ABSTRACT
A detailed map of rock glaciers at a scale of 1 : 40 000 is produced for the Western and High Tatra Mts., Western Carpathians, based on remotely sensed mapping. We inventoried a total of 383 rock glaciers, covering a total area of 13.84 km2. Most rock glaciers (85 %) are considered relict (without permafrost). These landforms have an average lower limit of 1684 m asl and occupy a total area of 12.50 km2. In contrast, intact rock glaciers (containing permafrost) cover a total area of 1.34 km2 and their average lower limit is located at 1986 m asl, which is 56 m above the previously suggested lower limit of discontinuous permafrost. The inventory adds new information about rock-glacier occurrence in the European high-mountain areas and improves the understanding of present and past environmental conditions in the region.
1. Introduction
Rock glaciers are tongue- or lobate-shaped landforms resulting from downslope creeping of ice-rich debris in mountain permafrost conditions (e.g. CitationBarsch, 1996; CitationBerthling, 2011; CitationKääb, 2013). Most rock glaciers exhibit characteristic surface morphology consisting of steep frontal and lateral slopes (for active rock glaciers mostly steeper than the angle of repose) enclosing rugged upper surface with frequent longitudinal and transversal ridges and furrows emerging due to the compression of debris-ice mixture associated with the rock-glacier flow, closely resembling lava streams (CitationKääb, 2013). Because of high portion of non-ice component, rock glaciers are usually able to retain much of their morphology long after the permafrost body has completely thawed (CitationHughes, Gibbard, & Woodward, 2003), meaning they can be identified even in a relict state and under vegetation cover if high-resolution remotely sensed imagery or digital elevation models are available (e.g. CitationColucci, Boccali, Žebre, & Guglielmin, 2016; CitationKellerer-Pirklbauer, Wagner, & Winkler, 2016).
Active and inactive rock glaciers represent important component of mountain cryosphere because they contain significant volumes of ground ice and act as long-term stores of water, even in arid or semi-arid regions (e.g. CitationAzócar & Brenning, 2010; CitationBrenning, 2005; CitationBurger, Degenhardt, & Giardino, 1999; CitationMillar, Westfall, & Delany, 2013). Analogously, relict rock glaciers indicate the existence of large amounts of ground ice in the past (e.g. CitationBarsch, 1996; CitationKääb, 2013) and because of a high storage capacity they noticeably affect current hydrology of mountain watersheds as well (e.g. CitationWagner, Pauritsch, & Winkler, 2016; CitationWinkler et al., 2016). Furthermore, rock glaciers are highly sensitive indicators of both present and past climate and permafrost evolution (e.g. CitationBarsch, 1996; CitationFrauenfelder & Kääb, 2000; CitationHaeberli et al., 2006), which can be mapped rapidly and at low cost to build-up extensive databases of permafrost evidence over large spatial scales. Consequently, there is a worldwide interest to compile rock-glacier inventories for a number of both purely scientific and practical reasons. In Europe, these investigations have been realized in larger scale particularly in the Alps (e.g. CitationBaroni, Carton, & Seppi, 2004; CitationColucci et al., 2016; CitationKellerer-Pirklbauer, Lieb, & Kleinferchner, 2012; CitationKrainer & Ribis, 2012; CitationNyenhuis, Hoelzle, & Dikau, 2005; CitationScotti, Brardinoni, Alberti, Frattini, & Crosta, 2013; CitationSeppi et al., 2012), the Pyrenees (e.g. CitationChueca, 1992; CitationSerrano, Agudo, & Martinez de Pisón, 1999), the Scandinavian Mts. (e.g. CitationLilleøren & Etzelmüller, 2011; CitationSollid & Sørbel, 1992) and the Southern Carpathians (e.g. CitationOnaca, Ardelean, Urdea, & Magori, 2017; CitationUrdea, 1992).
Unlike the above regions, rock-glacier mapping in the Western and High Tatra Mts., Western Carpathians (Slovakia and Poland), has been much less intensive. Rock glaciers in the study area were first noted by CitationPartsch (1923) who reported their occurrence in the High Tatra Mts. Later on, CitationKlimaszewski (1948, Citation1988) and CitationJahn (1958) described rock glaciers in the Polish territory of the region and called them ‘debris glaciers’ and ‘patch rock glaciers’, respectively. In the Slovak territory, many rock glaciers were described by CitationLukniš (1973) throughout the entire area of the High Tatra Mts., but they were referred to as ‘firn moraines’. A year later, CitationNemčok and Mahr (1974) published the most extensive rock-glacier inventory to date, in which they reported 13 and 18 sites with rock-glacier occurrence in the Slovak territory of the Western and High Tatra Mts., respectively, but a number of landforms have remained unmapped. After more than a 10-year hiatus, many rock-glacier studies have emerged since the turn of the eighties and nineties (CitationBaumgart-Kotarba & Kotarba, 2001; CitationDzierżek, Lindner, & Nitychoruk, 1987; CitationDzierżek & Nitychoruk, 1986; CitationKaszowski, Krzemień, & Libelt, 1988; CitationKociuba, 1996; CitationKotarba, 1986, Citation1988, Citation1991–1992, Citation1992; CitationKotarba, Kaszowski, & Krzemień, 1987; CitationLibelt, 1988), but exclusively from the Polish side of the mountains. This imbalance began to equalize from 2004 when CitationKędzia, Kotarba, and Mościcki (2004) published their case study of a single rock glacier located near the Veľké Hincovo pleso Lake in the Slovak High Tatra Mts. and since then, a large amount of work related to rock glaciers has been carried out in both Slovak (CitationKłapyta, 2009, Citation2010, Citation2011, Citation2012, Citation2013a, Citation2013b; CitationPánek et al., 2015; CitationPánek et al., 2016; CitationZasadni & Kłapyta, 2014) and Polish territory (CitationKłapyta, 2008, Citation2013b, Citation2015; CitationKłapyta & Kołaczek, 2009; CitationKotarba, 2007, Citation2013; CitationMakos, Rinterknecht, Braucher, Żarnowski, & Aster Team, 2016; CitationZasadni & Kłapyta, 2016) of the mountain ranges. In a surprising contrast, no rock glaciers have been reported in the regional overview of periglacial landforms occurring in the Western and High Tatra Mts. compiled by CitationRączkowska (2007). Most rock glaciers in the region are thought to be relict landforms (CitationKędzia, 2014) of the Late Glacial or the early Holocene age (e.g. CitationKaszowski et al., 1988; CitationKłapyta, 2011, Citation2013a; CitationKotarba, 1988, Citation2007).
Despite a considerable effort resulting in the above extensive list of literature, there still remain large areas both in the Western and High Tatra Mts., where rock glaciers have not been mapped yet. Furthermore, many previous investigations are only descriptive, incomplete and do not enable to determine the rock-glacier occurrence precisely or to extract their morphological attributes. Finally, none of the above rock-glacier studies have focused on the entire area of the Western and High Tatra Mts. Accordingly, the aim of our study is to complete the information on rock-glacier occurrence based on remotely sensed mapping, validated by field and literature data, and to introduce the first detailed map of rock glaciers covering the entire area of the Western and High Tatra Mts.
2. Study area
The Western and High Tatra Mts. are c. 42 km and c. 26 km, respectively, long mountain ranges, located in the northernmost part of the Carpathian arc and stretching longitudinally along the Slovak-Polish border (). They reach their maximum elevations of 2248 m asl and 2655 m asl at the top of Bystrá Peak and Gerlachovský štít Peak, respectively. Geology of the mountain ranges is dominated by Carboniferous-Permian granitic rocks, enriched with Palaeozoic methamorphic rocks, such as gneisses, migmatites or amplibolites in the Western Tatra Mts. On northern sides of the mountain ranges, the crystalline core is overlaid by thrust sheets of Mesozoic sedimentary rocks, particularly limestones and dolomites. The foothills have an extensive cover of Cretaceous-Palaeogene flysch rocks, such as claystones, siltstones, sandstones, conglomerates and breccias (CitationJurewicz, 2007; CitationNemčok et al., 1994; ).
Figure 1. Location map of the Western and High Tatra Mts. within Carpathians. Topography is based on 1 × 1 km resolution digital elevation model (DEM) derived from GTOPO30 and provided by the European Environment Agency (www.eea.europa.eu).
Note: The same data source was utilized to compile the inset map in the Main Map.
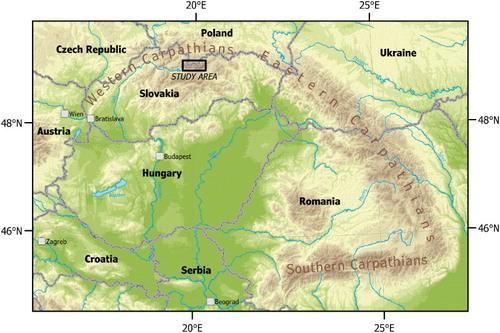
Figure 2. Schematic geological map of the Tatra Mts. based on CitationNemčok et al. (1994) and CitationBezák et al. (2013). Legend: 1 – gneiss rocks, migmatite, amphibolite (Palaeozoic); 2 – granitic rocks (Carboniferous-Permian); 3 – limestone, dolomite, sandstone, shale, quartzite (Mesozoic); 4 – claystone, siltstone, sandstone, conglomerate, breccia (Cretaceous-Palaeogene); 5 – sandstone, limestone, conglomerate, breccia (Palaeogene); 6 – fault (proved); 7 – fault (assumed). Red dashed lines indicate geographic subdivision of the Tatra Mts.
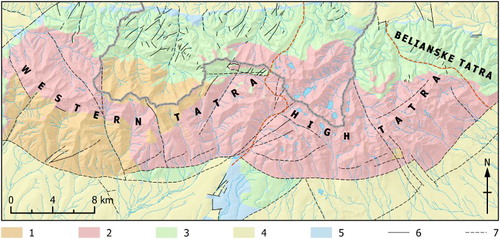
Extensive glaciers recurrently evolved during the cold phases of the Pleistocene (), which resulted in typical alpine-type topography with numerous cirques, U-shaped valleys and distinct moraine ridges in both mountain ranges (e.g. CitationKřížek & Mida, 2013; CitationMakos, Nitychoruk, & Zreda, 2013a, Citation2013b; CitationZasadni & Kłapyta, 2014), but all pronouncedly more developed in the High Tatra Mts. Currently, the mountains host no glaciers, but perennial snowfields and firn-ice patches can be found in suitable locations (CitationGądek, 2014). In the period 1981–2010, the mean annual air temperature (MAAT) at the top of Lomnický štít Peak, at 2635 m asl, was –3.4 °C and mean annual precipitation amounted 1653 mm (Slovak Hydrometerological Institute). The present-day climatic snowline has been calculated at c. 2500–2600 m asl and c. 2700–2800 m asl on northern and southern slopes, respectively (CitationZasadni & Kłapyta, 2009), and the lower limit of discontinuous permafrost has been estimated at 1930 ± 150–200 m asl based on the analysis of air thawing and freezing indices and complementary geophysical soundings and measurements of bottom temperature of snow (CitationDobiński, 1997a, Citation2004, Citation2005).
Figure 3. Last Glacial Maximum glacier extent (purple polygons) in the Tatra Mts. after CitationZasadni and Kłapyta (2014). Red dashed lines indicate geographic subdivision of the Tatra Mts. (color online).
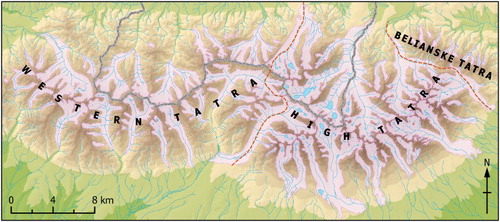
3. Methods
3.1. Areal extent and coordinate system
The map focuses on the Western and High Tatra Mts. in a scale of 1 : 40 000 and covers the area of 838.34 km2 between c. 49°06′–49°17′ N and c. 19°39′–20°15′ E (). The map is projected in the coordinate system WGS 1984 UTM Zone 34N. Accordingly, some layers, originally acquired in the coordinate system S-JTSK (based on Bessel ellipsoid), had to be transformed into the map projection. Considering the medium map scale, we converted the data using the transformation tools implemented in ESRI ArcMap 10.3. We employed the most accurate transformation algorithm (c. 1 m error) according to our own testing of the conversion accuracy between the above two coordinate systems (CitationKřížek, Uxa, & Mida, 2016).
3.2. Topography and additional map content
3.2.1. Digital elevation model
We used the existing digital elevation model (DEM) with a horizontal resolution of 10 m produced by stereo photogrammetry from aerial images (Tatra National Park, 2009) as a primary topographic base for the Main Map and as a supplementary source of information for mapping of rock glaciers, delineation of their contributing areas and extraction of elevation attributes. The model represents the most detailed and most widely utilized DEM covering the entire study area to date. Nonetheless, it contains elevation errors and other defects, such as straight break lines, which are mostly located in lower elevated areas unoccupied by rock glaciers, particularly along the alpine treeline and adjacent forest belt located on southern slopes (c.f. CitationZasadni & Kłapyta, 2014). Therefore, the model was adjusted prior to final map compilation so that the defective parts and their immediate surroundings (accounting for c. 11 % of the DEM area) were replaced by a supportive co-registrated 10 m DEM constructed from contour data with equidistance of 10 m provided by the Geodetic and Cartographic Institute Bratislava (GCIB). Both models were merged together and filtered by averaging within a moving window of 5 × 5 cells to ensure smooth transition at the contact of the models. Subsequently, shaded relief and contours with equidistance of 50 m were generated based on this updated and smoothed DEM and thereafter used in the final map.
3.2.2. Other topographic features
Beside the above DEM-based elements, we also added a set of elevation points into the map to provide complementary elevation information. In addition, other topographic features, such as watercourses, lakes, roads, railways, cable cars, built-up areas and state boundary, were integrated into the map for better orientation.
The elevation points and cable cars were obtained by digitizing the national topographic map series in a scale of 1 : 25 000 and 1 : 50 000 accessible on geoportals of the GCIB (geoportal.sk) and the Head Office of Geodesy and Cartography (HOGC; geoportal.gov.pl). In the Slovak territory of the study region, watercourses and lakes were taken from the database SVM 50, which is based on the Basemap of Slovak Republic in a scale of 1 : 50 000. In the Polish territory, these topographic features were acquired by manual digitization of the topographic map accessible at the geoportal of HOGC. Roads, railways and built-up areas for the entire area were extracted from the freely available OpenStreetMap database (download.geofabrik.de).
The topographic layers were checked for quality and particularly their mutual consistency and, if necessary, they were manually adjusted, for example, watercourse directions were slightly changed to appropriately fit the contour lines.
3.2.3. Additional map content
We included a schematic geological map () based on CitationNemčok et al. (1994) and CitationBezák et al. (2013) as a background layer in the Main Map in order to infer bedrock lithology of rock glaciers, with division into five geological units: Palaeozoic gneiss rocks, migmatite and amphibolite; Carboniferous-Permian granitic rocks; Mesozoic limestone, dolomite, sandstone, shale and quartzite; Cretaceous-Palaeogene claystone, siltstone, sandstone, conglomerate and breccia; and Palaeogene sandstone, limestone, conglomerate and breccia. Furthermore, the above layer was complemented with both proved and assumed faults based on the same data sources.
We also added the Last Glacial Maximum glacier extent in the Tatra Mts. () after CitationZasadni and Kłapyta (2014) to provide information about the relationship between rock glaciers and former glaciation limit.
Finally, as rock glaciers are widely accepted indicators of the lower limit of discontinuous mountain permafrost (CitationBarsch, 1996), we show the level of 1930 m asl in the Main Map, which corresponds to the average minimum elevation of discontinuous permafrost occurrence in the Tatra Mts. suggested by CitationDobiński (1997a, Citation2004, Citation2005).
3.3. Rock glaciers
3.3.1. Rock-glacier mapping
We mapped rock glaciers () throughout the entire area of the Western and High Tatra Mts. using aerial photographs from years 2010–2015 with a resolution of 0.1, 0.25, 0.5 and 1 m accessible on geoportals of the GCIB and HOGC and on Google Earth. Rock glaciers were identified and double-checked by both authors based on their typical surface morphological features visible on aerial imagery (), such as steep frontal and lateral slopes and longitudinal and/or transverse ridges and furrows. In areas with closed or semi-closed vegetation cover, we followed vegetation patterns associated with the rock-glacier surface morphology as well (CitationBarsch, 1996). The mapping was also supported by field inspections in 2014, 2015 and 2016, during which c. 22 % of the mapped landforms, mostly located in the High Tatra Mts., were visited and their occurrence and delineation was verified. The survey was supported by previous literature reports and maps as well (CitationDzierżek & Nitychoruk, 1986; CitationKłapyta, 2008, Citation2009, Citation2010, Citation2011, Citation2012, Citation2013a, Citation2013b, Citation2015; CitationKłapyta & Kołaczek, 2009; CitationKotarba, 1988, Citation1991–1992, Citation2007; CitationNemčok & Mahr, 1974; CitationZasadni & Kłapyta, 2016) that enable to extract precise information about the location of rock glaciers. However, we did not take over the literature information completely in all cases because the above mappings have emerged over several decades and had inconsistent data sources and mapping methodologies. Consequently, there is occasionally no consensus among researchers regarding the landform identification or classification. An excellent example of this inconsistency is the debris accumulation located near the Zadni Staw Polski Lake in the Pięciu Stawów Polskich Valley in the Polish High Tatra Mts., which was classified as a rock glacier (CitationDzierżek et al., 1987), a moraine ridge (CitationMakos et al., 2013a) as well as a rock avalanche (CitationZasadni & Kłapyta, 2016). Therefore, we carefully considered the integration of each individual previously mapped landform into the database mainly based on its appearance on aerial imagery in order to ensure the consistency of the inventory in terms of landform identification, classification and delineation throughout the whole region.
Figure 4. (A) Intact talus rock glacier near the Pusté pleso Lake in the Veľká Studená Valley, High Tatra Mts.; (B) relict talus rock glacier in the Kobylia Valley, Western Tatra Mts.; (C) fronts of intact and relict debris rock glaciers in the Slavkovská Valley, High Tatra Mts.; (D) densely vegetated relict talus rock glacier in the Furkotská Valley, High Tatra Mts.; note the person in the foreground for scale.
Note: White dashed lines delineate rock-glacier fronts.
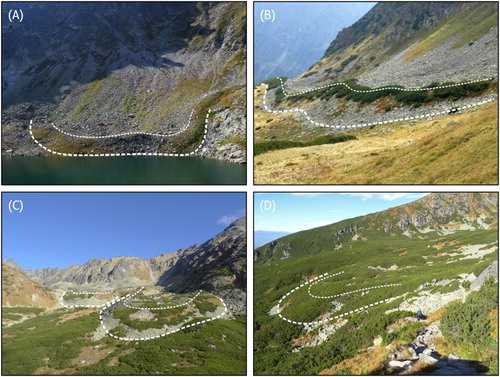
Figure 5. Aerial photographs showing the delineation of rock glaciers and their fronts (white solid lines) as well as contributing areas (white dashed lines); note well-defined ridge-and-furrow topography on some of the rock glaciers. (A) Relict debris rock glacier in the Spálená Valley, Western Tatra Mts.; (B) relict debris rock glacier in the Świstówka Roztocka Valley, High Tatra Mts.; (C) intact talus and debris rock glaciers forming a multipart rock glacier in the Batizovská Valley, High Tatra Mts.; (D) intact and relict talus and debris rock glaciers forming a multipart rock glacier in the Slavkovská Valley, High Tatra Mts. All the figures have a northern orientation.
Note: Parts of other rock glaciers and their contributing areas extending into the images are not highlighted.
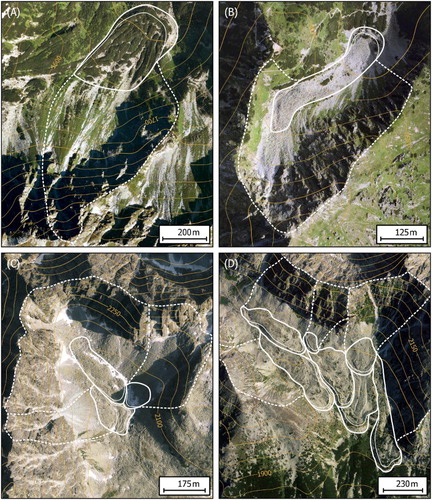
Each rock glacier was manually delineated along the foot of its frontal and lateral slopes towards the rooting zone () based on aerial photographs (c.f. CitationBaroni et al., 2004; CitationColucci et al., 2016; CitationKellerer-Pirklbauer et al., 2012; CitationKrainer & Ribis, 2012; CitationNyenhuis et al., 2005; CitationOnaca et al., 2017; CitationScotti et al., 2013). In the lower part, the interface between the rock glacier and its surroundings was in most cases clearly discernible. On the contrary, a distinction between the rock-glacier rooting zone and the above-lying contributing area was often much more difficult to differentiate (c.f. CitationColucci et al., 2016; CitationKrainer & Ribis, 2012). In such cases, the boundary was identified by both visual inspection of aerial images and searching for a sudden change in the DEM-derived slope inclination. Places with gradient exceeding the angle of repose of c. 35° (CitationBarsch, 1996) were implicitly considered as parts of the contributing area, probably corresponding to debris-free rock slopes or rock walls (sensu CitationGądek, Grabiec, Kędzia, & Rączkowska, 2016). The layers of slope inclination and shaded relief were also employed to refine the delineation of some large rock-glacier outlines. In cases where more rock-glacier bodies merge to form so-called multipart rock glacier (CitationBarsch, 1996), we delineated each of these distinguishable parts individually (c.f. CitationFalaschi, Castro, Masiokas, Tadono, & Ahumada, 2014; CitationKellerer-Pirklbauer et al., 2012).
3.3.2. Rock-glacier classification
After completion of the rock-glacier mapping, we classified rock glaciers according to the main source of material, permafrost presence and activity, and bedrock lithology.
Two rock-glacier categories, talus rock glaciers and debris rock glaciers (CitationBarsch, 1996; ), were adopted to differentiate the landforms based on the main source of material. As talus rock glaciers are regarded those landforms, which are unambiguously related to talus slopes situated above, delivering frost-shattered rock fragments for rock-glacier formation, and do not significantly extend down to the bottom of the valley. By contrast, debris rock glaciers are usually more extensive debris deposits, mostly covering valley floors, which evolve particularly from moraine-derived materials. We also include the most distinct landforms referred to as protalus (pronival) ramparts into the first category for two main reasons. Firstly, these landforms are usually very difficult to differentiate from talus rock glaciers not only from aerial imagery, but also in the field (e.g. CitationHedding, 2016; CitationHedding & Sumner, 2013). Secondly, these geomorphic features are largely genetically related to talus rock glaciers and many researchers describe them as embryonic stage of rock glaciers, that is, a kind of developmental continuum, closely resembling them in morphology, internal structure, ice content and morphodynamics (e.g. CitationBarsch, 1996; CitationHaeberli, 1985; CitationScapozza, 2015).
Intact and relict classes were set to differentiate the rock glaciers according to the presence of permafrost and activity (CitationBarsch, 1996). Intact rock glaciers (including both active and inactive landforms) host permafrost inside and may (active rock glaciers) or may not (inactive rock glaciers) move due to permafrost creep. In contrast, permafrost has completely thawed within relict rock glaciers. Borehole drilling, ground temperature measurements, geophysical soundings and/or monitoring of rock-glacier movements are particularly helpful to distinguish between the intact and relict rock glaciers (e.g. CitationHaeberli et al., 2006). Some of the above methods have been employed in the High Tatra Mts., suggesting probable permafrost presence in several rock glaciers (CitationKędzia, 2014), but the data are available for a limited number of landforms (CitationDobiński, 1997b; CitationGądek & Kędzia, 2008, Citation2009; CitationKędzia et al., 2004; CitationUxa & Mida, 2016, Citation2017). Consequently, the classification is largely based on morphological attributes discernible on aerial imagery that are widely accepted to be indicative of ground ice presence or absence and were adopted in many recent rock-glacier inventories as well (e.g. CitationColucci et al., 2016; CitationFalaschi et al., 2014; CitationOnaca et al., 2017; CitationScotti et al., 2013). Intact rock glaciers are characterized by steep frontal and lateral slopes, which often exceed the angle of repose of c. 35°, exhibit well-defined ridge-and-furrow topography, and completely lack or have sparse vegetation cover. In contrast, relict rock glaciers are typical of subdued topography with gentler slopes caused by permafrost degradation, and usually have extensive vegetation cover.
The bedrock lithology of individual rock glaciers was inferred from the schematic geological map (see section 3.2.3; ) based on rock-glacier outlines. In total, three geological units were identified for the mapped rock glaciers: Palaeozoic gneiss rocks, migmatite and amphibolite; Carboniferous-Permian granitic rocks; and Mesozoic limestone, dolomite, sandstone, shale and quartzite.
3.3.3. Rock-glacier contributing areas
Contributing area of each rock glacier, that is, the zone where source material for its formation is collected (), was computed using the Hydrology tools (e.g. CitationBolch & Gorbunov, 2014) implemented in ESRI ArcMap 10.3 based on the void-filled DEM (see section 3.2.1.). Because this debris-contributing area (sensu CitationJanke & Frauenfelder, 2008) may not be identical to the hydrological catchment of a rock glacier (particularly in the case of debris rock glaciers), only those parts of the respective rock glaciers, which presumably received material directly from the source zone (i.e. not secondarily through the rock-glacier flow), were used as the lower modelling limit. Several lines of evidence, such as the orientation of ridges and furrows and the connection between the outer edge of a rock glacier and neighbouring slope, were considered to determine these rock-glacier parts. The delineated contributing areas are essentially based on calculations confined to locations outside the rock glaciers, and therefore no problems that could be expected on rugged rock-glacier topography, such as flow divergence or presence of sinks with undefined flow direction, have been encountered. The contributing areas of lower-lying rock glaciers forming parts of multipart rock glaciers were set to include both the area of all the above-lying and genetically related rock glaciers and their contributing areas.
4. Mapping results and interpretation
The inventory contains a total of 383 rock glaciers occupying the area of 13.84 km2, which are supplied by rock material from 51.81 km2 of contributing areas (). Hence, the total rock-glacier-affected area in both mountain ranges constitutes 65.65 km2, which comprises c. 16 % of the area above 1375 m asl (∼lower limit of rock-glacier occurrence). The average rock-glacier density above this limit is 0.93 landforms per km2 and the average specific rock-glacier area accounts for 3.34 ha per km2. Less rock glaciers, 183 (c. 48 %), occur in the Western Tatra Mts. than in the High Tatra Mts. where 200 rock glaciers (c. 52 %) are located. However, this does not translate into the difference in the total rock-glacier area, which is by 0.45 km2 larger in the Western Tatra Mts. On the other hand, the total contributing area is by 2.60 km2 more extensive in the High Tatra Mts. ().
Table 1. General distribution and areal characteristics of rock glaciers and their contributing areas in the Western and High Tatra Mts.
Talus rock glaciers predominate in both mountain ranges, with c. 63 % and c. 74 % in the Western Tatra and High Tatra Mts., respectively, but their total area represents only c. 25 % and c. 42 % of all the rock glaciers. Accordingly, debris rock glaciers are substantially larger in size, which is on average around four to five times the average size of talus rock glaciers (). Debris rock glaciers also have substantially larger contributing areas (), which are capable to supply these voluminous landforms with a sufficient amount of material necessary for their development. Most rock glaciers are considered as relict; only seven landforms were classified as intact in the Western Tatra Mts. (c. 4 %) and other forty nine in the High Tatra Mts. (c. 25 %), representing c. 15 % of the total number of rock glaciers in the entire study region and covering the total area of 1.34 km2 (). Relict landforms extend down to 1375 m asl and 1414 m asl in the Western and High Tatra Mts., respectively, with the average front elevation of 1644 ± 119 m asl and 1731 ± 143 m asl, respectively (). Lower limit of intact rock glaciers occurs at 1761 m asl and 1831 m asl in the Western and High Tatra Mts., respectively, and their front elevation averages 1812 ± 30 m asl and 2011 ± 92 m asl, respectively. The discrepancy of nearly 200 m between the mountain ranges between the mountain ranges in the latter parameter results from wider elevation range where intact rock glaciers occur in the High Tatra Mts., and also from the limited number of intact landforms in the Western Tatra Mts. Consistently with the distribution patterns observed elsewhere (see CitationBarsch, 1996), both relict and intact rock glaciers have their lower limits located higher in southern aspects than in northerly exposed places. In the Western Tatra Mts., the average front elevation of relict and intact rock glaciers facing the NW–NE directions is at 1599 ± 107 m asl and 1823 ± 37 m asl, respectively, while landforms oriented to the SW–SE are at 1723 ± 103 m asl and 1819 ± 18 m asl, respectively. In the High Tatra Mts., the respective elevations are 1692 ± 159 m asl and 1943 ± 63 m asl for the NW–NE sectors and 1768 ± 116 m asl and 2018 ± 88 m asl for the SW–SE. Intact rock glaciers are mostly of talus type, with c. 86 % and c. 78 % in the Western and High Tatra Mts., respectively, and have on average around one and a half to two times smaller size than relict landforms ().
Figure 6. Minimum elevation of rock-glacier fronts in the (A) Western and (B) High Tatra Mts. Orange bars represent relict rock glaciers; intact rock glaciers are shown in blue. Black dashed line and contiguous grey areas indicate the lower limit of discontinuous permafrost occurrence of 1930 ± 150–200 m asl proposed by CitationDobiński (1997a, Citation2004, Citation2005).
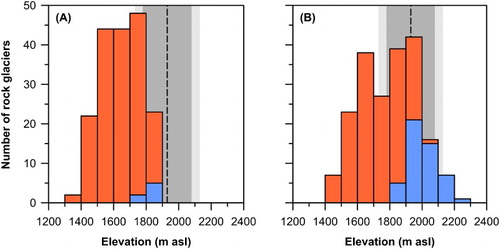
Rock glaciers occur in three different geological units (), which represent 100 % and c. 97 % of the area above 1375 m asl in the Western and High Tatra Mts., respectively. The total numbers and areas of rock glaciers built by different substrates well correlate with the areal proportion of these materials in the study region. Granitic rocks predominate here (), and therefore c. 65 % and 96 % of rock glaciers, covering the total area of 4.84 km2 and 6.46 km2, are formed within these substrates in the Western and High Tatra Mts., respectively (). More diverse geology of the Western Tatra Mts. promotes c. 27 % of rock glaciers (2.00 km2) consisting of Palaeozoic metamorphic rocks (particularly gneisses and migmatites) and c. 8 % of the landforms (0.31 km2) developed within Mesozoic limestones, dolomites, sandstones, shales and quartzites. On the contrary, only 1 % and 3 % of rock glaciers (0.07 km2 and 0.17 km2) in the High Tatra Mts. are formed by these materials (). In total, the non-granitic rock glaciers cover the area of 2.31 km2 and 0.24 km2 in the Western and High Tatra Mts., respectively.
Table 2. Areal extent of geological units above 1375 m asl (∼lower limit of rock-glacier occurrence) and rock-glacier presence in the Western and High Tatra Mts.
Rock glaciers consisting of Mesozoic limestones, dolomites, sandstones, shales and quartzites have the smallest average size and also show the lowest rock-glacier density as well as the lowest specific rock-glacier area in both mountain ranges (), which suggests that these materials are less favourable for rock-glacier formation in the study region. In contrast, rock glaciers built by granitic rocks and Palaeozoic metamorphic rocks are substantially larger and occur more frequently within the hosting geological units (). Their average sizes are almost identical in the individual mountain ranges, but in total the latter landforms are slightly larger, which is associated with both comparatively greater abundance of Palaeozoic metamorphic rocks in the Western Tatra Mts. and more extensive rock glaciers occurring there. On the other hand, the average rock-glacier density in the entire study region is distinctly the highest for granitic rocks, 1.12 landforms per km2, as is the average specific rock-glacier area, which in these substrates equals 4.07 ha per km2.
Intact rock glaciers can be utilized to approximate the contemporary lower limit of discontinuous permafrost on a regional scale, and relict rock glaciers can indicate the variations in discontinuous permafrost extent in the past (e.g. CitationBarsch, 1996; CitationHaeberli et al., 2006). The average front elevation of intact rock glaciers calculated collectively for both mountain ranges is at 1986 ± 109 m asl, which on average represents the area of 60.45 km2 above this level in the entire study region. The average value of 1986 m asl is 56 m above the previously proposed average discontinuous permafrost boundary of 1930 m asl (CitationDobiński, 1997a, Citation2004, Citation2005). Undoubtedly, the difference is principally affected by distinct methodologies. The former investigations (CitationDobiński, 1997a, Citation2004, Citation2005) assessed the permafrost occurrence in principle via the elevation of zero isotherm of air temperature, while the present study builds on the visual inspection of rock-glacier activity based on aerial imagery. Active rock glaciers typically exist in areas where MAAT is −2 °C or less (CitationBarsch, 1996). However, internal ice core may persist inside inactive rock glaciers even under positive MAAT because coarse blocky materials of rock glaciers tend to be substantially colder than outside air (e.g. CitationGorbunov, Marchenko, & Seversky, 2004; CitationHarris & Pedersen, 1998). Because inactive rock glaciers dominate the intact category in the Western and High Tatra Mts. and the cooling effect has been extensively observed here as well (CitationUxa & Mida, 2016, Citation2017), the average elevation of the intact rock-glacier front and the zero isotherm of air temperature should likely be reversed. This issue may be attributed to climate warming because the earlier estimates of permafrost distribution (CitationDobiński, 1997a, Citation2004, Citation2005) were mostly based on air temperature series two to three decades older (from 1985–1989 or 1985–1994) than our aerial images. The air temperature at Kasprowy Wierch (1991 m asl), located almost exactly in the middle of the study region, has been increasing on average by 0.02 °C per year (CitationŻmudzka, 2011) during 1966–2006 and has continued to rise at least until 2010 (CitationGądek & Leszkiewicz, 2012). Such a warming rate would elevate the zero isotherm level by 73–109 m in 20–30 years, assuming the average temperature lapse rate of 0.0055 °C per m that is based on 1951–1970 data from the eight highest elevated stations in the Western and High Tatra Mts. (CitationNiedzwiedz, 1992). In that case, the zero isotherm of air temperature would be at 2003–2039 m asl, which much better fits the presumed relation between air temperature and distribution of mostly inactive rock glaciers. In addition, the contemporary zero isotherm level may lie even higher because the warming apparently accelerated in the eighties of the last century (see e.g. CitationGądek & Leszkiewicz, 2012; CitationŻmudzka, 2011).
Because the lowest fronts of relict rock glaciers descend to around 1400 m asl (), the lower boundary of discontinuous permafrost in the Western and High Tatra Mts. in the Late Glacial or the early Holocene probably occurred around this level, provided that the rock glaciers could fully develop under the given climate conditions. In that case, discontinuous permafrost would occupy the area of c. 393 km2 in the entire study region, that is, around six and a half times more than the estimated contemporary discontinuous permafrost extent, and the MAAT at 1400 m asl could be −2 °C or less if we accept this value to be valid for active rock glaciers to develop (CitationBarsch, 1996). The contemporary MAAT at this elevation is estimated to be +3.4 °C based on the 1981–2010 temperature record from Lomnický štít Peak and the present average lapse rate of 0.0055 °C per m. Consequently, the temperature at the time of the rock-glacier formation was probably at least 5.4 °C lower than at present.
The spread between the average front elevation of intact and relict rock glaciers in the Western and High Tatra Mts. is well within the ranges observed in most surrounding regions, but local rock glaciers occur on average about 400–600 m lower than in the European Alps (). The decline in the front elevation is similar to that reported from some of the easternmost sub-regions of the European Alps and the Southern Carpathians (), which has been attributed to less precipitation towards the east (e.g. CitationOnaca et al., 2017), causing thinner snow cover during winter, and thus lower ground temperatures (sensu CitationGruber & Haeberli, 2009). About 100 m lower average front elevation of the intact rock glaciers in the Western and High Tatra Mts. than in the Southern Carpathians is most likely due to latitudinal temperature decrease, and is close to the previously reported difference in the contemporary lower limit of discontinuous permafrost of 70 m between these two Carpathian regions (CitationDobiński, 2005).
Table 3. Overview of front elevations of intact and relict rock glaciers in the European Alps and the Carpathians.
5. Conclusions
The map includes a total of 383 rock glaciers, making it the most comprehensive rock-glacier inventory for the entire area of the Western and High Tatra Mts. to date. Relict rock glaciers account for 85 % of the database and cover the total area of 12.50 km2. These landforms have their lowest limit around 1400 m asl, and thus the lower boundary of discontinuous permafrost zone in the Late Glacial or the early Holocene probably lied at this level, and covered the total area of around c. 393 km2. Intact rock glaciers constitute 15 % of the inventory and cover the total area of 1.34 km2. Their average front elevation of 1986 ± 109 m asl delineates the contemporary lower limit of discontinuous permafrost zone on a regional scale that occupies the total area of 60.45 km2.
The rock-glacier inventory adds to the current state of knowledge about the occurrence of these permafrost landforms in less investigated high-mountain regions located east of the European Alps. However, it must be emphasized that the inferred permafrost limits and extents should be understood as rather tentative in nature. Rock glaciers can provide a first-order evaluation of potential permafrost distribution, which generally tends to overestimate the permafrost extent at places without debris cover. Consequently, the present work is rather a starting point towards more thorough analyses of rock-glacier distribution and morphology and modelling of discontinuous permafrost distribution, which will substantially improve the understanding of present and past environmental conditions in the Western and High Tatra Mts.
Software
The mapping, digitizing, analyses of DEM and map compilation were all carried out using ESRI ArcMap 10.3. Final merger of vector and raster map elements was done in Adobe Acrobat Pro DC.
Rock Glaciers in the Western and High Tatra Mountains, Western Carpathians
Download PDF (29.9 MB)Acknowledgements
We would like to sincerely thank Marek Křížek for selfless financial support with payment of an article publishing charge. We also thank Bieke Cattoor, Daniel Falaschi and especially Andreas Kellerer-Pirklbauer for their thorough reviews that helped us to substantially improve both the article and the map. The Associate Editor, Jan-Christoph Otto, and the Editor-in-Chief, Mike J. Smith, are thanked for their editorial assistance.
Data availability
The shapefiles of rock-glacier outlines and contributing areas are available on request by the authors. Data users are requested to inform the data owners about the planned activities and invite them to contribute to any work that would lead to a co-authorship.
Disclosure statement
No potential conflict of interest was reported by the authors.
Additional information
Funding
References
- Azócar, G. F., & Brenning, A. (2010). Hydrological and geomorphological significance of rock glaciers in the Dry Andes, Chile (27°–33°S). Permafrost and Periglacial Processes, 21, 42–53. doi: 10.1002/ppp.669
- Baroni, C., Carton, A., & Seppi, R. (2004). Distribution and behaviour of rock glaciers in the Adamello-Presanella Massif (Italian Alps). Permafrost and Periglacial Processes, 15, 243–259. doi: 10.1002/ppp.497
- Barsch, D. (1996). Rockglaciers: Indicators for the present and former geoecology in high mountain environments. Berlin: Springer.
- Baumgart-Kotarba, M., & Kotarba, A. (2001). Deglaciation in the Sucha Woda and Pańszczyca Valleys in the Polish High Tatras. Studia Geomorphologica Carpatho-Balcanica, 35, 7–38.
- Berthling, I. (2011). Beyond confusion: Rock glaciers as cryo-conditioned landforms. Geomorphology, 131, 98–106. doi: 10.1016/j.geomorph.2011.05.002
- Bezák, V., Maglay, J., Polák, M., Kohút, M., Gross, P., Piotrowska, K., … Raczkowski, W. (2013). Geologicko-náučná mapa Tatier 1 : 50 000 [Geological-educational map of the Tatra Mts. 1 : 50 000]. Bratislava: State Geological Institute of Dionýz Štúr. Retrieved from http://mapserver.geology.sk/vtatry
- Bolch, T., & Gorbunov, A. P. (2014). Characteristics and origin of rock glaciers in northern Tien Shan (Kazakhstan/Kyrgyzstan). Permafrost and Periglacial Processes, 25, 320–332. doi: 10.1002/ppp.1825
- Brenning, A. (2005). Geomorphological, hydrological and climatic significance of rock glaciers in the Andes of central Chile (33–35°S). Permafrost and Periglacial Processes, 16, 231–240. doi: 10.1002/ppp.528
- Burger, K. C., Degenhardt, J. J., & Giardino, J. R. (1999). Engineering geomorphology of rock glaciers. Geomorphology, 31, 93–132. doi: 10.1016/S0169-555X(99)00074-4
- Chueca, J. (1992). A statistical analysis of the spatial distribution of rock glaciers, Spanish Central Pyrenees. Permafrost and Periglacial Processes, 3, 261–265. doi: 10.1002/ppp.3430030316
- Colucci, R. R., Boccali, C., Žebre, M., & Guglielmin, M. (2016). Rock glaciers, protalus ramparts and pronival ramparts in the south-eastern Alps. Geomorphology, 269, 112–121. doi: 10.1016/j.geomorph.2016.06.039
- Delaloye, R., & Morand, S. (1998). Rock glaciers, Entremont, Valais, Switzerland [International Permafrost Association, Data and Information Working Group, comp. Circumpolar Active-Layer Permafrost System (CAPS), version 1.0]. Retrieved from http://nsidc.org/data/docs/fgdc/ggd290_rockglac_switzer/index.html
- Dobiński, W. (1997a). Distribution of mountain permafrost in the High Tatras based on freezing and thawing indices. Biuletyn Peryglacjalny, 36, 29–37.
- Dobiński, W. (1997b). Warunki występowania zmarzliny w alpejskim piętrze Tatr Wysokich [The conditions of permafrost occurrence in the alpine belt of the High Tatra Mts.]. (Unpublished PhD thesis). Uniwersytet Śląski, Sosnowiec.
- Dobiński, W. (2004). Wieloletnia zmarzlina w Tatrach: Geneza, cechy, ewolucja [Permafrost in the Tatra Mts.: Genesis, features, evolution]. Przegląd Geograficzny, 76, 327–343.
- Dobiński, W. (2005). Permafrost of the Carpathian and Balkan Mountains, eastern and southeastern Europe. Permafrost and Periglacial Processes, 16, 395–398. doi: 10.1002/ppp.524
- Dzierżek, J., Lindner, L., & Nitychoruk, J. (1987). Rzeźba i osady czwartorzędowe Doliny Pięciu Stawow Polskich (Wysokie Tatry) [Relief and Quaternary sediments in the Pięć Stawów Polskich Valley (Wysokie Tatry Range)]. Przegląd Geologiczny, 35, 8–15.
- Dzierżek, J., & Nitychoruk, J. (1986). Types of fossil rock glaciers in the Polish High Tatra Mts. Bulletin of the Polish Academy of Sciences. Earth Sciences, 34, 409–418.
- Falaschi, D., Castro, M., Masiokas, M., Tadono, T., & Ahumada, A. L. (2014). Rock glacier inventory of the Valles Calchaquíes region (∼ 25°S), Salta, Argentina, derived from ALOS data. Permafrost and Periglacial Processes, 25, 69–75. doi: 10.1002/ppp.1801
- Frauenfelder, R. (1998). Rock glaciers, Fletschhorn area, Valais, Switzerland [International Permafrost Association, Data and Information Working Group, comp. Circumpolar Active-Layer Permafrost System (CAPS), version 1.0]. Retrieved from http://nsidc.org/data/docs/fgdc/ggd287_rockglac_switzer/index.html
- Frauenfelder, R., & Kääb, A. (2000). Towards a palaeoclimatic model of rock-glacier formation in the Swiss Alps. Annals of Glaciology, 31, 281–286. doi: 10.3189/172756400781820264
- Gądek, B. (2014). Climatic sensitivity of the non-glaciated mountains cryosphere (Tatra Mts., Poland and Slovakia). Global and Planetary Change, 121, 1–8. doi: 10.1016/j.gloplacha.2014.07.001
- Gądek, B., Grabiec, M., Kędzia, S., & Rączkowska, Z. (2016). Reflection of climate changes in the structure and morphodynamics of talus slopes (the Tatra Mountains, Poland). Geomorphology, 263, 39–49. doi: 10.1016/j.geomorph.2016.03.024
- Gądek, B., & Kędzia, S. (2008). Winter ground surface temperature regimes in the zone of sporadic discontinuous permafrost, Tatra Mountains (Poland and Slovakia). Permafrost and Periglacial Processes, 19, 315–321. doi: 10.1002/ppp.623
- Gądek, B., & Kędzia, S. (2009). Problemy detekcji wieloletniej zmarzliny na podstawie temperatury u spągu zimowej pokrywy śnieżnej na przykładzie Tatr [The problem of permafrost detection based on bottom temperature snow cover – The Tatra Mts. case]. Przegląd Geograficzny, 81, 75–91. doi: 10.7163/PrzG.2009.4.3
- Gądek, B., & Leszkiewicz, J. (2012). Impact of climate warming on the ground surface temperature in the sporadic permafrost zone of the Tatra mountains, Poland and Slovakia. Cold Regions Science and Technology, 79–80, 75–83. doi: 10.1016/j.coldregions.2012.03.006
- Gorbunov, A. P., Marchenko, S. S., & Seversky, E. V. (2004). The thermal environment of blocky materials in the mountains of Central Asia. Permafrost and Periglacial Processes, 15, 95–98. doi: 10.1002/ppp.478
- Gruber, S., & Haeberli, W. (2009). Mountain permafrost. In R. Margesin (Ed.), Permafrost soils (pp. 33–44). Berlin: Springer.
- Guglielmin, M., & Smiraglia, C. (1998). The rock glacier inventory of the Italian Alps. In A. G. Lewkowicz & M. Allard (Eds.), Proceedings of the Seventh International Conference on Permafrost (pp. 375–382). Ottawa: National Research Council of Canada.
- Haeberli, W. (1985). Creep of mountain permafrost: Internal structure and flow of alpine rock glaciers. Mitteilungen der Versuchsanstalt für Wasserbau, Hydrologie und Glaziologie, 77, 1–142.
- Haeberli, W., Hallet, B., Arenson, L., Elconin, R., Humlum, O., Kääb, A., … Vonder Mühll, D. (2006). Permafrost creep and rock glacier dynamics. Permafrost and Periglacial Processes, 17, 189–214. doi: 10.1002/ppp.561
- Harris, S. A., & Pedersen, D. E. (1998). Thermal regimes beneath coarse blocky materials. Permafrost and Periglacial Processes, 9, 107–120. doi: 10.1002/(SICI)1099-1530(199804/06)9:2<107::AID-PPP277>3.0.CO;2-G
- Hedding, D. W. (2016). Pronival ramparts: Origin and development of terminology. Erdkunde, 70, 141–151. doi: 10.3112/erdkunde.2016.02.03
- Hedding, D. W., & Sumner, P. (2013). Diagnostic criteria for pronival ramparts: Site, morphological and sedimentological characteristics. Geografiska Annaler: Series A, Physical Geography, 95, 315–322. doi: 10.1111/geoa.12021
- Hoelzle, M. (1998). Rock glaciers, Upper Engadin, Switzerland [International Permafrost Association, Data and Information Working Group, comp. Circumpolar Active-Layer Permafrost System (CAPS), version 1.0]. Retrieved from http://nsidc.org/data/docs/fgdc/ggd288_rockglac_switzer/
- Hughes, P. D., Gibbard, P. L., & Woodward, J. C. (2003). Relict rock glaciers as indicators of Mediterranean palaeoclimate during the Last Glacial Maximum (Late Würmian) in northwest Greece. Journal of Quaternary Science, 18, 431–440. doi: 10.1002/jqs.764
- Imhof, M. (1998). Rock glaciers, Bernese Alps, Western Switzerland [International Permafrost Association, Data and Information Working Group, comp. Circumpolar Active-Layer Permafrost System (CAPS), version 1.0]. Retrieved from https://nsidc.org/data/docs/fgdc/ggd286_rockglac_switzer/index.html
- Jahn, A. (1958). Mikrorelief peryglacjalny Tatr i Babiej Góry [Periglacial microrelief of the Tatras and Babia Góra Massif]. Biuletyn Peryglacjalny, 6, 57–80.
- Janke, J., & Frauenfelder, R. (2008). The relationship between rock glacier and contributing area parameters in the Front Range of Colorado. Journal of Quaternary Science, 23, 153–163. doi: 10.1002/jqs.1133
- Jurewicz, E. (2007). Multistage evolution of the granitoid core in Tatra Mountains. In A. Kozłowski & J. Wiszniewska (Eds.), Granitoids in Poland (pp. 307–317). Warszawa: Warsaw University.
- Kaszowski, L., Krzemień, K., & Libelt, P. (1988). Postglacjalne modelowanie cyrków lodowcowych w Tatrach Zachodnich [Postglacial modelling of the glacial cirques in the Western Tatras]. Prace Geograficzne, 71, 121–141.
- Kääb, A. (2013). Rock glaciers and protalus forms. In S. A. Elias & C. J. Mock (Eds.), Encyclopedia of Quaternary science (2nd ed., pp. 535–541). Amsterdam: Elsevier.
- Kellerer-Pirklbauer, A., Lieb, G. K., & Kleinferchner, H. (2012). A new rock glacier inventory of the eastern European Alps. Austrian Journal of Earth Sciences, 105, 78–93.
- Kellerer-Pirklbauer, A., Wagner, T., & Winkler, G. (2016). Inventarisierung von blockgletscherverdächtigen Formen und deren hydrologischen Einzugsgebieten in den steirischen Niederen Tauern mit Hilfe von hochaufgelösten Geländemodellen [Inventorying rock glacier-suspected landforms and their hydrological catchments in the Styrian part of the Niedere Tauern range using high-resolution digital elevation models]. Joannea – Geologie und Palaontologie, 12, 53–62.
- Kędzia, S. (2014). Are there any active rock glaciers in the Tatra Mountains? Studia Geomorphologica Carpatho-Balcanica, 48, 5–16. doi: 10.1515/sgcb-2015-0001
- Kędzia, S., Kotarba, A., & Mościcki, J. (2004). Lodowiec gruzowy nad Wielkim Hińczowym Stawem w Tatrach Słowackich – wyniki wstępnych badań termicznych [Rock glacier above the Wielki Hińczowy Staw in the Slovak Tatra Mts. – Preliminary results of thermal investigations]. In A. Styczyńska & A. A. Marsz (Eds.), XXX Międzynarodowe sympozjum polarne (pp. 167–177). Gdynia: Akademia Morska.
- Klimaszewski, M. (1948). Polskie Karpaty Zachodnie w okresie dyluwialnym [Polish Western Carpathians during the diluvium]. Wrocław: Wrocławskie towarzystwo naukowe.
- Klimaszewski, M. (1988). Rzeźba Tatr Polskich [Relief of the Polish Tatra Mountains]. Warszawa: Państwowe Wydawnictwa Naukowe.
- Kłapyta, P. (2008). Reliktowe wały lodowo-morenowe w zachodniej Cyrku Pyszniańskiego, Tatry Zachodnie [Relic ice-cored lateral moraines in the western part of the Pyszniański Cirque, the Western Tatra Mts.]. Prace Geograficzne, 120, 65–77.
- Kłapyta, P. (2009). Glacial and periglacial relief on the southern slopes of the Western Tatra Mts. (Slovakia) – The results of the first detailed geomorphological mapping of the Žiarska, Jamnicka, Račkova and Bystra Valleys. Landform Analysis, 10, 50–57.
- Kłapyta, P. (2010). Przebieg deglacjacji Doliny Bystrej (Tatry Zachodnie, Słowacja) podczas ostatniego zlodowacenia w świetle analiz geomorfologicznych oraz datowania względnego form metodą młotka Schmidta [Deglaciation of the Bystra Valley (Western Tatra Mts., Slovakia) during the last glaciation in the light of geomorphological analyses and relative age dating by Schmidt hammer]. In Z. Krzan (Ed.), Nauka a zarządzanie obszarem Tatr i ich otoczeniem, Tom I – Nauki o Ziemi (pp. 63–68). Zakopane: Tatrzański Park Narodowy.
- Kłapyta, P. (2011). Relative surface dating of rock glacier systems in the Žiarska Valley, the Western Tatra Mountains, Slovakia. Studia Geomorphologica Carpatho-Balcanica, 45, 89–106.
- Kłapyta, P. (2012). Ewolucja rzeźby wysokogórskiej Tatr Zachodnich w późnym glacjale i holocenie [Late glacial and Holocene evolution of alpine relief of the Western Tatra Mts.]. (Unpublished PhD thesis). Uniwersytet Jagielloński, Kraków.
- Kłapyta, P. (2013a). Application of Schmidt hammer relative age dating to Late Pleistocene moraines and rock glaciers in the Western Tatra Mountains, Slovakia. Catena, 111, 104–121. doi: 10.1016/j.catena.2013.07.004
- Kłapyta, P. (2013b). Ewolucja rzeźby wysokogórskiej Tatr Zachodnich w późnym glacjale [Late glacial evolution of the Western Tatras’ alpine relief]. In R. K. Borówka, A. Cedro, & I. Kavetskyy (Eds.), Współczesne problemy badań geograficznych (pp. 73–82). Szczecin: University of Szczecin.
- Kłapyta, P. (2015). Deglacjacja północnego i południowego skłonu Tatr Zachodnich w trakcie ostatniego zlodowacenia w świetle dotychczasowych badań geomorfologicznych [Deglaciation of northern and southern slope of the Tatra Mountains during the last glaciation, in the light of geomorphological studies]. In A. Chrobak & A. Kotarba (Eds.), NAUKA TATROM, Tom I – Nauki o Ziemi (pp. 67–78). Zakopane: Tatrzański Park Narodowy.
- Kłapyta, P., & Kołaczek, P. (2009). The last millenium slope processes and anthropogenic activity recorded in the sediments from the Pyszniańska Glade, Western Tatra Mountains (Poland). Studia Geomorphologica Carpatho-Balcanica, 43, 145–163.
- Kociuba, W. (1996). Textural features of some debrises patches in the High Tatra. In J. Repelewska-Pękalowa & K. Pękala (Eds.), Wyprawy Geograficzne na Spitsbergen (pp. 163–172). Lublin: Maria Curie-Skłodowska University.
- Kotarba, A. (1986). Lodowce gruzowe w Tatrach [Rock glaciers in the Tatra Mountains]. Wszechświat, 87, 97–99.
- Kotarba, A. (1988). Fossil rock glaciers in the Polish Tatra Mountains: Origin and age. In M. Pecsi & S. Starkel (Eds.), Palaeography of Carpathian regions (pp. 161–169). Budapest: Hungarian Academy of Sciences.
- Kotarba, A. (1991–1992). Reliktowe lodowce gruzowe jako element deglacjacji Tatr Wysokich [Relict rock glaciers as an element of the deglaciation of the High Tatra Mountains]. Studia Geomorphologica Carpatho-Balcanica, 25–26, 133–149.
- Kotarba, A. (1992). Natural environment and landform dynamics of the Tatra Mountains. Mountain Research and Development, 12, 105–129. doi: 10.2307/3673786
- Kotarba, A. (2007). Lodowce gruzowe i wały niwalne – efekt późnoglacjalnej ewolucji rzeźby Tatr [Rock glaciers and protalus ramparts – An effect of the Lateglacial evolution of the Tatra Mountains]. Przegląd Geograficzny, 79, 199–213.
- Kotarba, A. (2013). Rzeźba systemu dolinnego Suchej Wody i Pańszczycy [Relief of valley systems of the Suchej Wody and Pańszczyca Valleys]. In Z. Rączkowska & A. Kotarba (Eds.), Dolina Suchej Wody w Tatrach. Środowisko i jego współczesne przemiany (pp. 15–33). Warszawa: Polish Academy of Sciences.
- Kotarba, A., Kaszowski, L., & Krzemień, K. (1987). High-mountain denudational system of the Polish Tatra Mountains. