. As a consequence, they may not produce the desired overall fish population response because they do not strategically target key limiting factors, connections between and across isolated reaches, or address the root causes of degradation at the appropriate scale (CitationBennett et al., 2016). Moreover, many restoration efforts have the best of intentions, but fail to produce physically realistic goals for the streams they are intended to improve. Restoration efforts can benefit greatly from geomorphic assessments that recognize the importance of the watershed-scale context when evaluating individual stream reach conditions (CitationBeechie et al., 2010; CitationBeechie, Pess, Roni, & Giannico, 2008; CitationDemarchi, Bizzi, & Piégay, 2016). The resulting network-scale maps (i.e. reach resolution and watershed extent over the drainage network) represent the most concise way to distill and communicate the end products of such a geomorphic assessment in a way that can directly support watershed management (CitationWheaton et al., 2017).
Through geomorphic assessment, the rivers and streams that comprise a drainage network of a watershed can be broken into distinctive reaches and similar reach types grouped together (CitationBuffington & Montgomery, 2013; CitationKasprak et al., 2016). Landscape units, lithology and rock strength, stream power and drainage basin area are all important controls on river character and behavior (CitationChurch, 1992; CitationSchumm, 1977). Interactions among these factors shape channels and their floodplains forming reaches of relatively distinctive structure and function (CitationBuffington & Montgomery, 2013; CitationKellerhals, Church, & Bray, 1976). A reach break is the physical transition between different adjacent reach types with characteristic valley setting, planform, bed material, and geomorphic unit assemblages. In this study, such process-based reach types are synonymous with distinct river styles (cf. CitationBrierley & Fryirs, 2005). Valley confinement is a key driver of reach breaks throughout a watershed (e.g. CitationFryirs, Wheaton, & Brierley, 2016a; CitationMontgomery & Buffington, 1997) (see Plate 3). The degree of confinement controls the ability of a channel to adjust laterally and, to some extent, vertically on the valley bottom. Measures of confinement are used to differentiate valley settings (CitationBrierley & Fryirs, 2005; CitationFryirs et al., 2016a).
The geomorphology of river channels and their floodplains is key to understanding the processes that create and maintain habitat conditions suitable for salmonid species (CitationBeechie & Sibley, 1997; CitationGilbert, Macfarlane, & Wheaton, 2016; CitationWheaton et al., 2010). An analysis of a river’s current geomorphic condition and its recovery potential not only informs potential restoration targets and priorities, it can also support assessments of salmonid–habitat relationships at a variety of spatial scales (e.g. CitationISEMP/CHaMP, 2015). Hierarchical geomorphic assessments provide insight that can enhance the success and cost-effectiveness of ongoing salmonid habitat restoration efforts (CitationBennett et al., 2016). Salmonids implicitly ‘consider’ geomorphic features at multiple levels of a geomorphic hierarchy when selecting/using habitats (CitationFausch, Torgersen, Baxter, & Li, 2002). Ideal spawning locations for bull trout (Salvelinus confluentus), for example, are characterized by both a particular in-channel geomorphic unit assemblage (i.e. pool–riffle transitions) and specific valley setting (i.e. unconfined alluvial valleys (CitationBaxter & Hauer, 2000; CitationBean, Wilcox, Woessner, & Muhlfeld, 2014)). In a case such as this, restoration priorities set by in-channel features alone are likely to be misleading. Further, a watershed-scale perspective on the abundance and spatial arrangement of particular reach types – some of which may be rare but critical to particular species and/or life stages – is needed to understand the overall feasibility of watersheds to support robust and resilient fish populations (CitationFausch et al., 2002; CitationRosenfeld & Hatfield, 2006). Yet, fish population and habitat assessments have historically neglected this critical multi-scale view (CitationFausch et al., 2002). This hierarchical perspective of riverine habitat also helps restoration practitioners avoid some of the costly mistakes of the past. For instance, the U.S. Pacific Northwest is replete with examples of large wood placement projects that aimed to enhance salmonid habitat but failed due to a lack of consideration of local geomorphic conditions and watershed hydrology (e.g. CitationFrissell & Nawa, 1992). By formally considering a reach’s natural behavior, trajectory, and capacity for adjustment, such assessments can help restoration practitioners to ‘work with nature’ (CitationBrierley, Fryirs, Outhet, & Massey, 2002) leading to longer lasting and more appropriately sited restoration treatments.
This purpose of this paper and the associated maps is to illustrate a practical application of a multiscalar geomorphic assessment framework that can aid in planning and prioritization of ecological restoration and management. The Main Map embodying the assessment is packaged as an atlas. The atlas supports building realistic expectations for watershed managers and stakeholders to constrain management actions based on a sound understanding of watershed-scale processes. Specifically, links between the physical environment and aquatic ecosystems, support efforts to move beyond site or reach-specific management applications to procedures that work with watershed-specific process relationships. This is especially important for fish protection (CitationFausch et al., 2002). We use the Middle Fork John Day Watershed in Oregon, USA as a case study.
2. Study watershed
The Middle Fork John Day (hereafter MFJD) Watershed, northeast Oregon, USA, is home to populations of summer steelhead listed under the Endangered Species Act and at-risk Chinook salmon (Oncorhynchus tshawytscha) and has been the focus of numerous studies, which make it an excellent candidate for illustrating the potential utility of the River Styles Framework. The MFJD River has been the focus of multiple previous geomorphic investigations (e.g. CitationButcher, Crown, Brannan, Kishida, & Hubler, 2010; CitationDietrich, 2014, Citation2016; CitationMcDowell, 2001; CitationReclamation, 2008; CitationTorgersen, Price, Li, & McIntosh, 1999). CitationKasprak et al. (2016) used the MFJD to compare and contrast different reach typing (stream channel classification) frameworks (including river styles). The MFJD has been the subject of stream temperature thermal fish habitat studies (e.g. CitationFeldhaus, Heppell, Hiram, & Mesa, 2010; CitationMcNyset, Volk, & Jordan, 2015; CitationTorgersen et al., 1999), continuous fish surveys and habitat assessments (e.g. CitationBlanchard, 2015), site-scale bioenergetic ecohydraulic modeling (CitationWall, Bouwes, Wheaton, Saunders, & Bennett, 2015), and salmonid life cycle modeling (CitationMcHugh et al., in press). In addition to fish studies, the MFJD has been the focus of research on freshwater mussels (e.g. CitationBox et al., 2006; CitationHegeman, Miller, & Mock, 2014; CitationMock et al., 2010) that have shed new light on what sort of habitats these species prefer. The MFJD Watershed is also an Intensively Monitored Watershed (CitationBennett et al., 2016) in which extensive restoration is being coordinated (CitationHolburn, Turner, Piety, & Klinger, 2009; CitationReclamation, 2008) in an effort to understand how specific actions influence fish and their habitat (i.e. determine if restoration is effective at increasing the populations). In addition, habitat status and trend monitoring is conducted through the Columbia Habitat and Monitoring Program [CHaMP] (Citation2012). While not the focus of this paper, collectively these past studies in the MFJD provide an excellent backdrop in which the maps presented here can help shed new light and context for.
We conducted a hierarchical geomorphic assessment using CitationBrierley and Fryirs (2005) in the MFJD Watershed to inform ongoing and future research and restoration planning efforts. This framework organizes traditional geomorphic assessment in terms of four stages: (1) river classification (i.e. reach typing); (2) geomorphic condition assessment; (3) recovery potential analysis; and (4) development of a strategic management plan to address potential restoration and rehabilitation goals. Analyses are ‘nested’ across spatial scales of watersheds, landscape units, river reaches, and geomorphic units (landforms) (). Initially, morphometric, hypsometric, and geomorphic analyses are required to characterize river character, behavior and patterns at the watershed scale (summarized as reach types). An understanding of current and historic geomorphic processes along with human perturbation influences are used to assess condition and forecast recovery potential as part of developing a strategic river management plan (CitationWhite, Justice, Kelsey, McCullough, & Smith†, 2017).
Figure 1. Products produced when undertaking a River Styles geomorphic assessment noting the examples that are included in this paper. Superscripts note the hierarchical scale at which the analysis is undertaken.
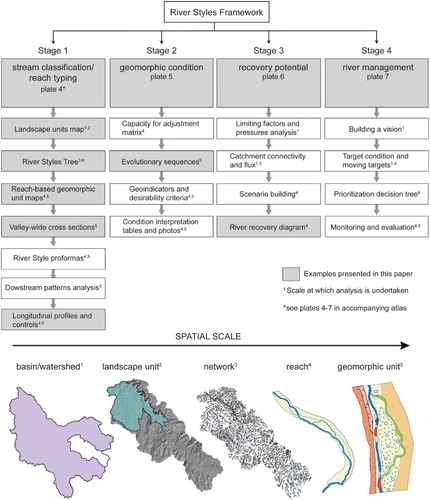
The MFJD Watershed is a 2050 km2 subwatershed of the Columbia River Basin located in east-central Oregon (see Plate 1). The MFJD River flows northwesterly from headwaters on the western flank of the Blue Mountains, a rugged series of ranges in northeastern Oregon. The John Day Basin lies in the rain shadow of the Cascade Range (mean annual precipitation = ∼35–56 cm; temperature range = −10°C to 5°C in winter and 10–30°C in summer) and is underlain by Cretaceous volcanic, marine sedimentary, and granitic rocks overlain by the Miocene Picture Gorge Basalt of the Columbia River Basalt Group (e.g. CitationWalker & MacLeod, 1991). The basin has a semi-arid climate across upland landscapes, but is locally diverse, ranging from alpine and forested mountains to grass- and scrublands of the adjacent foothills and low-relief, temperate steppe uplands. Vegetation communities are stratified along moisture and elevation gradients between mesic highland, mixed spruce and subalpine fir forests, and sage grasslands of the upland and tableland environments.
The MFJD Watershed consists of five Hydrologic Unit Code 10 (HUC) subwatersheds (CitationSeaber, Kapinos, & Knapp, 1987) that join the 131-km long central trunk stream of the MFJD River. The topography contains a high-relief stream network with high drainage density, marked by steep-sloped canyons, deeply dissected highlands, broad tablelands, and rounded uplands replete with broad meadows. We identified and mapped six ‘landscape units’ that range from high elevation, moist alpine terrain in the south and east, to semi-arid volcanic tablelands to the northwest (Plate 2).
3. Mapping data and methods
The methods used to implement the geomorphic assessment are well documented in CitationBrierley and Fryirs (2005; i.e. the River Styles Framework) and summarized in . Here we focus more on describing the specifics of how we implemented that framework within the Middle Fork John Day to produce the maps presented here.
3.1. Desktop analyses and stream survey
The bulk of the desktop analysis and field-based validation work is centered on the regional landscape and watershed investigations essential to the stream classification exercise. To aid in our desktop analysis, we used Google Earth Pro (v.7.1.2.2041, 2013) and other geographic information system (GIS) readable imagery, in conjunction with the National Elevation Dataset (NED; CitationUSGS, 1999) and National Hydrography Dataset (NHD; CitationUSGS, 2007), to document the landscape-scale physiographic attributes such as underlying geology, vegetation patterns and composition, relief, drainage density and a thorough visual interpretation of stream and valley attributes. Air photo analysis is critical for validating preliminary mapping of the valley bottom (CitationGilbert et al., 2016), channel, and where aerial photo resolution allows, for bed material inference and in-channel geomorphic units. Determining reach breaks (e.g. CitationBuffington & Montgomery, 2013; CitationWohl & Merritt, 2008) is the single most important analytical step in developing network-based maps comprising multiple variables (e.g. stream classification, geomorphic condition, recovery potential, and prioritized management classes) (). Reach breaks are identified through changes in valley setting and associated channel confinement (CitationFryirs, Wheaton, & Brierley, 2016b), river planform, the assemblage of geomorphic units (i.e. floodplain and channel landforms; cf. CitationNotebaert & Piégay, 2013; CitationWheaton et al., 2015) and bed material texture. We validated our remotely sensed interpretations with field visits to representative reach type localities to map valley slope, floodplain and in-channel geomorphic units for each unique reach type (i.e. ‘River Style’; e.g. see Plate 3). The field-based ground-truthing, mapping, and data collection efforts are critical for extrapolating channel classes throughout the study watershed.
Table 1. Categorical levels used for the network stream line attributes in each classification and status map (see Plates 4–7 of the atlas).
Longitudinal profile plots provide a key tool for understanding and interpreting the downstream patterns of rivers in each watershed, and controls that govern their form and function. This data display allows for efficient analysis of downstream variations in types of landscape units (and sediment process zones), upstream watershed area, slope, total stream power and their relationships to valley confinement and reach type (). Longitudinal profiles were constructed using the National Hydrography Dataset version 1 (1:24,000) and WBD layers to derive upstream watershed area from an integrated flow accumulation raster derived from a 10 m digital elevation model (DEM). To extract longitudinal profiles, we segmented the streamlines into 100 m reach segments for which we calculated upstream watershed area and reach slope. For this operation we used the Geospatial Modeling Environment (GME) tool (CitationBeyer, 2012).
Figure 2. Controls on channel morphology and downstream patterns of reach types on the Middle Fork John Day River and Squaw Creek.
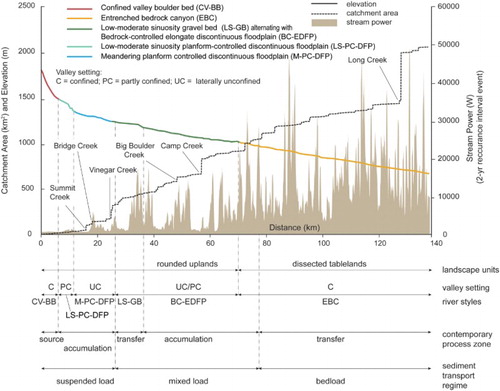
Total stream power, a measure of the capability of a river to do work (i.e. rework and transport sediment) against the bed and banks of the river channel per unit downstream length (e.g. CitationWorthy, 2005), was calculated for each 100 m interval:where ρ is the density of water, g is acceleration due to gravity, Q is a characteristic discharge, S is the channel slope, and Ω is stream power in Watts. We used a two-year recurrence interval flow for discharge (Q2), given the effectiveness of frequent bankfull flows in modifying and maintaining channel form relative to larger magnitude, infrequent flood stage flows (CitationWolman & Miller, 1960). To estimate Q for the Middle Fork John Day River, a regional regression equation was obtained from the United States Geological Survey (USGS) National Streamflow Statistics Website (URL: http://water.usgs.gov/osw/programs/nss/pubs.html) and we used the National Streamflow Statistics Program (CitationRies, 2006) to compute an area-discharge relationship between Q2 and drainage area. The relationship was verified by calculating a linear regression based on seven gauges in the John Day basin, including the Middle Fork, and regional gauge data from northeastern Oregon (CitationHarris & Hubbard, 1982; CitationKasprak & Wheaton, 2012). Streamflow data of flood recurrence and flow duration analyses were obtained from the USGS streamflow website for Oregon (URL: http://or.water.usgs.gov/). The Log-Pearson III analysis of peak discharge data was performed using the methods outlined by CitationKlingeman, Bogavelli, Coles, and Wright (2002) (see Plate 3).
3.2. Building the network-based classification and status maps
The network-based status maps display results of landscape units, river type, geomorphic condition, recovery potential, and prioritized strategic plan analyses. The atlas maps (see Plates 1–7) were built in Esri ArcMap using the 1:24,000 NHD version 1 (CitationUSGS, 2007) as the baseline network for delineating reach breaks and other variables on maps. This cartographically derived, digital vector dataset closely matches the actual course of the river visible in air photos. Line segments of interest were assigned the appropriate categorical variables. For example, segments denoting river classifications begin and end at geomorphic reach breaks. In addition, segments are categorized according to their geomorphic condition, recovery potential and prioritized management (). Stream length and valley confinement proportions were summarized for the whole MFJD Watershed and its five subwatersheds (). We used NED 30 m raster DEMs to extract elevation data and hillshade images, clipped to hydrologic unit codes (HUC) 8 and 10 watershed boundaries. Stream length statistics for each analysis were generated in ArcMap and exported to Microsoft Excel for processing. The completed raster and vector data were exported to Adobe Illustrator for rendering of maps and summary figures.
4. Map guides and discussion
4.1. Stream classification (river character and behavior)
Fifteen different reach types were identified, spanning the range of confined, partly confined, and laterally unconfined valley settings found within the MFJD Watershed (see Plates 1 and 4). This included both perennial and ephemeral streams. Stream attributes leading to the classification are listed in organizational trees that include explicit, objective, and/or quantitative criteria (). We summarized the frequency of stream length by river classes and valley settings for five HUC 10 subwatersheds (). These data are critical for understanding the partitioned nature of the watershed and to track attributes that are helpful for a variety of geomorphic and habitat-related analyses. For example, summarizes stream length data for the 962 km perennial network, which is used by anadromous fish, whereas Plate 1 shows the equivalent mapping for the entire 4110 km perennial, ephemeral, and intermittent drainage network. This provides insight into geomorphic parameters that may be directly relevant to fish and their habitat or indirectly through their more sporadic contributions of water, wood, and sediment to the perennial network from upstream tributaries. Since Plate 4 summarizes the same numbers for the entire perennial, intermittent, and ephemeral drainage network, it may be more appropriate to informing a holistic watershed management approach as opposed to just fish-centric management activities. For those interested in how the River Styles classification reported here compares to that of other common classification systems, the reader is referred to CitationBuffington and Montgomery (2013) and CitationKasprak et al. (2016). This latter paper includes a comparison specific to the MFJD Watershed.
Figure 3. Example River Styles tree for Middle Fork John Day Watershed streams in the partly confined valley setting. This tree documents the key attributes of these reach types and is ordered in a hierarchical fashion. River Styles trees were also completed for streams of confined, and laterally unconfined valley settings but are not shown here (see CitationO’Brien & Wheaton, 2015).
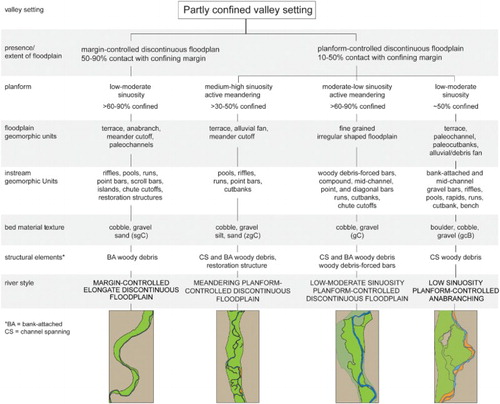
Figure 4. Summary distribution of reach types (River Styles) of perennial stream in terms of stream length and valley confinement. For information that includes the ephemeral stream network, see Plate 4 of the atlas (supplemental material).
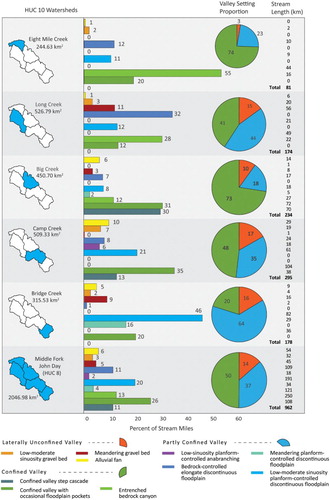
For each representative downstream pattern of River Styles, CitationO’Brien and Wheaton (2015) produced a longitudinal profile depicting geomorphic controls including landscape units (and geology), total stream power, and sediment process zones (i.e. ). As noted by CitationMay, Roering, Eaton, and Burnett (2013) and CitationMay, Roering, Snow, Griswold, and Gresswell (2017), geomorphic controls upon knickpoint development and valley confinement relationships exert a primary control upon fish stocks, and associated fish management issues, in this part of the world.
4.2. Geomorphic condition
Streams and rivers are dynamic entities. The propensity for channel adjustment varies across River Styles. The current geomorphic condition of each reach reflects its capacity for adjustment, and an analysis of river evolution () that considers whether the reach has a contemporary structure and function that is expected for that River Style (CitationFryirs, 2015). A range of geomorphic indicators are used to perform this analysis (Plate 5). Thus, reaches of the same style can be in various states of geomorphic condition. Analyses of geomorphic condition highlight the discrepancy between historic and current channel configuration and identifies potential locations for mitigation or protection.
We assigned geomorphic condition for each reach based on the physical indicators that informed the condition assessment. These explanations, in conjunction with watershed maps, offer managers a resource for more effectively identifying problem areas and opportunities when designing a management plan (see CitationO’Brien & Wheaton, 2015). The MFJD Watershed contains a range of rivers in various geomorphic conditions. Plate 5 partitions the stream network into categories of intact, good, moderate, and poor geomorphic condition. We also derived stream length metrics for the perennial network to include the portions of subwatersheds hosting populations of salmonid species ((A) and Plate 1).
Figure 6. Combined perennial stream length data for subwatersheds of the Middle Fork John Day Watershed. (A) Geomorphic condition data are on left hand panel and (B) recovery potential data are on the right hand panel. Note these data summarize the perennial network, whereas stream length data summarized for the whole watershed are presented in Plates 4–7 of the atlas.
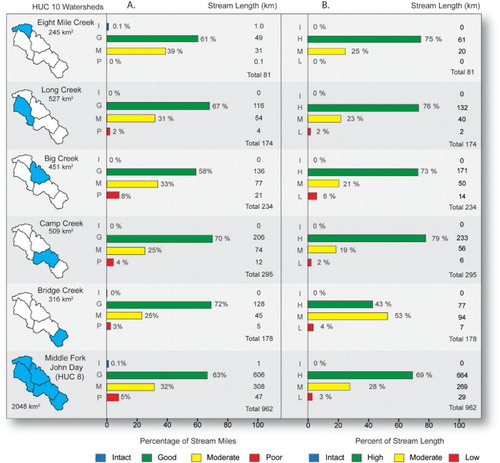
4.3. Geomorphic recovery potential
An analysis of a reach’s capacity for improvement in geomorphic condition over a relevant time period, generally 50–100 years, serves as the primary basis for assessing river recovery potential (CitationFryirs & Brierley, 2016). Key to these assessments are (1) an understanding of the sensitivity to adjustment and responses to historical impacts; (2) the landscape/watershed position of the affected reach and its proximity to either good or poor condition reaches (particularly those positioned upstream); and (3) consideration of the current (and likely future) limiting factors and pressures that impact upon that reach. The recovery potential of a specific reach is represented on a river recovery diagram that presents the current state and the predicted, potential future outcome, given different management scenarios from the ‘do-nothing’ (passive restoration) to the ‘full intervention’ options (). The sum of these assessments is shown on Plate 6 and summarized as perennial stream length data in (B).
