ABSTRACT
The paper presents a detailed mass movement inventory map of the Licetto River basin, an intermountain catchment of 50 km2 formed during the Quaternary in response to extensional tectonics dissecting fold-and-thrust belts of the Calabrian Coastal Range (Southern Italy). The map (Main Map) is the result of both an integration between geological and morphological data derived from the visual analysis of aerial photographs at different times and scales, and the collection of new data obtained from multi-temporal field surveys. The study area is affected by a total of 824 mass movements, frequently made by superimposed bodies of different types, states of activity and sizes, including some kilometre-scale Deep-Seated Gravitational Slope. The majority of the mapped landslides, mainly of slide type, involve low-grade metamorphic rocks which also show the exclusive presence of deep-seated gravitational slope deformations. Analysis of the inventory map revealed that 40% of the mapped landslides, often attributable to very slow-moving landslides, can be considered active. The Main Map represents a useful tool for territorial planning and engineering – geological and environmental purposes in this complex geo-structural area, providing a useful contribution for quantitative landslide risk analyses and the design of appropriate risk-mitigation measures.
1. Introduction
Mass movements constitute an important process in the evolution of landscapes in mountain regions and represent a serious hazard in many areas of the Italian territory (CitationGuzzetti, Stark, & Salvati, 2005).
Mass movement inventory maps, which can be prepared using traditional methods and new techniques (CitationGuzzetti et al., 2012), are very important to both investigate the distribution, types, activity and frequency of occurrence of these phenomena and study the spatio-temporal evolution of landscapes dominated by landslides. In addition, a detailed inventory of mass movements constitutes an essential tool for landslide susceptibility, hazard and risk assessment (CitationFell et al., 2008).
The Calabria region (Southern Italy, ), a result of its peculiar geological framework, tectonic history and climatic characteristics, is very prone to mass movement development, due to the combinations of lithological, tectonic, structural and morphological factors and, very often, to the destabilizing effects of human activities (CitationAntronico et al., 2013; CitationBorrelli, Antronico, Gulla, & Sorriso-Valvo, 2014; CitationCiurleo, Calvello, & Cascini, 2016; CitationCiurleo, Cascini, & Calvello, 2017; CitationConforti, Muto, Rago, & Critelli, 2014; CitationGullà, Peduto, Borrelli, Antronico, & Fornaro, 2017; CitationSorriso-Valvo & Tansi, 1996; CitationTansi et al., 2016).
The aim of this study was both the reconstruction of the geological and structural setting and the identification and mapping of mass movements in the Licetto River basin (Calabrian Coastal Range, ). To pursue these goals, landslides were identified through the visual analysis of stereoscopic aerial photographs (e.g. CitationBrardinoni, Slaymaker, & Hassan, 2003; CitationBrunsden, 1993), field surveys, landslide event information and the related effects (as suggested by CitationAntronico et al., 2013, Citation2015; CitationBorrelli, Gioffrè, Gullà, & Moraci, 2012; CitationSchuster, 1996).
The studied area (of about 50 km2) is widely affected by active and dormant landslides, of different types and sizes, and deep-seated gravitational slope deformations (DSGSD) which make built-up areas and roads/infrastructure prone to continuous deformation and severe landslide risk.
Finally, through the storage and management of the collected data using a Geographic Information System (GIS), the mass movement inventory map (Main Map) was produced.
2. Materials and method
The geological and geomorphological study of the Licetto River catchment includes two successive steps. In the first step, geological and structural investigations were carried out through aerial photo interpretation and field surveys, to both investigate and verify the morphological evidence linked to tectonics, and identify faults and rock types.
In the second step, a mass movement inventory map of the study area was prepared using both the interpretation of multi-temporal stereoscopic aerial photographs (1:25,000 and 1:33,000 scale black-and-white and 1:15,000 scale colour aerial photographs, acquired from 1955 to 2001) and the visual analysis of freely accessible Google Earth images (e.g. CitationBoardman, 2016; CitationBorrelli, Cofone, Coscarelli, & Gullà, 2015) available from 2001 to 2014, followed by detailed geomorphological field surveys carried out from March 2010 to February 2017.
Type and state of activity of landslides were attributed following the classification proposed by CitationCruden and Varnes (1996), and using geomorphological criteria based on field recognition and freshness of the topographic signatures typical of gravity-related landforms (CitationRib & Liang, 1978), including scarps, conjugate scarps, step terraces, irregular slope profiles (alongside convex or concave forms), ground cracks, slope ruptures, changes in the drainage network, and damage to buildings and infrastructure taken at different times.
Finally, the Main Map was produced on a 1:5000 topographic base (Carta Tecnica Regionale – CTR) and implemented in a GIS using a 5 m resolution digital elevation model. All the collected data were mapped (as polygon or polyline features) and coded in the UTM-WGS84 coordinate system.
3. Geology and tectonics
The geological setting of the study area is characterized by the presence of different basement units, each with a different tectono-metamorphic evolution arranged in a nappe-pile stack, forming an allochthonous tectonic building piled up in the Oligocene – Lower Miocene (CitationAmodio-Morelli et al., 1976; CitationCirrincione et al., 2015; CitationFilice et al., 2015; CitationMuto & Perri, 2002; CitationOrtolano, Cirrincione, Pezzino, Tripodi, & Zappala, 2015; CitationTansi, Muto, Critelli, & Iovine, 2007; CitationVan Dijk et al., 2000), which overthrusted the Mesozoic carbonate Unit of Apennine affinity (). The lower plate outcrops along the Coastal Range in several tectonic windows ((A)), among these the most extensive is represented by the Mt. Cocuzzo Unit (CitationIetto & Ietto, 1998; CitationTansi et al., 2007; CitationVan Dijk et al., 2000; CitationVespasiano et al., 2014).
