ABSTRACT
A few hydrogeological studies have been carried out worldwide in peridotite aquifer systems, despite their wide distribution. The ophiolites are one of the main groundwater reservoir within the northern Apennines (Italy). This paper suggests the graphical solution to set the hydrogeological map of heterogeneous, multi-layered ophiolitic aquifers mapped on large scale (1:1600). The site investigation area is an ophiolite outcrop of the External Ligurian of the northern Apennines: the Mountain Prinzera rock complex area (44°38′30″N, 10°5′E; Parma Province, Emilia-Romagna Region). The hydrogeological characteristics of the tested aquifer system do not allow setting a hydrogeological map by applying usual graphical approaches. The hydrogeological map in such complex aquifer systems will show the classic hydrogeological data but must put in evidence above all (i) the main heterogeneities of the system, from the hydraulic point of view and (ii) the modifications of groundwater scenarios and pathways over time. The hydrogeological database of Mt Prinzera aquifer was managed in ESRI ArcGIS 10.0 software.
1. Introduction
Ophiolites are mostly composed of hard rocks as basalt, gabbro, serpentinite and peridotite and represent fragments of upper mantle and oceanic crust which are widespread on the surface of continents: from the Iberian Peninsula to the Himalayas, through Cyprus, Syria, Oman, and in Cuba, Papua-New Guinea, New Caledonia, Newfoundland (CitationAbbate et al., 1986; CitationAzor et al., 2008; CitationDilek & Furnes, 2011; CitationDilek & Furnes, 2014; CitationFonseca et al., 1999; CitationFonseca & Ribeiro, 1993).
The ophiolite bodies of the Alpine and Apennine belt are oceanic lithospheric remnants of the Ligure-Piemontese basin, developed in the Middle to Upper Jurassic, separating the Europe plate from the Adria plate (CitationMarroni, Meneghini, & Pandolfi, 2010). In the suture zone of the Western Alps and Alpine Corsica, the ophiolites have undergone metamorphism under HP-conditions during the Alpine subduction and collision (CitationDal Piaz, Bistacchi, & Massironi, 2003), while in the northern Apennines the oceanic lithosphere is found within the Ligurian tectonic units as obducted ophiolites without significant metamorphic signature (CitationAbbate et al., 1986).
In the northern Apennine the ophiolites outcrop in two palaeogeographic domains (see tectonic sketch in the Main Map): the Internal ligurides and External ligurides, which are identified on the basis of their current structural characteristics and their relationships with the associated sedimentary sequences (CitationAbbate et al., 1986; CitationAzor et al., 2008; CitationBortolotti, Principi, & Treves, 2001; CitationElter, 1972; CitationFonseca et al., 1999; CitationFonseca & Ribeiro, 1993; CitationMarroni & Tribuzio, 1996). Internal ligurides contain peridotites, serpentinites, gabbros and basalts, which are the bedrock of the Upper Jurassic – Paleocene sedimentary sequence deposits. External ligurides, on the other hand, contain ophiolitic sequences that occur as huge and large slide blocks in Upper Cretaceous sedimentary melanges (CitationAbbate et al., 1986). Many olistolithes of basalts and peridotites are embedded in prevalent pelitic rock complexes of External ligurian units which represent the stratigraphic bed of the Upper Cretaceous Helminthoid Flysch (CitationElter, 1972; CitationElter, Marroni, Molli, & Pandolfi, 1991; CitationMarroni et al., 2010).
At regional scale, the Mt Prinzera is an ophiolite outcrop of the External ligurian units of the northern Apennines area and has been the subject of several studies that have investigated its geological, geomorphological and structural characteristics (CitationChelli, Segadelli, Vescovi, & Tellini, 2016; CitationDi Dio, Martini, Lasagna, & Zanzucchi, 2005 and references therein; CitationGiammetti, 1964; CitationPagani et al., 1972; CitationRicci Lucchi, 1990; CitationServizio Geologico d’Italia, 1968; CitationVenturelli, Contini, Bonazzi, & Mangia, 1997; CitationZanzucchi, 1980).