Figure 7. Geomorphic condition variants shown as conceptual cross sections, and their recovery potential, for the low sinuosity gravel bed River Style. The current conditions are shown at left, and restored, rehabilitated and created conditions and potential pathways are shown to the right.
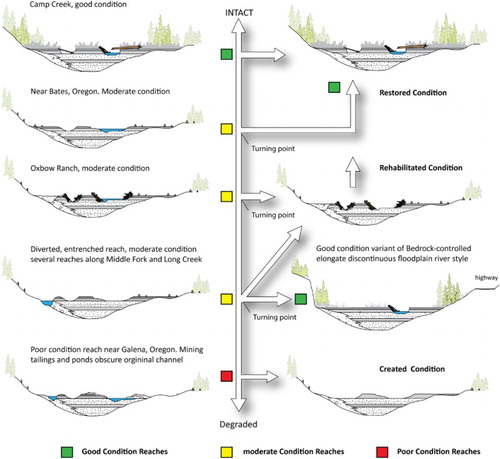
Our watershed map of geomorphic recovery potential (Plate 6) suggests that, with a few exceptions, most streams in the MFJD Watershed have a high capacity to recover from land use pressures without intervention. However, streams in the southeast portion of the watershed have incurred disproportionate impacts in a relatively delicate landscape (basic soils, sparse forests, accessible terrain for multiple land uses), and have only moderate recovery potential. Isolated reaches of the mainstem and a few tributaries have poor recovery potential – their geomorphic condition and function will not improve without intervention (e.g. (B), Bridge Creek Unit).
4.4. Building a prioritized river management plan
Using the results of reach types, geomorphic condition, and recovery potential, we developed a watershed-framed strategic plan wherein realistic goals for river rehabilitation and restoration occurring over a timeframe of 50–100 years are defined (see Plate 7). The proposed plan is not a major departure from the key management drivers (e.g. CitationReclamation, 2010) that are currently operating in the MFJD Watershed. Management objectives in our hypothetical, geomorphically focused strategic management plan encourage conservation of unique or remaining natural areas, followed by restoration and rehabilitation efforts that support and promote the geomorphic function (i.e. discharge and sediment flux) of good condition reaches with high recovery potential. Reaches in poor condition with little recovery potential are given the lowest priority for rehabilitation or restoration.
5. Conclusions and implications
Our study presents a series of maps for the MFJD Watershed in northeast Oregon, which help set physically realistic, geomorphic bounds on what might be possible for managers to achieve through restoration and conservation actions. The maps provide consistent, watershed-wide assessments of geomorphic reach type, condition and recovery potential to guide river restoration planning and inform strategic river management practice. The communication of findings using maps is intuitive, simplifying outputs from quite complex geomorphic assessments such as CitationO’Brien and Wheaton (2015) and CitationReclamation (2008). The results corroborate previous documentation that the MFJD Watershed has experienced significant impact through grazing operations, road building and clear-cut logging, channel re-routing, floodplain/wetland drainage, and channel bed mining throughout the last century (CitationNOAA, 2013; CitationReclamation, 2010). Fortunately, the most damaging of these practices have since been curtailed and the recovery potential for the watershed is very favorable with 69% of perennial streams and 74% of all streams showing high recovery potential. While the maps can provide geomorphic insight that is immediately relevant to assessments of physical habitat for fish, they do not consider other ecological (e.g. temperature and food availability) or socio-political (e.g. land ownership) factors that might influence the inherent value or recovery potential of reaches. The preliminary strategic management map we present here is reasonable from a physical feasibility perspective, but further modifications to reflect the values of the various stakeholders involved in the planning process would be necessary (CitationO’Brien, Wheaton, & Bouwes, 2015). In systems with a more complicated array of impacts extending beyond just physical habitat (CitationWheaton et al., 2017), the River Styles Framework can easily be combined with other lines of evidence, beyond the physical environment, to inform management decisions.
Software
Network-based analyses and their derivative maps were processed Using Esri ArcMap™ 10.3.1.4959. Google Earth Pro v.7.1.2.2041 was used to search and validate our geomorphic interpretations during the ‘desktop’ phase of the study. Longitudinal profile plots were extracted using the GME tool (CitationBeyer, 2012). Stream length data were summarized and plotted in Microsoft Excel, and all maps and figures were rendered using Adobe Illustrator version CC version 17.1.0 (64 bit).
A geomorphic assessment to inform strategic stream restoration planning in the Middle Fork John Day Watershed, Oregon, USA.pdf
Download PDF (34.4 MB)Acknowledgements
Kirstie Fryirs thanks the Australian Research Council for financial support. Gary Brierley thanks University of Auckland for support on study leave. The authors are grateful to three reviewers for their helpful critiques and insights, which helped improve the clarity of the maps and manuscript.
Disclosure statement
No potential conflict of interest was reported by the authors.