Figure 2. (A) Geo-structural sketch of the study area (modified from CitationTansi et al., 2007). Legend (1) Holocene deposits; (2) terraced Late Pleistocene marine deposits; (3) terraced Late Pleistocene continental deposits; (4) Middle Pliocene – Middle Pleistocene deposits; (5) Middle Miocene – Early Pliocene deposits; (6) Sheared basement remnants (Paleozoic-Mesozoic); (7) Paragneiss Unit (Paleozoic); (8) Ortogneiss Unit (Paleozoic); (9) Slate and Metapelite Unit (Paleozoic-Mesozoic); (10) Ophiolite Unit (Tithonian-Neocomian); (11) Mesozoic carbonate Unit (Late Triassic); (12) normal fault; (13) major strike-slip fault; (14) minor strike-slip fault; (15) transpressive thrust; (16) overthrust. (B) Geotectonic map of the Licetto River basin: (1) landslide debris; (2) colluvial/detrital deposits; (3) debris/alluvial fans; (4) alluvial deposits; (5) terraced conglomerates and sands; (6) clays and marls; (7) calcarenites; (8) sandstones; (9) conglomerates; (10) gneiss; (11) phyllites and relative residual covers; (12) serpentinites and metabasites; (13) dolostones and limestone; (14) normal fault; (15) strike-slip fault; (16) fault with undetermined kinematics; (17) thrust fault; and (18) attitude of bed and foliation.
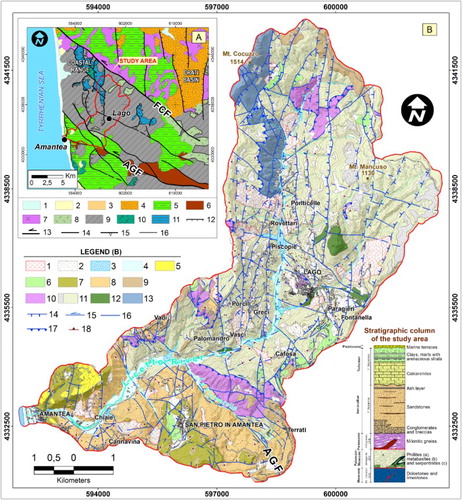
The geology of the Licetto River basin ((B)) is mainly constituted by foliated black and greysh slates ((A)), metalimestones ((B)), metarenites – interbedded with quartzite horizons defining isoclinal folds ((C,D)) – belonging to the Metapelite Unit (sensu CitationVan Dijk et al., 2000). The Metapelite Unit (Palaeozoic-Mesozoic, sensu CitationTansi et al., 2007) is structured in flat lying thrust (duplex) and transposed folds which cause the thickening of the unit. In some cases, especially at the base of the tectono-metamorphic unit, these rocks contain metabasite and serpentinite (CitationPiluso, Cirrincione, & Morten, 2000) involved in the duplex structures and in the core of major folds ((E,F)). Locally, at the top of the Metapelite Unit, limited outcrops of gneiss, mylonitic augen-gneiss ((G)) and schists, belonging to the Orthogneiss Unit (Castagna Unit of CitationAmodio-Morelli et al., 1976), tectonically remain ((B)).
Figure 3. Field photographs of rock types outcropping in the study area. (A) Phyllites with well foliation and quartz mullions in transposed fold hinge crosscutted by vein quartz infilled tension gashes; (B) dark metacalcarenites; (C) quartzite interbedded to phyllite; (D) isoclinal hinge fold in quartzite layers; (E) macroscopic fold core involving serpentinites and phyllites; (F) serpenitites; (G) orthogneiss Unit made by augen milonitic gneiss; (H) megalodon rich dolostones; (I) overthrust of the Metapelite Units on the Mt. Cocuzzo Unit (the thrust is offset by Neogene-Quaternary major faults); (J) matrix and clast-supported Serravallian conglomerates; (K) well stratified Serravallian sandstones (the bed show conglomerates at the base evolving amalgamated layer and siltstone); (L) Tortonian calcarenites (bioclastic/siliciclastic mixed arenites) showing low- and high-angle cross stratification of tidal environment; (M) clays and marls of upper Tortonian age (blackish and manganesiferous silty layers are interbedded); (N) Pleistocene terraced surface and particular of marine (beach face) deposits; and (O) alluvial deposits of the Licetto River near the Amantea town.
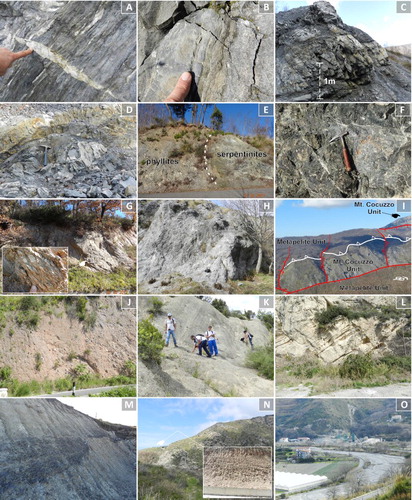
The crystalline basement is overthrusted on the Mesozoic carbonate rocks (Mt. Cocuzzo Unit in CitationIetto & Ietto, 1998), mainly constituted by dolostone ((H)) and limestone. The carbonate Unit and the overthrust are well exposed in the north-western sector of the studied area ((B) and (I)).
On the metamorphic units of the chain a transgressive Middle-Late Miocene sedimentary succession onlaps extensively, mostly in the south-western sector of the area ((B)) and is referred to the perithyrrenian Neogene basins (CitationCritelli, Muto, Perri, & Tripodi, 2017; CitationMattei et al., 2002; CitationMuto & Perri, 2002; CitationMuto, Spina, Tripodi, Critelli, & Roda, 2014).
The sedimentary infill consists of a basal sequence (CitationColella, 1995; CitationMuto & Perri, 2002) made up of breccias, conglomerates and sandstones of a continental and marine environment. The breccia and conglomerate stratigraphic unit outcrop around the Terrati village and in the south-western sector of the map ((B)). They are made up of pebbles and boulders of crystalline and metamorphic composition with a coarse grained matrix and massive structures ((J)). Conglomerates pass laterally and upward to deltaic arenites and sands with intercalations, in the upper part, of an ash layer (CitationMuto & Perri, 2002). The arenites are well stratified showing fine to coarse grained texture and fossiliferous content ((K)). In the upper part of the unit, grey silts and clays are interbedded.
The basal sequence ((B)) passes upward to a second sequence made up of calcarenites, mixed arenites and clays (CitationColella, 1995; CitationDi Nocera, Ortolani, Russo, & Torre, 1974; CitationLonghitano & Nemec, 2005; CitationMastandrea et al., 2002; CitationMattei et al., 2002; CitationMuto & Perri, 2002; CitationMuto et al., 2015). In particular, the calcarenite unit is made up of basal fossil-bearing breccia and conglomerates, evolving upward to massive, normal-graded sandstones and cross-stratified mixed, bioclastic/siliciclastic sandstones ((L)). The two sequences are separated by a strong erosional discontinuity and regional angular unconformity (CitationCritelli et al., 2017; CitationMuto et al., 2015).
Upward the sequence evolves to open marine deposits consisting of clays, marls and grey to black silts ((M)); in the study area the clay stratigraphic unit outcrops in limited flaps, among these the most extensive is located to the south-east of Lago town ((B)).
In the south-western sector of the study area ((B)), Middle to Late Pleistocene marine and continental terraced deposits (CitationMuto, Robustelli, Scarciglia, Spina, & Critelli, 2003; CitationRobustelli, Muto, Scarciglia, Spina, & Critelli, 2005; CitationTortorici et al., 2002; CitationWestaway, 1993) are well exposed ((N)).
Finally, widespread Holocene and actual alluvial deposits and debris and/or alluvial fans are found along the Licetto River valley ((B) and (O)).
From a tectonic perspective, during the late Neogene and Quaternary, the fold-and-thrust belt of northern Calabria experienced multiphase tectonic deformation and alternating transpressional and transtentional tectonic events (CitationBorrelli, Critelli, Gulla, & Muto, 2015; CitationBrutto et al., 2016; CitationLonghitano, Chiarella, & Muto, 2014; CitationMuto et al., 2015; CitationScarciglia et al., 2016; CitationSpina, Tondi, & Mazzoli, 2011; CitationTansi et al., 2007; CitationVan Dijk et al., 2000; CitationZecchin et al., 2013, Citation2015). During the Quaternary, a predominant extension phase affected the western domain of the Calabrian Arc and controlled the evolution of the Crati Basin and Coastal Range (CitationFabbricatore, Robustelli, & Muto, 2014; CitationLanzafame & Tortorici, 1981; CitationMuto et al., 2015).
In the study area, the major tectonic lineaments ((B)) are represented by both the intraformational thrust faults ((A)), which structured the tectono-metamorphic units of the chain, and the low-angle overthrust between the Metapelite Unit and Mt. Cocuzzo Unit ((I)). The so structured chain was cross-cut by three main high-angle fault systems striking NW-SE, NE-SW and N–S.
Figure 4. Examples of tectonic structures outcropping in the study area. (A) Flat lying thrust, duplexing the Metapelite Unit; (B) NW-SE trending left-lateral strike-slip fault; (C) NW-SE fault with left-lateral kinematics (slickenline in the inset); (D) N–S-oriented thrust in the Serravallian sandstone of the Amantea basin infill; (E) oblique slickenline on left-lateral NW-SE oriented fault; (F) fault damage zone constituted by cataclasite and breccia along the regional AGF zone; (G) NE-SW fault plane displacing the dolostone unit (footwall) and the phyllite unit (hanging-wall); (H) right-lateral transcurrent indicators; (I) dip-slip normal kinematic indicators; (J) Array of parallel normal faults in the high Licetto River valley; (K) particular of the spectacular N–S fault plane crossing the Mt. Cocuzzo Unit; (L) East dipping N–S normal faults involving the Metapelite Unit in the Pizzotto slope; (M) particular of a N–S fault plane in quartzite layers overprinted by slikenlines with dip-slip normal kinematics (in the inset); (N) N–S normal fault offsets a NW-SE strike-slip fault.
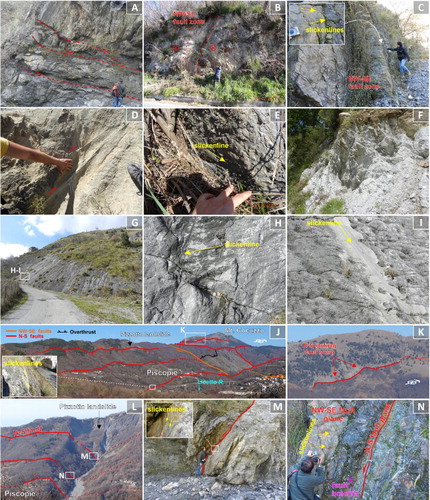
The NW-SE left-lateral strike-slip faults cross the whole study area ((B)), and two main first-order faults bound its northern (i.e. Falconara-Carpanzano Fault –FCF) and southern (i.e. Amantea-Gimigliano Fault – AGF) sectors ((A)) (CitationTansi et al., 2007). The major NW-SE oriented strike-slip faults ((B)) identify sub-vertical brittle shear zones ((B,C)) to which synthetic and antithetic strike-slip faults and high-angle thrusts ((D)) are associated. At the mesoscale, the fault planes strike from N120E to N145E, and show sub-vertical dip mostly towards the SW. Fault planes are characterized by horizontal slickensides documenting left-lateral strike-slip motions ((C,E)), often superimposed by sub-vertical slickensides indicating a normal displacement. The major faults of this system are characterized by pluri-kilometre length and consistent displacement which produced large damage fault zones consisting of breccias and cataclastite ((F)).
The NE-SW-oriented fault system is less represented than the others and is displaced by the N–S faults ((B)). Spectacular fault planes of this fault system outcrop along the northern and southern sectors of the Licetto valley. The major fault offsets the Metapelite Unit with respect to the Mt. Cocuzzo Unit ((G)); this fault, and the overall NE-SW system, is characterized by right-lateral strike-slip kinematics ((H)) superimposed by a dip-slip normal offset ((I)).
Since the Middle Pleistocene a generalized regional uplift affected the Coastal Range (and more generally throughout the western sector of the Calabrian Arc) (CitationFabbricatore et al., 2014; CitationTansi et al., 2007; CitationTortorici, Monaco, Tansi, & Cocina, 1995), and was accompanied by the development of N–S striking normal faults. As a result, the Mt. Cocuzzo ridge and the surrounding areas were affected by intense exhumation and high uplift rates.
The N–S-oriented faults, very frequent and pervasive in the study area ((B)), control the Licetto River valley course and displace the overthrust between the Metapelite and Mt. Cocuzzo Unit. They are morphologically represented by sharp and discontinuous rectilinear escarpments ((J,K)), and are locally marked by active alluvial fans (e.g. Pizzotto fan system, CitationSorriso-Valvo, Gullà, Antronico, Tansi, & Amelio, 1999), bounding the uplifted footwalls, where an antecedent drainage network flows perpendicular to the N–S fault segments ((B)). This fault system can be seen most clearly within the Pizzoto subcatchment ((B)), where an array of N–S trending, E-dipping normal faults are exposed on the E–W trending scarp face ((L)). However, the N–S fault planes show different slip directions and pitch, which are sometimes superimposed; the relative chronology of the kinematic indicators suggests that right transcurrent movements (evolving from strongly left-lateral to moderately right-lateral) postdate the last normal fault slip ((M,N)).
The regional uplift and recent N–S fault activity are responsible for rapid incision of the drainage network within the Licetto River basin and for abundant slope wasting processes, including several landslides and DSGSDs (CitationCalcaterra, Gambino, Borrelli, Muto, & Gullà, 2015; CitationSorriso-Valvo et al., 1999).
3. Geomorphological features and mass movement inventory map
The topographic relief of the Licetto River catchment () ranges from 0 m above sea level (at the Amantea coastal plain) to 1541 m of elevation (at the top of the Mt. Cocuzzo, one of the highest peaks of the southern Coastal Range).
The morphology () is very complex and strongly controlled by geology and tectonics (CitationMuto & Perri, 2002; CitationSorriso-Valvo et al., 1999). In particular, the Quaternary tectonic uplift and related deepening of the hydrographic network increased the relief energy – giving rise to steep slopes and deeply cut valleys in the bedrock (e.g. Licetto River and La Fiumarella stream) – and made the slopes prone to failure (CitationBonci et al., 2010; CitationCalcaterra et al., 2015; CitationSorriso-Valvo et al., 1999). Slope gradient depends on the hardness of the different lithological units. Steep scarps and cliffs consistently correspond to outcrops of harder rocks (e.g. Mesozoic dolostones, Late Miocene calcarenites, and Palaeozoic-Mesozoic foliated slates and quartzites belonging to the Metapelite Unit), generally forming scarps along the fault surfaces, including the main slope break which occurs at the upper 2/10th of the slope height ().
The mass movement inventory map () shows 824 landslides (of different type, size, age and state of activity), with an average density of about 16 landslides/km2. The inventory shows 523 slides (the most common type of landslide), 176 complex/compound phenomena and 36 shallow-medium-deep zones affected by creep processes. The mapped landslides range in size from AL = 2.4 × 102 m2 to AL = 4.5 × 105 m2, for a total landslide area of AL = 1.3 × 107 m2, corresponding to 33% of the whole study area. This datum, significantly larger than the other areas of Calabria, demonstrates the importance of landslides as a mass-wasting process in the Licetto catchment.
Figure 5. Mass movement inventory map of the Licetto River basin: (1) active debris fan; (2) dormant debris/alluvial fan; (3) alluvial deposits; (4) drainage network; (5) study area; (6) contour line; (7) main scarp; (8) slide; (9) complex; (10) creep; and (11) DSGSD of Sackung-type.
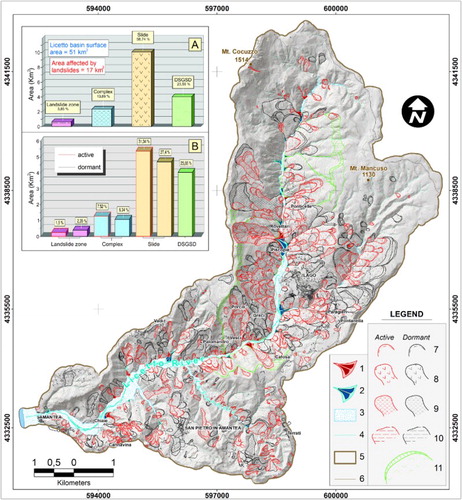
The effects of landslide activity are well exposed in the whole study area. Evidence of continuous creeping consists in the deformation and cracking of buildings and roads, distortion and cracking of road walls, and bending of trees. They assume the typical forms of steps and cuts that have been used as diagnostic evidence for the mass movement inventory map.
Visual inspection of the mass movement map () reveals high spatial persistence of medium-deep slope failures of different generations inside pre-existing (older) deep landslides. Deep landslides (largely classifiable as translational rock slide) are widespread along the middle sector of the Licetto River (e.g. around the Lago town) ( and (A–C)). Characteristic features of this type of mass movement are easily identified from aerial photographs through their effects on slope morphology, such as main scarps, secondary downhill- and uphill-facing scarps, counter slopes, landslide terraces, irregular slope profiles (undulation and swells of the topographic surface and convex or concave forms), changes in the drainage network and toe bulging (CitationCruden & Varnes, 1996; CitationHungr, Leroueil, & Picarelli, 2014; CitationHutchinson, 1968, Citation1988; CitationVarnes, 1978). The kinematic characteristics of some of these phenomena have been investigated by the measurements, carried out over a significant time-span, provided by an Integrated Monitoring Network (CitationBonci et al., 2010). In particular, deep landslides, located along the right side of the Licetto River (i.e. Greci-Piscopie slope, (A,B)) whose thickness was assessed by inclinometer measurements (CitationBonci et al., 2010), usually show very slow rates of displacement (Cruden and Varnes classification), multiple shear surfaces ranging in depth from 11 to 58 m (CitationCalcaterra et al., 2015), and a prevalent translational movement.