From the hydrogeological point of view, a few studies have been carried out in ophiolitic media worldwide (e.g. CitationBoronina, Balderer, Renard, & Stichler, 2005; CitationBoronina, Renard, Balderer, & Christodoulides, 2003; CitationCritelli et al., 2015; CitationDewandel et al., 2005; CitationDewandel, Lachassagne, & Qatan, 2004; CitationDewandel, Lachassagne, Bakalowicz, Weng, & Al, 2003; CitationJoin, Robineau, Ambrosi, Costis, & Colin, 2005; CitationMarques et al., 2008; CitationNikic et al., 2013; CitationSegadelli et al., 2017), contrary to what is observed in the scientific literature focused on other types of aquifer systems made up of other rocks of magmatic origin (e.g. CitationBinet, Guglielmi, Bertrand, & Mudry, 2007; CitationCruz & Silva, 2001; CitationDavis & Turk, 1964; CitationDewandel et al., 2005; CitationDewandel, Lachassagne, Maréchal, Wyns, & Krishnamurthy, 2006; CitationGustafson & Krásný, 1994; CitationLachassagne et al., 2001; CitationLachassagne et al., 2009; CitationSander, 1997; CitationWalker, Holdsworth, Armitage, & Faulkner, 2013; CitationWright, 1992).
However, the ophiolites are one of the main groundwater reservoir within the northern Apennines (CitationGargini, De Nardo, Piccinini, Segadelli, & Vincenzi, 2014). Therefore, an experimental study (CitationSegadelli et al., 2017) has been carried out recently in the test site of Mt Prinzera, in order to define an effective hydrogeological conceptual model. In particular, the hydrogeological, hydrochemical, isotopic and biomolecular investigations have pointed out the existence of a heterogeneous system, where different groundwater bodies coexist and interact, therefore feeding both perennial and seasonal springs. Moreover, the deeper aquifer system is compartmentalized, due to faults dislocating the base aquitard.
Taking into account the results of the experimental research, the present work has been focused on the devices that are necessary to set an effective hydrogeological map in these heterogeneous and multi-layered ophiolitic systems.
2. Test site
The Mt Prinzera (Lat. 44°38′30″N, Long. 10°5′E, 725 m a.s.l.) consists of serpentinized mantle tectonites (CitationDi Dio et al., 2005; CitationGiammetti, 1964; CitationVenturelli et al., 1997) and appears as an orographic culmination of the External ligurid units of the northern Apennines (see tectonic sketch in the Main Map). It is located about 36 km SW of the town of Parma (Emilia-Romagna Region, Italy), near the confluence of the Taro River and Ceno Stream. The ultramafic olistolith of the Mt Prinzera covers an area of about 0.94 km2 (see geological map and geological cross section in the Main Map).
The Mt Prinzera consists mainly of strongly serpentinized ultramafites that have a very low primary permeability but being extensively fractured. Due to the compositional heterogeneity of its vertical sequence, the ultramafic medium is made of five lithological units, tectonically overlapped (; modified from CitationSegadelli et al., 2017). Between them, a low-permeability, discontinuous unit has been identified in a wide portion of the system. This unit behaves as an aquitard.
Figure 1. Schematic representation of the five units identified in the ultramafic medium. The red line separate lithologic units with homogeneous characteristics (modified from CitationSegadelli et al., 2017).
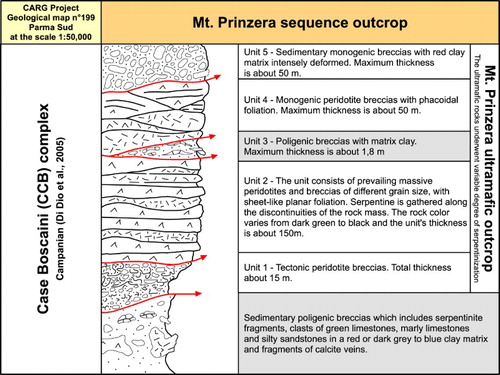
In the ultramafic outcrop, several deformation phases were recognized (CitationSegadelli et al., 2017). The last deformation shows a clear extensional kinematic with high-angle faults (dips of the fault planes 50°–70°), which cut across the entire ultramafic sequence. The normal faults characterized by greater offset can reach and displace the underlying impermeable unit, compartmentalizing the deep aquifer from the hydraulic point of view. The horizontal heterogeneity of the hydrostructure is strongly influenced by the presence of these normal faults.
The ophiolite outcrop is about 250 m thick and the hydrostructure is gently dipping to the northwest.
The ultramafites of Mt Prinzera are bordered by low-permeability deposits which are predominantly characterized by polygenic breccias (Case Boscaini complex, Campanian age. CitationDi Dio et al., 2005), made of blocks of limestones or marly limestones immersed in a fine-grained matrix or a mineral cement.
Nine seasonal and six perennial springs have been identified in the Mt Prinzera area. The former are located within the serpentinized peridotites, whilst the latter are located along the contact between the ophiolitic medium and the low-permeability rocks, being controlled by the compartmentalization of the system, due to high-angle tectonic discontinuities.