Additional information
Funding
References
- Baxter, C. V., & Hauer, F. R. (2000). Geomorphololgy, hyporheic exchange, and selection of spawning habitat by bull trout (Salvelinus confluentus). Canadian Journal of Fisheries and Aquatic Sciences, 57, 1470–1481. doi:10.1139/f00-056
- Bean, J. R., Wilcox, A. C., Woessner, W. W., & Muhlfeld, C. C. (2014). Multiscale hydrogeomorphic influences on bull trout (Salvelinus confluentus) spawning habitat. Canadian Journal of Fisheries and Aquatic Sciences, 72(4), 17. doi:10.1139/cjfas-2013-0534
- Beechie, T., & Imaki, H. (2014). Predicting natural channel patterns based on landscape and geomorphic controls in the Columbia River basin, USA. Water Resources Research, 50, 39–57. doi:10.1002/2013WR013629
- Beechie, T., Pess, G., Roni, P., & Giannico, G. (2008). Setting river restoration priorities: A review of approaches and a general protocol for identifying and prioritizing actions. North American Journal of Fisheries Management, 28, 891–905. doi:10.1577/M06-174.1
- Beechie, T., Sear, D. A., Olden, J. D., Pess, G. R., Buffington, J. M., Moir, H., … Pollock, M. M. (2010). Process-based principles for river restoration. Bioscience, 60(3), 209–222. doi:10.1525/bio.2010.60.3.7
- Beechie, T. J., & Sibley, T. H. (1997). Relationships between channel characteristics, woody debris, and fish habitat in northwestern Washington streams. Transactions of the American Fisheries Society, 126, 217–229. doi:10.1577/1548-8659(1997)126<0217:RBCCWD>2.3.CO;2
- Bennett, S., Pess, G., Bouwes, N., Roni, P., Bilbly, R. E., Gallagher, S. P., … Greene, C. (2016). Progress and challenges of testing the effectiveness of stream restoration in the Pacific Northwest using Intensively Monitored Watersheds. Fisheries, 41(2), 92–103. doi:10.1080/03632415.2015.1127805
- Beyer, H. L. (2012). Geospatial modelling environment (Version 0.7.2.1) (software) [software]. Retrieved from http://www.spatialecology.com/gme
- Blanchard, M. R. (2015). Using network models to predict steelhead abundance Middle Fork John Day, Oregon (Masters of science masters). Utah State University, Logan, UT.
- Box, J. B., Howard, J., Wolf, D., O Brien, C., Nez, D., & Close, D. (2006). Freshwater mussels (Bivalvia: Unionoida) of the Umatilla and Middle Fork John Day rivers in eastern Oregon. Northwest Science, 80(2), 95–107.
- Brierley, G., & Fryirs, K. (2005). Geomorphology and river management: Applications of the River Styles Framework. Victoria: Blackwell Publishing.
- Brierley, G., Fryirs, K., Outhet, D., & Massey, C. (2002). Application of the River Styles Framework as a basis for river management in New South Wales, Australia. Applied Geography, 22(1), 31. doi:10.1016/S0143-6228(01)00016-9
- Buffington, J. M., & Montgomery, D. R. (1999). A procedure for classifying textural facies in gravel-bed rivers. Water Resources Research, 35(6), 1903–1914. doi:10.1029/1999WR900041
- Buffington, J. M., & Montgomery, D. R. (2013). Geomorphic classification of rivers. Treatise on Geomorphology, 9, 730–767. Elsevier. doi: 10.1016/B978-0-12-374739-6.00263-3
- Butcher, D., Crown, J., Brannan, K., Kishida, K., & Hubler, S. (2010). John Day River basin Total Maximum Daily Load (TMDL) and Water Quality Management Plan (WQMP). Portland, OR: Oregon Department of Environmental Quality. DEQ 10-WQ-025.
- Church, M. (1992). Channel morphology and typology. In P. Callow & G. E. Petts (Eds.), The rivers handbook (pp. 126–143). Oxford: Blackwell.
- Columbia Habitat Monitoring Program (CHaMP). (2012). Scientific protocol for salmonid habitat surveys within the Columbia Habitat Monitoring Program. Wauconda, WA. Retrieved from http://champmonitoring.org/Program/RetreiveProgramDocumentFile/1/Protocol%20Documents/1113376526
- Demarchi, L., Bizzi, S., & Piégay, H. (2016). Hierarchical object-based mapping of riverscape units and in-stream mesohabitats using LiDAR and VHR imagery. Remote Sensing, 8(2), 97. doi:10.3390/rs8020097
- Dietrich, J. T. (2014). Applications of structure-from-motion photogrammetry to fluvial geomorphology (PhD). University of Oregon, Eugene, OR.
- Dietrich, J. T. (2016). Riverscape mapping with helicopter-based structure-from-motion photogrammetry. Geomorphology, 252, 144–157. doi:10.1016/j.geomorph.2015.05.008
- Fausch, K. D., Torgersen, C. E., Baxter, C. V., & Li, H. W. (2002). Landscapes to riverscapes: Bridging the gap between research and conservation of stream fishes. Bioscience, 52(6), 483–498. doi:10.1641/0006-3568(2002)052[0483:ltrbtg]2.0.co;2
- Feldhaus, J. W., Heppell, S. A., Hiram, L., & Mesa, M. G. (2010). A physiological approach to quantifying thermal habitat quality for redband rainbow trout (Oncorhynchus mykiss gairdneri) in the South Fork John Day River, Oregon. Environmental Biology of Fishes, 87, 277–290. doi: 10.1007/s10641-010-9580-6
- Frissell, C. A., & Nawa, R. K. (1992). Incidence and causes of physical failure of artificial habitat structures in streams of Western Oregon and Washington. North American Journal of Fisheries Management, 12(1), 15. doi:10.1577/1548-8675(1992)012<0182:IACOPF>2.3.CO;2
- Fryirs, K. (2015). Developing and using geomorphic condition assessments for river rehabilitation planning, implementation and monitoring. WIREs Water, 2(6), 649–667. doi:10.1002/wat2.1100
- Fryirs, K., Wheaton, J., & Brierley, G. J. (2016a). An approach for measuring confinement and assessing the influence of valley setting on river forms and processes. Earth Surface Processes and Landforms. doi:10.1002/esp.3893
- Fryirs, K. A., & Brierley, G. J. (2016). Assessing the geomorphic recovery potential of rivers: forecasting future trajectories of adjustment for use in management. Wiley Interdisciplinary Reviews: Water, 3(5), 727–748. doi:10.1002/wat2.1158
- Fryirs, K. A., Wheaton, J. M., & Brierley, G. (2016b). An approach for measuring confinement and assessing the influence of valley setting on river forms and processes. Earth Surface Processes and Landforms. doi:10.1002/esp.3893
- Gilbert, J. T., Macfarlane, W. W., & Wheaton, J. M. (2016). The Valley Bottom Extraction Tool (V-BET): A GIS tool for delineating valley bottoms across entire drainage networks. Computers & Geosciences, 97, 1–14. doi:10.1016/j.cageo.2016.07.014
- Harris, D. D., & Hubbard, L. E. (1982). Magnitude and frequency of floods in eastern Oregon. Retrieved from USGS Water-Resources Investigations Report.
- Hegeman, E. E., Miller, S. W., & Mock, K. E. (2014). Modeling freshwater mussel distribution in relation to biotic and abiotic habitat variables at multiple spatial scales. Canadian Journal of Fisheries and Aquatic Sciences, 71(10), 1483–1497. doi: 10.1139/cjfas-2014-0110
- Holburn, E., Piety, L. A., Lyon, E. W., McAffee, R., & Callahan, D. (2008). Middle Fork and Upper John Day Tributary Assessments, Grant County, Oregon. Boise, Idaho: American Society of Civil Engineers.
- Holburn, E., Turner, T., Piety, L., & Klinger, R. (2009). Habitat restoration on the Middle Fork John Day River. World environmental and water resources congress 2009: Great Rivers (pp. 1–12). Kansas City, MO: American Society of Civil Engineers.
- ISEMP/CHaMP. (2015). Combined integrated and status monitoring program and Columbia habitat monitoring program calendar year 2014 annual report. Prepared by ISEMP and CHaMP for the Bonneville Power Administration. B. P. Administration.
- Kasprak, A., Hough-Snee, N., Beechie, T., Bouwes, N., Brierley, G., Camp, R., … Wheaton, J. (2016). The blurred line between form and process: A comparison of stream channel classification frameworks. PLoS One, 11(3), e0150293. doi:10.1371/journal.pone.0150293
- Kasprak, A., & Wheaton, J. (2012). Development of a rapid geomorphic assessment procedure for streams in the John Day River Watershed, Oregon. Logan, UT: Ecogeomorphology & Topographic Analysis Lab for Eco Logical Research, Inc.
- Kellerhals, R., Church, M., & Bray, D. I. (1976). Classification and analysis of river processes. Journal of the Hydraulics Division-ASCE, 102(7), 813–829.
- Klingeman, P., Bogavelli, V., Coles, D., & Wright, M. (2002). Streamflow evaluations for river restoration planning and design. Retrieved from http://streamflow.engr.oregonstate.edu/index.htm
- Mann, C. C., & Plummer, M. L. (2000). Can science rescue salmon? Science, 289(5480), 716–719. doi:10.1126/science.289.5480.716
- May, C., Roering, J., Eaton, L. S., & Burnett, K. M. (2013). Controls on valley width in mountainous landscapes: The role of landsliding and implications for salmonid habitat. Geology, 41(4), 503–506. doi:10.1130/g33979.1
- May, C., Roering, J., Snow, K., Griswold, K., & Gresswell, R. (2017). The waterfall paradox: How knickpoints disconnect hillslope and channel processes, isolating salmonid populations in ideal habitats. Geomorphology, 277, 228–236. doi:10.1016/j.geomorph.2016.03.029
- McDowell, P. F. (2001). Spatial variations in channel morphology at segment and reach scales, Middle Fork John Day River, northeastern Oregon. In J. M. Dorava, D. R. Montgomery, B. B. Palcasak, & F. A. Fitzpatrick (Eds.), Geomorphic processes and riverine habitat ( Vol. water science and application volume 4; pp. 159–172). Washington, D.C.: American Geophysical Union.
- McHugh, P., Saunders, C., Bouwes, N., Wall, E., Bangen, S., Wheaton, J., … Jordan, C. (in press). Linking models across scales to assess the viability and restoration potential of a threatened population of steelhead (Oncorhynchus mykiss) in the Middle Fork John Day River, Oregon, USA. Ecological Modelling.
- McNyset, K., Volk, C., & Jordan, C. (2015). Developing an effective model for predicting spatially and temporally continuous stream temperatures from remotely sensed land surface temperatures. Water, 7(12), 6827–6846. doi:10.3390/w7126660
- Mock, K. E., Box, J. C. B., Chong, J. P., Howard, J. K., Nez, D. A., Wolf, D., & Gardner, R. S. (2010). Genetic structuring in the freshwater mussel Anodonta corresponds with major hydrologic basins in the western United States. Molecular Ecology, 19(3), 569–591. doi:10.1111/j.1365-294X.2009.04468.x
- Montgomery, D. R. (2004). Geology, geomorphology, and the restoration ecology of salmon. GSA Today, 14(11), 4–12. doi:10.1130/1052-5173(2004)014<4:GGATRE>2.0.CO;2
- Montgomery, D. R., & Buffington, J. M. (1997). Channel-reach morphology in mountain drainage basins. Geological Society of America Bulletin, 109(5), 596–611. doi:10.1130/0016-7606(1997)109<0596:CRMIMD>2.3.CO;2
- NMFS. (2008). Endangered Species Act – Section 7 Consultation Biological Opinion and Magnuson-Stevens Fishery Conservation and Management Act Essential Fish Habitat Consultation: Consultation on Remand for Operation of the Federal Columbia River Power System, 11 Bureau of Reclamation Projects in the Columbia Basin and ESA Section 10(a)(1)(A) Permit for Juvenile Fish Transportation Program. In N. M. F. Service (Ed.). Portland, Oregon: National Marine Fisheries Service.
- NOAA. (2013). Pacific salmonids: Major threats and impacts. Retrieved from http://www.nmfs.noaa.gov/pr/species/fish/salmon.htm
- Notebaert, B., & Piégay, H. (2013). Multi-scale factors controlling the pattern of floodplain width at a network scale: The case of the Rhône basin, France. Geomorphology, 200, 155–171. doi:10.1016/j.geomorph.2013.03.014
- O’Brien, G. R., & Wheaton, J. M. (2015). River Styles report for the Middle Fork John Day Watershed, Oregon – example report for exploring leveraging the River Styles Framework in tributary habitat management for the Columbia River Basin. Logan, UT: Ecogeomorphology and Topographic Analysis Lab.
- O’Brien, G. R., Wheaton, J. M., & Bouwes, N. (2015). Synthesis & recommendations from Middle Fork John Day River Styles – leveraging the River Styles Framework in tributary habitat management for the Columbia River Basin. Logan, UT: Fluvial Habitats Center, Utah State University.
- Reclamation, U. S. B. o. (2010). Oxbow conservation area reach assessment, Middle Fork John Day River, Grant County, Oregon. Boise, Idaho: Pacific Northwest Regional Office.
- Reclamation, U. S. B. o. (2008). Middle Fork and Upper John Day River tributary assessments Grant County, Oregon. Boise, ID: Pacific Northwest Regional Office.
- Ries, KG,III. (2006). The national streamflow statistics program: A computer program for estimating streamflow statistics for ungaged sites. Reston, Virginia: U. S. G. Survey. Retrieved from U.S. Geological Survey Techniques and Methods Report TM Book 4, Chapter A6.
- Rosenfeld, J., & Hatfield, T. (2006). Information needs for assessing critical habitat of freshwater fish. Canadian Journal of Fisheries and Aquatic Sciences, 63, 683–698. doi:10.1139/f05-242
- Rucklehaus, M. H., Levin, P., Johnson, J. B., & Kareiva, P. M. (2002). The Pacific salmon wars: What science brings to the challenge of recovering species. Annual Review of Ecology and Systematics, 33(Annual Reviews), 665–706. doi:10.1146/annurev.ecolsys.33.010802.150504
- Schumm, S. A. (1977). The fluvial system. New York, NY: Wiley.
- Seaber, P. R., Kapinos, F. P., & Knapp, G. L. (1987). Hydrologic unit maps. (2294). United States Government Printing Office.
- Torgersen, C. E., Price, D. M., Li, H. W., & McIntosh, B. A. (1999). Multiscale thermal refugia and stream habitat associations of Chinook salmon in northeastern Oregon. Ecological Applications, 9(1), 301–319. doi:10.1890/1051-0761(1999)009[0301:MTRASH]2.0.CO;2
- USGS. (1999). National Elevation Dataset. Retrieved from http://ned.usgs.gov/
- USGS. (2007). National Hydrography Dataset. Retrieved from http://nhd.usgs.gov/
- Walker, G. W., & MacLeod, N. S. (Cartographer). (1991). Geologic map of Oregon. Retrieved from http://mrdata.usgs.gov/sgmc/or.html
- Wall, C. E., Bouwes, N., Wheaton, J. M., Saunders, W. C., & Bennett, S. N. (2015). Net rate of energy intake predicts reach-level steelhead (Oncorhynchus mykiss) densities in diverse basins from a large monitoring program. Canadian Journal of Fisheries and Aquatic Sciences, 1–11. doi:10.1139/cjfas-2015-0290
- Wheaton, J., Bouwes, N., McHugh, P., Saunders, W. C., Bangen, S. G., Bailey, P. E., … Jordan, C. (2017). Upscaling site-scale ecohydraulic models to inform salmonid population-level life cycle modelling and restoration actions – lessons from the Columbia River Basin. Earth Surface Processes and Landforms. doi:10.1002/esp.4137
- Wheaton, J. M., Brasington, J., Darby, S. E., Merz, J., Pasternack, G. B., Sear, D., & Vericat, D. (2010). Linking geomorphic changes to salmonid habitat at a scale relevant to fish. River Research and Applications, 26(4), 469–486. doi:10.1002/rra.1305
- Wheaton, J. M., Fryirs, K. A., Brierley, G. J., Bangen, S. G., Bouwes, N., & O’Brien, G. R. (2015). Geomorphic mapping and taxonomy of fluvial landforms. Geomorphology, 248, 273–295. doi:10.1016/j.geomorph.2015.07.010
- White, S. M., Justice, C., Kelsey, D. A., McCullough, D. A., & Smith†, T. (2017). Legacies of stream channel modification revealed using general land office surveys, with implications for water temperature and aquatic life. Elementa Science of the Anthropocene, 5(3). doi:10.1525/journal.elementa.192
- Wohl, E., & Merritt, D. M. (2008). Reach-scale channel geometry of mountain streams. Geomorphology, 93(3–4), 168–185. doi:10.1016/j.geomorph.2007.02.014
- Wolman, M. G., & Miller, J. P. (1960). Magnitude and frequency of forces in geomorphic processes. Journal of Geology, 68, 54–74. doi: 10.1086/626637
- Worthy, M. (2005). High-resolution total stream power estimates for the Cotter River, Namadgi National park, Australian Capital Territory. In I. C. Roach (Ed.), Regolith 2005 – ten years of the centre for resource and environment studies (pp. 338–343). Canberra: Australian National University.