Figure 6. Examples of the typical mass movements widespread in the study area. (A–C) Panoramic view of deep slides affecting the Metapelite Unit along the Licetto River slopes; (D) general view of the Pizzotto complex landslide; (E) particular of the main scarp; (F) main landslide channel; (G) active debris fan at the toe of the Pizzotto landslide (note the upstream aggradation and the closure of the Licetto River); (H,I) other examples of complex landslide; and (J–L) panoramic view of the three Sackung-type DSGSDs identified in the study area.
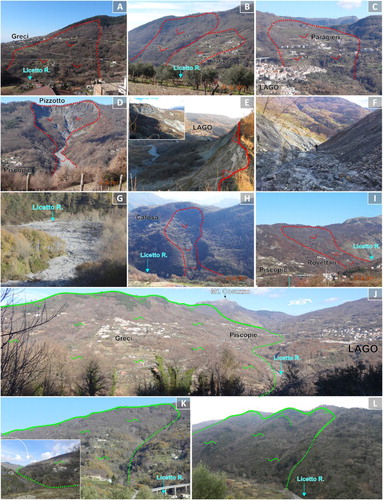
Furthermore, on the Greci-Piscopie slope (), there is a spectacular example of an active and complex instability phenomenon, the Pizzotto landslide-and-fan system (CitationSorriso-Valvo et al., 1999), characterized by an amphitheatre-shaped upper feeding zone ((D)), marked by a 950 m-wide scarp ((E)) from which rock masses are periodically detached along a strong incised channel ((F)) forming an active debris-flow fan whose deposits are encroaching the Licetto River stream ((G)). In 1951, as reported by CitationSorriso-Valvo et al. (1999), the active fan caused the temporary damming of the river and a conspicuous aggradation, suddenly followed by a 10-m incision evidenced by small terrace remnants on both sides of the Licetto valley. At present, the fan is in an aggradation phase only on the northern lobe ( and (G)).
More generally, complex/composite landslides are randomly widespread in the whole study area, and mainly located within the morphological hollows (), where colluvial/detrital cover fills hard/weak metamorphic or sedimentary rocks ((H)), or where weak and/or degraded, very fine-grained, metamorphic rocks show a steeply and downslope dipping foliation along which weakness planes develop ((I)). Characteristic features of these mass movements are gently hummocky topography and ridges of accumulated material in the toe area, often causing the deviation of river channels. Many complex-type landslides show elongated accumulation zones, ending with fan-shaped toes ().
Finally, three wide gravity-driven deformation areas (ranging in size from 1.1 × 106 to 3.5 × 106 m2), with diagnostic features linked to Sackung-type DSGSDs (CitationAgliardi, Crosta, & Zanchi, 2001; CitationDramis & Sorriso-Valvo, 1994) and partially masked by the superimposition of the ongoing landslides, were mapped ( and (J–L)). The typical observed morphological characteristics are: doubled ridges, scarps, counterscarps, trenches and toe bulging. Sackung-type DSGSDs, characterized by kinematic mechanisms strongly influenced by the structural setting, display a mature evolution stage (CitationNemčok, 1982), lack of well-defined rupture surfaces and show extremely slow rates of movement (e.g. CitationBonci et al., 2010; CitationCalcaterra et al., 2015).
The complex geo-structural setting of the Licetto River catchment (made by a geometrically complex system of high- and low-angle faults) plays a key role in controlling the geometry of the failure surface and its development. The high-angle faults often bound the main scarps and the flanks of deep-large landslides and DSGSDs, while, frequently, intraformational low-angle thrust faults (e.g. CitationBorrelli & Gullà, 2017) or foliation planes (dipping towards the Licetto valley) control the development of the failure surfaces, particularly within the low-grade metamorphic rocks (e.g. CitationChigira, Hariyama, & Yamasaki, 2013).
4. Conclusions
The paper presented the mass movement inventory map (Main Map), coupled with the geotectonic setting, for an area of 50 km2 in the Calabrian Coastal Range, Southern Italy. We produced the Main Map using conventional/consolidated methods, by means of multi-temporal aerial photo interpretation, analyses of Google Earth images and fieldwork. A total of 824 landslides (about 33% of the whole study area), of different types, states of activity and sizes, including some kilometre-scale DSGSDs were recognized. The mass movement types, distribution and shapes are strongly controlled by both the lithological characteristics and the multi history structural setting. About 40% of all the mapped landslides show a typical behaviour of active slow-moving landslides, commonly characterized by multiple and superimposed landslide bodies, confirming that the spatial distribution of the recent landslides is frequently influenced by the presence of older landslides. It is the authors’ opinion that the new mass movement inventory map of the Licetto River catchment represents a useful tool for territorial planning, and engineering-geological and environmental purposes in the study area. It is, also, the starting point for both quantitative landslide risk analyses and the design of the most appropriate risk-mitigation measures.
Software
The mass movement map and related layout (, , and Main map) were produced using Esri ArcGIS 10.0. Corel Draw X6 was used for compiling , and .
Geology and mass movements of the Licetto River catchment (Calabrian Coastal Range, Southern Italy).pdf
Download PDF (27 MB)Acknowledgements
This paper represents the scientific collaboration between the Italian National Research Council – Research Institute for Geo-Hydrological Protection (CNR-IRPI) – and the Department of Biology, Ecology and Earth Sciences (DiBEST), University of Calabria. The work was conducted under the Project DTA.AD003.077.001 ‘Tipizzazione di eventi di dissesto idrogeologico’ of the CNR Department of ‘Scienze del Sistema Terra e Tecnologie per l'Ambiente’. The authors thank Satoru Kojima, Gaetano Ortolano and Nick Scarle for their constructive comments that helped improve the manuscript and map.
Disclosure statement
No potential conflict of interest was reported by the authors.
References
- Agliardi, F., Crosta, G., & Zanchi, A. (2001). Structural constraints on deep-seated slope deformation kinematics. Engineering Geology, 59, 83–102. doi: 10.1016/S0013-7952(00)00066-1
- Amodio-Morelli, L., Bonardi, G., Colonna, V., Dietrich, D., Giunta, G., Ippolito, F., … Zuppetta, A. (1976). L’arco Calabro-Peloritano nell’orogene appenninico Maghrebide (The Calabrian-Peloritan Arc in the Apennine-Maghrebide orogen). Memorie – Società Geologica Italiana, 17, 1–60.
- Antronico, L., Borrelli, L., Coscarelli, R., & Gullà, G. (2015). Time evolution of landslide damages to buildings: The case study of Lungro (Calabria, Southern Italy). Bulletin of Engineering Geology and the Environment, 74, 47–59. doi: 10.1007/s10064-014-0591-y
- Antronico, L., Borrelli, L., Coscarelli, R., Pasqua, A. A., Petrucci, O., & Gullà, G. (2013). Slope movements induced by rainfalls damaging an urban area: The Catanzaro case study (Calabria, Southern Italy). Landslides, 10, 801–814. doi: 10.1007/s10346-013-0431-3
- Boardman, J. (2016). The value of Google Earth™ for erosion mapping. Catena, 143, 123–127. doi: 10.1016/j.catena.2016.03.031
- Bonci, L., Calcaterra, S., Cesi, C., Gambino, P., Gullà, G., Niceforo, D., … Sorriso-Valvo, M. (2010). Displacements on a slope affected by deep-seated gravitational slope deformation: Greci slope (Lago, Calabria, Italy). Geografia Fisica e Dinamica Quaternaria, 33, 141–153.
- Borrelli, L., Antronico, L., Gulla, G., & Sorriso-Valvo, G. M. (2014). Geology, geomorphology and dynamics of the 15 February 2010 Maierato landslide (Calabria, Italy). Geomorphology, 208, 50–73. doi: 10.1016/j.geomorph.2013.11.015
- Borrelli, L., Cofone, G., Coscarelli, R., & Gullà, G. (2015). Shallow landslides triggered by consecutive rainfall events at Catanzaro strait (Calabria-Southern Italy). Journal of Maps, 11, 730–744. doi: 10.1080/17445647.2014.943814
- Borrelli, L., Critelli, S., Gulla, G., & Muto, F. (2015). Weathering grade and geotectonics of the western-central Mucone River basin (Calabria, Italy). Journal of Maps, 11, 606–624. doi: 10.1080/17445647.2014.933719
- Borrelli, L., Gioffrè, D., Gullà, G., & Moraci, N. (2012). Susceptibility to shallow and rapid landslides in ground alteration: A possible contribution of propagation modeling. Rendiconti Online – Società Geologica Italiana, 21, 534–536.
- Borrelli, L., & Gullà, G. (2017). Tectonic constraints on a deep-seated rock slide in weathered crystalline rocks. Geomorphology, 290, 288–316. doi: 10.1016/j.geomorph.2017.04.025
- Brardinoni, F., Slaymaker, O., & Hassan, M. A. (2003). Landslide inventory in a rugged forested watershed: A comparison between air-photo and field survey data. Geomorphology, 54, 179–196. doi: 10.1016/S0169-555X(02)00355-0
- Brunsden, D. (1993). Mass movement; the research frontier and beyond: A geomorphological approach. Geomorphology, 7, 85–128. doi: 10.1016/0169-555X(93)90013-R
- Brutto, F., Muto, F., Loreto, M. F., De Paola, N., Tripodi, V., Critelli, S., & Facchin, L. (2016). The Neogene-quaternary geodynamic evolution of the central Calabrian Arc: A case study from the western Catanzaro trough basin. Journal of Geodynamics, 102, 95–114. doi: 10.1016/j.jog.2016.09.002
- Calcaterra, S., Gambino, P., Borrelli, L., Muto, F., & Gullà, G. (2015). Landslide activity and integrated monitoring network: The Greci slope (Lago, Calabria, Italy). Engineering Geology for Society and Territory, Landslide Processes, 2, 1065–1068. doi: 10.1007/978-3-319-09057-3_188
- Chigira, M., Hariyama, T., & Yamasaki, S. (2013). Development of deep-seated gravitational slope deformation on a shale dip-slope: Observations from high-quality drill cores. Tectonophysics, 605, 104–113. doi: 10.1016/j.tecto.2013.04.019
- Cirrincione, R., Fazio, E., Fiannacca, P., Ortolano, G., Pezzino, A., & Punturo, R. (2015). The Calabria-Peloritani Orogen, a composite terrane in Central Mediterranean; Its overall architecture and geodynamic significance for a pre-alpine scenario around the tethyan basin. Periodico di Mineralogia, 84(3B), 701–749.
- Ciurleo, M., Calvello, M., & Cascini, L. (2016). Susceptibility zoning of shallow landslides in fine grained soils by statistical methods. Catena, 139, 250–264. doi: 10.1016/j.catena.2015.12.017
- Ciurleo, M., Cascini, L., & Calvello, M. (2017). A comparison of statistical and deterministic methods for shallow landslide susceptibility zoning in clayey soils. Engineering Geology, 223, 71–81.
- Colella, A. (1995). Sedimentation, deformational events and eustacy in the perityrrhenian Amantea basin: Preliminary synthesis. Giornale di Geologia, 57, 179–193.
- Conforti, M., Muto, F., Rago, V., & Critelli, S. (2014). Landslide inventory map of north-eastern Calabria (South Italy). Journal of Maps, 10, 90–102. doi: 10.1080/17445647.2013.852142
- Critelli, S., Muto, F., Perri, F., & Tripodi, V. (2017). Interpreting provenance relations from sandstone detrital modes, southern Italy foreland region: Stratigraphic record of the Miocene tectonic evolution. Marine and Petroleum Geology doi: 10.1016/j.marpetgeo.2017.01.026
- Cruden, D. M., & Varnes, D. J. (1996). Landslide types and processes. In A. K. Turner & R. L. Schuster (Eds.), Landslides, investigation and mitigation (Transportation Research Board Special Report 247, pp. 36–75). Washington, DC: National Academy of Sciences.
- Di Nocera, S., Ortolani, F., Russo, M., & Torre, M. (1974). Successioni sedimentarie messiniane e limite Miocene-Pliocene nella Calabria settentrionale [Messinian sedimentary successions and Miocene-Pliocene boundary in northern Calabria]. Bollettino Della Società Geologica Italiana, 93, 575–607.
- Dramis, F., & Sorriso-Valvo, M. (1994). Deep-seated gravitational slope deformations, related landslides and tectonics. Engineering Geology, 38, 231–243. doi: 10.1016/0013-7952(94)90040-X
- Fabbricatore, D., Robustelli, G., & Muto, F. (2014). Facies analysis and depositional architecture of shelf-type deltas in the Crati basin (Calabrian Arc, South Italy). Italian Journal of Geosciences, 133, 131–148. doi: 10.3301/IJG.2013.19
- Fell, R., Corominas, J., Bonnard, C. H., Cascini, L., Leroi, E., Savage, W. Z., & on behalf of the JTC-1 Joint Technical Committee on Landslides and Engineered Slopes. (2008). Guidelines for landslide susceptibility, hazard and risk zoning for land use planning. Engineering Geology, 102, 85–98. doi: 10.1016/j.enggeo.2008.03.022
- Filice, F., Liberi, F., Cirillo, D., Pandolfi, L., Marroni, M., & Piluso, E. (2015). Geology map of the central area of Catena Costiera: Insights into the tectono-metamorphic evolution of the Alpine Belt in northern Calabria. Journal of Maps, 11(1), 114–125. doi: 10.1080/17445647.2014.944877
- Gullà, G., Peduto, D., Borrelli, L., Antronico, L., & Fornaro, G. (2017). Geometric and kinematic characterization of landslides affecting urban areas: The Lungro case study (Calabria, Southern Italy). Landslides, 14, 171. doi: 10.1007/s10346-015-0676-0
- Guzzetti, F., Mondini, A. C., Cardinali, M., Fiorucci, F., Santangelo, M., & Chang, K. T. (2012). Landslide inventory maps: New tools for an old problem. Earth-Science Reviews, 112, 42–66. doi: 10.1016/j.earscirev.2012.02.001
- Guzzetti, F., Stark, C. P., & Salvati, P. (2005). Evaluation of flood and landslide risk to the population of Italy. Environmental Management, 36, 15–36. doi: 10.1007/s00267-003-0257-1
- Hungr, O., Leroueil, S., & Picarelli, L. (2014). The Varnes classification of landslide types, an update. Landslides, 11, 167–194. doi: 10.1007/s10346-013-0436-y
- Hutchinson, J. N. (1968). Mass movement. In R.W. Fairbridge (Ed.), Encyclopedia of geomorphology (pp. 688–695). New York, NY: Reinhold Publishers.
- Hutchinson, J. N. (1988). General report: Morphological and geotechnical parameters of landslides in relation to geology and hydrogeology. In C. Bonnard (Ed.), Proceedings, fifth international symposium on landslides (Vol. 1, pp. 3–35). Rotterdam: Balkema.
- Ietto, A., & Ietto, F. (1998). Sviluppo e annegamento di un sistema carbonatico piattaforma-bacino nel Trias superiore della Catena Costiera Calabrese [Development and drowning of a platform-basin carbonate system in the Calabrian Coastal Chain during Late Triassic]. Bollettino Della Società Geologica Italiana, 117, 313–331.
- Lanzafame, G., & Tortorici, L. (1981). La tettonica recente del Fiume Crati (Calabria). Geografia Fisica e Dinamica Quaternaria, 4, 11–21.
- Longhitano, S. G., Chiarella, D., & Muto, F. (2014). Three-dimensional to two-dimensional cross-strata transition in the lower Pleistocene Catanzaro tidal strait transgressive succession (Southern Italy). Sedimentology, 61, 2136–2171. doi: 10.1111/sed.12138
- Longhitano, S. G., & Nemec, W. (2005). Statistical analysis of bed-thickness variation in a Tortonian succession of biocalcarenitic tidal dunes, Amantea Basin, Calabria, Southern Italy. Sedimentary Geology, 179, 195–224. doi: 10.1016/j.sedgeo.2005.05.006
- Mastandrea, A., Muto, F., Neri, C., Papazzoni, C. A., Perri, E., & Russo, F. (2002). Deep-water coral banks: An example from the ‘Calcare di Mendicino’ (Upper Miocene, northern Calabria, Italy). Facies, 47, 27–42. doi: 10.1007/BF02667704
- Mattei, M., Cipollari, P., Cosentino, D., Argentieri, A., Rossetti, F., Speranza, F., & Di Bella, L. (2002). The Miocene tectono-sedimentary evolution of the southern Tyrrhenian Sea: Stratigraphy, structural and palaeomagnetic data from the on-shore Amantea Basin (Calabrian Arc, Italy). Basin Research, 14, 147–168. doi: 10.1046/j.1365-2117.2002.00173.x
- Muto, F., Critelli, S., Robustelli, G., Tripodi, V., Zecchin, M., Fabbricatore, D., & Perri, F. (2015). A Neogene-Quaternary Geotraverse within the northern Calabrian Arc from the foreland peri-Ionian margin to the backarc Tyrrhenian margin. Geological Field Trips, 7(2.2), 1–65. doi: 10.3301/GFT.2015.04
- Muto, F., & Perri, E. (2002). Evoluzione tettono-sedimentaria del bacino di Amantea, Calabria occidentale [Tectonic-sedimentary evolution of the Amantea Basin, Western Calabria]. Bollettino Della Società Geologica Italiana, 121, 1–19.
- Muto, F., Robustelli, G., Scarciglia, F., Spina, V., & Critelli, S. (2003). Geomorphology, tectonics and sedimentology of Late Quaternary fans between Guardia Piemontese and Paola (Tyrrhenian Coast of Calabria, Southern Italy). Il Quaternario, Italian Journal of Quaternary Sciences, 16, 217–229.
- Muto, F., Spina, V., Tripodi, V., Critelli, S., & Roda, C. (2014). Neogene tectonostratigraphic evolution of allochthonous terranes in the Eastern Calabrian foreland (Southern Italy). Italian Journal of Geosciences, 133, 455–473. doi: 10.3301/IJG.2014.23
- Nemčok, A. (1982). Zosuvy v Slovenskych Karpatov [Landslides in the Slovak Carpathians]. Bratislava: Slovak Academy of Sciences.
- Ortolano, G., Cirrincione, R., Pezzino, A., Tripodi, V., & Zappala, L. (2015). Petro-structural geology of the Eastern Aspromonte Massif crystalline basement (Southern Italy-Calabria): An example of interoperable geo-data management from thin section – to field scale. Journal of Maps, 11(1), 181–200. doi: 10.1080/17445647.2014.948939
- Piluso, E., Cirrincione, R., & Morten, L. (2000). Ophiolites of the Calabrian Peloritan Arc and their relationships with the crystalline basement (Catena Costiera and Sila Siccola, Calabria, Southern Italy). Ofioliti, 25(2), 117–140.
- Rib, H. T., & Liang, T. (1978). Recognition and identification. In R. L. Schuster & R. J. Krizek (Eds.), Landslide analysis and control (Transportation Research Board Special Report 176, pp. 34–80). Washington, DC: National Academy of Sciences.
- Robustelli, G., Muto, F., Scarciglia, F., Spina, V., & Critelli, S. (2005). Eustatic and tectonic control on late Quaternary alluvial fans along the Tyrrhenian Sea coast of Calabria (South Italy). Quaternary Science Reviews, 24, 2101–2119. doi: 10.1016/j.quascirev.2004.08.025
- Scarciglia, F., Critelli, S., Borrelli, L., Coniglio, S., Muto, F., & Perri, F. (2016). Weathering profiles in granitoid rocks of the Sila Massif uplands, Calabria, southern Italy: New insights into their formation processes and rates. Sedimentary Geology, 336, 46–67.
- Schuster, R. L. (1996). Socioeconomic significance of landslides. In A. K. Turner & R. L. Schuster (Eds.), Landslides, investigation and mitigation (Transportation Research Board Special Report 247, pp. 12–35). Washington, DC: National Academy of Sciences.
- Sorriso-Valvo, M., Gullà, G., Antronico, L., Tansi, C., & Amelio, M. (1999). Mass movement, geologic structure and morphologic evolution of the Pizzotto-Greci slope (Calabria, Italy). Geomorphology, 30, 147–163. doi: 10.1016/S0169-555X(99)00051-3
- Sorriso-Valvo, M., & Tansi, C. (1996). Grandi frane e deformazioni gravitative profonde di versante della Calabria. Note illustrative per la carta al 250.000. Geografia Fisica e Dinamica Quaternaria, 19, 395–408.
- Spina, V., Tondi, E., & Mazzoli, S. (2011). Complex basin development in a wrench-dominated back-arc area: Tectonic evolution of the Crati Basin, Calabria, Italy. Journal of Geodynamics, 51, 90–109. doi: 10.1016/j.jog.2010.05.003
- Tansi, C., Folino Gallo, M., Muto, F., Perrotta, P., Russo, L., & Critelli, S. (2016). Seismotectonics and landslides of the Crati Graben (Calabrian Arc, Southern Italy). Journal of Maps, 12, 363–372. doi: 10.1080/17445647.2016.1223760
- Tansi, C., Muto, F., Critelli, S., & Iovine, G. (2007). Neogene-quaternary strike-slip tectonics in the central Calabrian Arc (Southern Italy). Journal of Geodynamics, 43, 393–414. doi: 10.1016/j.jog.2006.10.006
- Tortorici, G., Bianca, M., Monaco, C., Tortorici, L., Tansi, C., De Guidi, G., & Catalano, S. (2002). Quaternary normal faulting and marine terracing in the area of Capo Vaticano and S. Eufemia plain (Southern Calabria). Studi Geologici Camerti, 1, 155–171.
- Tortorici, L., Monaco, C., Tansi, C., & Cocina, O. (1995). Recent and active tectonics in the Calabrian Arc (Southern Italy). Tectonophysics, 243, 37–55. doi: 10.1016/0040-1951(94)00190-K
- Van Dijk, J. P., Bello, M., Brancaleoni, G. P., Cantarella, G., Costa, V., Frixa, A., … Zerilli, A. (2000). A regional structural model for the northern sector of the Calabrian Arc (Southern Italy). Tectonophysics, 324, 267–320. doi: 10.1016/S0040-1951(00)00139-6
- Varnes, D. J. (1978). Slope movement types and processes. In R. L. Schuster & R. J. Krizek (Eds.), Landslides, analysis and control (Transportation Research Board Special Report 176, pp. 11–33). Washington, DC: National Academy of Sciences.
- Vespasiano, G., Apollaro, C., Muto, F., Dotsika, E., De Rosa, R., & Marini, L. (2014). Chemical and isotopic characteristics of the warm and cold waters of the Luigiane Spa near Guardia Piemontese (Calabria, Italy) in a complex faulted geological framework. Applied Geochemistry, 41, 73–88. doi: 10.1016/j.apgeochem.2013.11.014
- Westaway, R. (1993). Quaternary uplift of Southern Italy. Journal of Geophysical Research: Solid Earth, 98, 21741–21772. doi: 10.1029/93JB01566
- Zecchin, M., Caffau, M., Di Stefano, A., Maniscalco, R., Lenaz, D., Civile, D., … Critelli, S. (2013). The Messinian succession of the Crotone Basin (southern Italy) II: Facies architecture and stratal surfaces across the Miocene–Pliocene boundary. Marine and Petroleum Geology, 48, 474–492. doi: 10.1016/j.marpetgeo.2013.08.013
- Zecchin, M., Praeg, D., Ceramicola, S., & Muto, F. (2015). Onshore to offshore correlation of regional unconformities in the Plio-Pleistocene sedimentary successions of the Calabrian Arc (central Mediterranean). Earth-Science Reviews, 142, 60–78. doi: 10.1016/j.earscirev.2015.01.006