3. Materials and methods
The hydrogeological survey was carried out from May 2012 to July 2013. The location and elevation of each spring point have been measured using a global positioning system receiver. Complementary data are available from the geological survey database. Labile parameters (i.e. temperature, electric conductivity at 25°C, pH and discharge measurements) were collected onsite by means of portable tools/devices (Eutech Instrument: Cond 6+ model and pH6+ model). The spring discharge was monitored on a daily or weekly basis. Electrical conductivity (EC), temperature and pH of spring waters were measured on the same regular basis.
Precipitation amounts and air temperature data were provided by meteorological station arrays from Dexter3r service (http://www.smr.arpa.emr.it/dext3r/). Local rainfall were collected at three stations located at different elevations.
The chemical analyses (major and minor elements) were carried out twice, during the low- and high-flow periods. Stable isotope analyses (δ18O and δ2H) were carried out on a monthly basis for both spring- and rainwaters from May 2012 to July 2013. In particular, stable isotope content was analyzed on a daily or weekly basis, from January to March 2013, for four springs (P01, P02, P03, and P04b).
A detailed mapping survey (scale 1:2.500) was carried out from March 2011 to October 2011 over an area of about 1 km2 in order to characterize the geological parameters inferred to control the hydrogeologic behavior of the system, with emphasis on both lithologic and tectonic discontinuities. The results of this fieldwork activity helped to refine the available geological map (CitationDi Dio et al., 2005) based on CARG (Italian Geological Cartography Project) data.
To better characterize the fracture network and to quantitatively evaluate the parameters influencing the groundwater flow, discrete structural attributes (e.g. fault-fracture frequencies, number of fracture sets, persistence, aperture and spatial distribution) were collected along a 73-m-long scan-line crossing at least three of the major normal fault zones and water-conductive tectonic lineaments, identified during the geological mapping, and hosting one of the monitored seasonal springs (CitationSegadelli et al., 2017).
The hydrogeological complexes have been obtained by grouping the lithologies of the geological map (CitationSegadelli et al., 2017) and by considering their relative permeability. Green colors have been adopted to show the higher permeability complexes, while grey ones describe the lower permeability complexes.
The hydrogeological cross sections have been produced along the lines of the corresponding geological sections shown on the geological map of Mt Prinzera (CitationSegadelli et al., 2017).
The contour lines related to the bottom of the perched aquifer have been obtained manually resorting to a triangulation method, and then digitalized.
The basic map elements have been taken from the contents of topographic database of the Emilia-Romagna region. This cartography is made of the following themes: (i) contour line at 5 m and index contour at 25 m, (ii) spot elevation, (iii) building and main roads and (iv) raster digital images. For more information:
The hydrogeological symbols used on the Main Map are in agreement with the recommendations reported in the Italian Official Guidelines for hydrogeological survey and representation (CitationServizio Geologico d’Italia, 1995).
The hydrogeological and topographic database of the Emilia-Romagna region has been managed in ESRI. 2011. ArcGIS Desktop 10.0. Redlands, CA. Environmental Systems Research Institute. All these information are available in vector digital format and organized in a database in ArcGIS 10.0 and georeferenced in World Geodetic System 1984 (WGS 84 datum) and the metric coordinates reported in the Main Map area refer to the UTM 32N projection zone.
4. Hydrogeological setting
4.1. Hydrogeological units
Considering their hydraulic features, the lithologic units, which have been identified within the study area (), have been grouped into four hydrogeological units.
Quaternary covers: due to the absence of vegetation cover, quaternary deposits influence runoff and infiltration processes, and then they are of utmost importance in governing the aquifer recharge; their high permeability is due to primary porosity.
Serpentinized peridotites breccia with phacoidal foliation (PPF, units 4 and 5 in ): this unit is made of prevailing breccias of different grain size, with also phacoidal foliation; their high permeability is mainly due to interconnected fractures; the whole thickness is about 50 m; due to the contrast in permeability at the bottom of this unit, retention of percolation and storage of water in a perched temporary aquifer are observed.
Peridotites with sheet-like planar foliation (PSF, units 1 and 2 in ): this unit is made of prevailing massive peridotites with sheet-like planar foliation; their high permeability is mainly due to interconnected fractures; the whole thickness is about 165 m (); this unit is the main and deeper aquifer of the hydrogeological system.
Poligenic breccias (PB); this unit is made of centimetric to decimetric clasts of limestones, marly limestones, silty sandstones and ophiolites immersed in a clayey matrix; it is characterized by low permeability and behaves as an aquitard; within the PB unit two different geologic units have been grouped: (i) a shallower and thinner unit (unit 3 in ) interposed between the PPF and PSF units () and (ii) a deeper and thicker unit (sedimentary polygenic breccias in ) underlying and surrounding the ophiolitic hydrostructure ().
4.2. Springs and hydrogeological behavior of the system
The conceptual hydrogeological model of Mt Prinzera aquifers is described in CitationSegadelli et al. (2017), . Several springs have been identified within the test area, therefore showing a significant heterogeneity of the system from the hydraulic point of view. Both perennial and seasonal springs coexist. The perennial springs flow out along the contact between the ophiolitic massif and the surrounding low-permeability rocks. On the contrary, the seasonal springs can be found only within the western part of the hydrostructure and flow out at higher altitudes, where peridotites with phacoidal foliation crop out.
Figure 5. Hydrogeologic conceptual model of the Mt Prinzera aquifer system. Legend – 1: ultramafic aquifer; 2: aquiclude; 3: discontinuous aquitard; 4: fault; 5: fracture; 6: perched groundwater phreatic surface; 7: basal groundwater piezometric surface; 8: flow line of the perched groundwater; 9: flow line of the basal groundwater; 10: springs and their code; 11: infiltration within the unsaturated ultramafic medium.
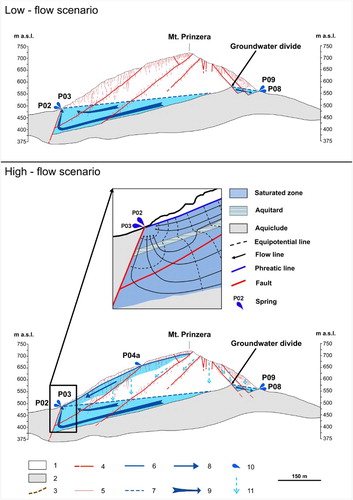
The type and the distribution of the springs are due to both layered and discontinuous heterogeneity within the system.
The seasonal high-altitude springs are fed by shallow perched groundwater. This groundwater flows into the PPF unit, above the lower permeability PB unit, which gently dips westwards. The PB unit is characterized by spatial discontinuity, therefore allowing some percolation towards the deeper groundwater. These springs flow out during the wet seasons and their discharge (up to some liters per second) rapidly responds to rainfall. The discharge progressively increases along the valleys (see seasonal springs discharge in the Main Map), and then, during the high-flow periods, new seasonal outflows are observed along the border of the ophiolitic massif. These springs, when active, are seasonally fed also by the deeper groundwater, therefore inducing cyclic modifications of its flow pathway. The high-altitude springs are characterized by (CitationSegadelli et al., 2017): (i) low EC (EC up to 225 µS/cm), (ii) nearly neutral pH, (iii) temperature ranging according to that of the atmosphere, (iv) inverse relationship between springs discharge versus EC and (v) isotopic signature (oxygen and deuterium) strictly linked to that of the local rainwater. Moreover, the tritium content is the same detected in local rainwater (about 8 TU). On the whole, there is a short time lag between effective infiltration and arrival of fresh-infiltration water at the high-altitude springs.
The perennial springs are mainly fed by the deeper groundwater. This groundwater flows into the PSF unit, above the deeper PB unit. These springs have a discharge ranging from a few to several liters per second, and rapidly respond to rainfall. However, in that case, springs water are characterized by (CitationSegadelli et al., 2017): (i) higher EC (up to 330 µS/cm) and (ii) pH (up to 11), (iii) temperature fluctuations smoothed with respect to that of the atmosphere, (iv) direct relationship between springs discharge versus EC and (v) stable isotopic signature (oxygen and deuterium). The mean residence time of this deeper groundwater is significantly greater than that of the perched one, as confirmed by the tritium content (usually, a few tritium units [TUs]). Concerning the hyperalkaline spring, several tens of TU have been detected, therefore suggesting waters correlated with the nuclear explosions occurred between the ‘40th and ‘60th. Thus, the springs hydrographs, which looks like those of the seasonal springs, are mainly influenced by the hydraulic head increase due to the arrival of fresh-infiltration water at the groundwater surface. The existence (during both the low-flow and high-flow periods) of several perennial springs along the contact between the ophiolitic structure and the surrounding aquitard (at the north-western and the south-eastern ends) is due to the compartmentalization of the system, that is caused by normal faults displacing the base aquitard.
5. Hydrogeological mapping strategy
In light of its static and dynamic features, the application of usual graphical approaches in preparing a hydrogeological map for the study area would result as uncomplete and uneffective. Therefore, in such complex aquifer systems, the classic hydrogeological mapping approaches (e.g. CitationChaminé, 2015; CitationChaminé, Carvalho, Teixeira, & Freitas, 2015; CitationDesiderio, Folchi Vici D’arcevia, Nanni, & Sergio Rusi, 2012; CitationLa Vigna et al., 2016; CitationManca, Viaroli, & Mazza, 2017; CitationMargat & van der Gun, 2013; CitationServizio Geologico d’Italia, 1995; CitationStruckmeier & Margat, 1995) must be coupled with a new graphical solution, as to describe the main hydraulic heterogeneities, as well as the modifications of the groundwater scenarios and pathways over time. The following devices to solve these requirements have been here adopted and proposed.
The hydrogeological map was designed with two different sketches, which share the same static hydrogeological features, but depicting the dynamic behavior under the low- and high-flow conditions, respectively. The two sketches describe, superimposed to the same hydrogeological base, the two extreme seasonal conditions for the groundwater flow.
In light of the great importance of the role played by the shallower aquitard in controlling the coexistence of the two groundwater bodies during the high-flow periods, the same aquitard must be identified and emphasized in terms of thickness, so to clearly explain the seasonal high-altitude springs. Obviously, the high-flow scenario sketch results as more complex, as the seasonal springs and the flow lines related to the perched groundwater are overlapped to the perennial springs and the flow lines related to the deeper groundwater.
In light of the complex hydrogeological behavior of the system, as well as of the coexistence of more and less prolonged pathways within the deeper aquifer, each of the two sketches must be linked to a hydrogeological section. These sections must show the main heterogeneities within the system, visualize the coexistence of perched and deeper groundwater, and schematize the possibility of groundwater pathways with different mean residence time, so to justify the coexistence of perennial springs characterized by significant differences in terms of physico-chemical and isotopic features.
6. Discussion and concluding remarks
Concerning the specific case of Italian ophiolites, the technical solution here proposed can be applied in other ophiolite outcrops of the northern Apennines that belong to external ligurian units where the ophiolites are present as gravity-slide blocks in Upper Cretaceous sedimentary mélanges. Instead, as mentioned in the introduction, the internal ligurian units represent an ophiolite sequence of Jurassic age with overlying sedimentary cover which includes pelagic, trench and lower slope deposits ranging in age from Late Jurassic to Paleocene (CitationMarroni et al., 2010). The internal ligurian ophiolite nappe occurs at the uppermost and westernmost position in the northern Apennine belt and is not incorporated by cretaceous low-permeability deposits (polygenic breccias in fine-grained matrix). For this reason, the approach proposed for the Mt Prinzera ophiolitic aquifer system could be not applicable, in its present form, for the internal ligurian ophiolites.
In a wider context, the approach proposed for the Mt Prinzera ophiolitic aquifer system can be applied also in hydrogeological settings made of different lithology. As a matter of fact, the solutions tested with this map can be used in an easy way in those systems where lithological and/or tectonic discontinuities cause the system to be compartmentalized and/or multi-layered. This is the case, for example, of the classic karst aquifers, where epikarstic horizons cause the temporary retention of percolation and storage of water in a perched aquifer above the deeper one.
Software
The hydrogeological database was managed in ESRI. 2011. ArcGIS Desktop 10.0. Redlands, CA. Environmental Systems Research Institute.
Main_Map_Rev02.pdf
Download PDF (45.5 MB)Main_Map_Rev02_changes_highligted.pdf
Download PDF (35.5 MB)Acknowledgements
The authors gratefully acknowledge the Associate Editor Tommaso Piacentini and the three reviewers Steven Bernard, Helder Chaminé, Sara Taviani for detailed and helpful suggestions and recommendations. The authors also acknowledge Prof. Giuliana Villa (University of Parma) for the age determination of Sedimentary polygenic breccias through biostratigraphic analysis, and Prof. Paola Iacumin and Dr Enrico Selmo (University of Parma) for the isotopic analyses.
Disclosure statement
No potential conflict of interest was reported by the authors.
ORCID
Alessandro Chelli http://orcid.org/0000-0001-5035-733X
References
- Abbate, E., Bortolotti, V., Conti, M., Marcucci, M., Principi, G., Passerini, P., & Treves, B. (1986). Apennines and Alps ophiolites and the evolution of the Western Tethys. Memorie della Società Geologica Italiana, 31, 23–44.
- Azor, A., Rubatto, D., Simancas, J. F., González Lodeiro, F., Martínez Poyatos, D., Martín Parra, L. M., & Matas, J. (2008). Rheic Ocean ophiolitic remnants in southern Iberia questioned by SHRIMP U-Pb zircon ages on the Beja-Acebuches amphibolites. Tectonics, 27, TC5006. doi: 10.1029/2008TC002306
- Binet, S., Guglielmi, Y., Bertrand, C., & Mudry, J. (2007). Unstable rock slope hydrogeology: Insights from the large-scale study of western Argentera-Mercantour hillslopes (south-east France). Bulletin de la Societe Geologique de France, 178, 159–168. doi: 10.2113/gssgfbull.178.2.159
- Boronina, A., Balderer, W., Renard, P., & Stichler, W. (2005). Study of stable isotopes in the Kouris catchment (Cyprus) for the description of the regional groundwater flow. Journal of Hydrology, 308, 214–226. doi: 10.1016/j.jhydrol.2004.11.001
- Boronina, A., Renard, P., Balderer, W., & Christodoulides, A. (2003). Groundwater resources in the Kouris catchment (Cyprus): Data analysis and numerical modelling. Journal of Hydrology, 271, 130–149. doi: 10.1016/S0022-1694(02)00322-0
- Bortolotti, V., Principi, G., & Treves, B. (2001). Ophiolites, Ligurides and the tectonic evolution from spreading to convergence of a Mesozoic Western Tethys segment. In G. B. Vai & I. P. Martini (Eds.), Anatomy of an orogen: The Apennine and adjacent Mediterranean basins (pp. 151–164). London: Kluwer Academic Publishers.
- Chaminé, H. I. (2015). Water resources meet sustainability: New trends in environmental hydrogeology and groundwater engineering. Environmental Earth Sciences, 73, 2513–2520. doi: 10.1007/s12665-014-3986-y
- Chaminé, H. I., Carvalho, J. M., Teixeira, J., & Freitas, L. (2015). Role of hydrogeological mapping in groundwater practice: Back to basics. European Geologist Journal, 40, 34–42.
- Chelli, A., Segadelli, S., Vescovi, P., & Tellini, C. (2016). The geomorphological mapping as a tool in the groundwater study: The case of Mt. Prinzera rock aquifers (northern Apennines, Italy). Journal of Maps, 12(5), 770–776. doi: 10.1080/17445647.2015.1072115
- Critelli, T., Vespasiano, G., Apollaro, C., Muto, F., Marini, L., & De Rosa, R. (2015). Hydrogeochimical study of an ophiolitici aquifer: A case stusy of Lago (Southern Italy, Calabria). Environmental Earth Sciences, 74, 533–543. doi: 10.1007/s12665-015-4061-z
- Cruz, J. V. & Silva, M. O. (2001). Hydrogeologic framework of Pico Island, Azores, Portugal. Hydrogeology Journal, 9, 177–189. doi: 10.1007/s100400000106
- Dal Piaz, G. V., Bistacchi, A., & Massironi, M. (2003). Geological outline of the Alps. Episodes, 26, 175–180.
- Davis, S. N., & Turk, L. J. (1964). Optimun depth of wells in crystalline rocks. Ground Water, 2, 6–11. doi: 10.1111/j.1745-6584.1964.tb01750.x
- Desiderio, G., Folchi Vici D’arcevia, C., Nanni, T., & Sergio Rusi, S. (2012). Hydrogeological mapping of the highly anthropogenically influenced Peligna Valley intramontane basin (Central Italy). Journal of Maps, 8, 165–168. doi: 10.1080/17445647.2012.680778
- Dewandel, B., Lachassagne, P., Bakalowicz, M., Weng, P., & Al, M. (2003). Evaluation of aquifer thickness by analyzing recession hydrographs. Application to the Oman ophiolite hard-rock aquifer. Journal of Hydrology, 274, 248–269. doi: 10.1016/S0022-1694(02)00418-3
- Dewandel, B., Lachassagne, P., Boudier, F., Al-Hattali, S., Ladouche, B., Pinault, J. L., & Al-Suleimani, Z. (2005). A conceptual hydrogeological model of ophiolite hard-rock aquifers in Oman based on a multiscale and a multidisciplinary approach. Hydrogeology Journal, 13, 708–726. doi: 10.1007/s10040-005-0449-2
- Dewandel, B., Lachassagne, P., Maréchal, J. C., Wyns, R., & Krishnamurthy, N. S. (2006). A generalized 3-D geological and hydrogeological conceptual model of granite aquifer controlled by single or multiphase weathering. Journal of Hydrology, 330, 260–284. doi: 10.1016/j.jhydrol.2006.03.026
- Dewandel, B., Lachassagne, P., & Qatan, A. (2004). Spatial measurements of stream baseflow, a relevant method for aquifer characterization and permeability evaluation. Application to a hard-rock aquifer, the Oman ophiolite. Hydrological Processes, 18, 3391–3400. doi: 10.1002/hyp.1502
- Di Dio, G., Martini, A., Lasagna, S., & Zanzucchi, G. (2005). Note illustrative della Carta Geologica d’Italia alla scala 1:50.000, Foglio n° 199 Parma Sud-Ovest. [Sheet n° 199 Parma Sud-Ovest, Servizio Geologico della Regione Emilia-Romagna, Servizio Geologico Nazionale, ISPRA, Rome].
- Dilek, Y. & Furnes, H. (2011). Ophiolite genesis and global tectonics: Geochemical and tectonic fingerprinting of ancient oceanic lithosphere. Geological Society of America Bulletin, 123, 387–411. doi: 10.1130/B30446.1
- Dilek, Y., & Furnes, H. (2014). Ophiolites and their origins. Elements, 10, 93–100. doi: 10.2113/gselements.10.2.93
- Elter, P. (1972). La zona ofiolitifera del Bracco nel quadro dell’Appennino Settentrionale. Introduzione alla geologia delle Liguridi [The Bracco ophiolitic area in the northern Apennines. Introduction to the geology of the Ligurian Units]. 66° Congr. Soc. Geol. It., Guida alle escursione, Pacini editore, Pisa, 35 pp.
- Elter, P., Marroni, M., Molli, G., & Pandolfi, L. (1991). Le caratteristiche stratigrafiche del Complesso di M.Penna/Casanova (Alta Val Trebbia, Appennino settentrionale) [The stratigraphic characteristics of the Mt. Penna/Casanova Complex (Trebbia Valley, northern Apennines, Italy]. Atti Tic. Sc. Terra, 34, 97–106.
- Fonseca, P. E., Munhá, J., Pedro, J. C., Moita, P., Araújo, A., Rosas, F., & Leal, N. (1999). Variscan ophiolites and high-pressure metamorphism in Southern Iberia. Ofioliti, 24, 259–268.
- Fonseca, P. E. & Ribeiro, A. (1993). The tectonics of Beja-Acebuches ophiolite: A major suture in the Iberian Variscan Fold Belt. Geologische Rundschau, 82, 440–447. doi: 10.1007/BF00212408
- Gargini, A., De Nardo, M. T., Piccinini, L., Segadelli, S., & Vincenzi, V. (2014). Spring discharge and groundwater flow systems in sedimentary and ophiolitic hard rock aquifers: Experiences from northern Apennines (Italy). In J. M. Sharp (Ed.), Fractured rock hydrogeology (pp. 129–146). Balkema: IAH Series n°20, CRC press.
- Giammetti, F. (1964). Le serpentine del Monte Prinzera [The Mt. Prinzera serpentinites]. . Memorie della Società Geologica Italiana, 4, 283–303.
- Gustafson, G. & Krásný, J. (1994). Crystalline rock aquifers: Their occurrence, use and importance. Hydrogeology Journal, 2, 64–75. doi: 10.1007/s100400050051
- Join, J. L., Robineau, B., Ambrosi, J. P., Costis, C., & Colin, F. (2005). Système hydrogéologique d’un massif minier ultrabisique de Nouvelle-Calédonie. Comptes Rendus Geoscience, 337, 1500–1508. doi: 10.1016/j.crte.2005.08.011
- Lachassagne, P., Ahmed, S. H., Dewandel, B., Courois, N., Maréchal, J. C., Perrin, J., & Wyns, R. (2009). Recent improvements in the conceptual model of hard rock aquifers and its application to the survey, management, modelling and protection of groundwater. Groundwater and Climate in Africa (Proceedings of the Kampala Conference, June 2008) IAHS Publ. 334.
- Lachassagne, P., Wyns, R., Bérard, P., Bruel, T., Chéry, L., Coutand, T., … Le Strat, P. (2001). Exploitation of high-yield in hard-rock aquifers: Downscaling methodology combining GIS and multicriteria analysis to delineate field prospecting zones. Ground Water, 39, 568–581. doi: 10.1111/j.1745-6584.2001.tb02345.x
- La Vigna, F., Mazza, R., Amanti, M., Di Salvo, C., Petitta, M., Pizzino, L., … Succhiarelli, C. (2016). Groundwater of Rome. Journal of Maps, 12, 88–93. doi: 10.1080/17445647.2016.1158669
- Manca, F., Viaroli, S., & Mazza, R. (2017). Hydrogeology of the Sabatini Volcanic District (Central Italy). Journal of Maps, 13, 252–259. http://www.tandfonline.com/doi/full/10.1080/17445647.2017.1297740 doi: 10.1080/17445647.2017.1297740
- Margat, J., & van der Gun, J. (2013). Groundwater around the world: A geographic synopsis. Boca Raton, Florida: CRC Press. ISBN 9781138000346.
- Marques, J. M., Carreira, P. M., Carvalho, M. R., Matias, M. J., Goff, F. E., Basto, M. J., … Rocha, L. (2008). Origins of high pH mineral waters from ultramafic rocks, Central Portugal. Applied Geochemistry, 23, 3278–3289. doi: 10.1016/j.apgeochem.2008.06.029
- Marroni, M., Meneghini, F., & Pandolfi, L. (2010). Anatomy of the Ligure-Piemontese subduction system: Evidence from late Cretaceous-middle Eocene convergent margin deposits in the northern Apennines, Italy. International Geology Review, 1–33. doi:10.1080/00206810903545493.
- Marroni, M., & Tribuzio, R. (1996). Gabbro-derived granulites from External Liguride units (northern Apennine, Italy): implications for the rifting processes in the western Tethys. Geologische Rundschau, 85, 239–249. doi: 10.1007/BF02422231
- Nikic, Z., Sreckovic-Batocanin, D., Burazer, M., Ristic, R., Papic, P., & Nikolic, V. (2013). A conceptual model of mildly alkaline water discharging from the Zlatibor ultramafic massif, western Serbia. Hydrogeology Journal, 21, 1147–1163. doi: 10.1007/s10040-013-0983-2
- Pagani, G., Papani, G., Rio, D., Torelli, L., Zanzucchi, G., & Zerbi, M. (1972). Osservazioni sulla giacitura delle ofioliti nelle alte valli del T. Ceno e del F. Taro [Remarks on the ophiolitic placement in the high valleys of Ceno and Taro streams (northern Apennines, Italy]. Memorie della Società Geologica Italiana, 11, 531–546.
- Ricci Lucchi, F. (1990). Turbidites in foreland and on-thrust basins of the northern Apennines. Palaeogeography, Palaeoclimatology, Palaeoecology, 77, 51–66. doi: 10.1016/0031-0182(90)90098-R
- Sander, P. (1997). Water-well siting in hard-rock areas: Identifying promising targets using a probabilistic approach. Hydrogeology Journal, 5, 32–43. doi: 10.1007/s100400050109
- Segadelli, S., Vescovi, P., Ogata, K., Chelli, A., Zanini, A., Boschetti, T., … Celico, F. (2017). A conceptual hydrogeological model of ophiolitic aquifers (serpentinised peridotite): The test example of Mt. Prinzera (Northern Italy). Hydrological Processes, 32, 969–1201. doi:10.1002/hyp.11090.
- Servizio Geologico d’Italia. (1968). Carta Geologica d’Italia in scala 1:100.000, Foglio 85- Castelnuovo né Monti, II Edizione [Geological map of Italy, scale 1:100.000. Sheet 85 Castelnuovo né Monti, II Edition]. Servizio Geologico d’Italia, Rome. http://193.206.192.231/carta_geologica_italia/tavoletta.php?foglio=85
- Servizio Geologico d’Italia. (1995). Carta Idrogeologica d’Italia – scala 1:50.000. Guida al rilevamento e alla rappresentazione [Hydrogeological Map of Italy, scale 1:100.000. A guide to mapping and a standard legend]. Istituto Poligrafico e Zecca dello Stato, 37pp. http://www.isprambiente.gov.it/it/pubblicazioni/periodici-tecnici/i-quaderni-serie-iii-del-sgi/carta-idrogeologica-d2019italia-1-50-000-guida-al
- Struckmeier, W., & Margat, J. (1995). Hydrogeological Maps – A Guide and a Standard Legend. – Int. Contrib. to Hydrogeol., Vol. 17; Hannover.
- Venturelli, G., Contini, S., Bonazzi, A., & Mangia, A. (1997). Weathering of ultramafic rocks and element mobility at Mt. Prinzera, northern Apennines, Italy. Mineralogical Magazine, 61, 765–778. doi: 10.1180/minmag.1997.061.409.02
- Walker, R. J., Holdsworth, R. E., Armitage, P. J., & Faulkner, D. R. (2013). Fault zone permeability structure evolution in basalts. Geology, 41, 59–62. doi: 10.1130/G33508.1
- Wright, E. P. (1992). The hydrogeology of cristalline basement aquifers in Africa. In E.G. Wright, & W.G. Burges (Eds.), Hydrogeology of crystalline basement aquifers in Africa (pp. 1–27). London: Geological Society Special Pubblication. n°66.
- Zanzucchi, G. (1980). I lineamenti geologici dell’Appennino parmense. Note illustrative alla Carta geologica e Sezioni geologiche della Provincia di Parma e zone limitrofe (1:100.000) [The geological features of the Apennines of the province of Parma administration. Illustrative notes to the geological paper and geological sections of the Province of Parma and surrounding areas (1:100,000)]. Parma, STEP editore.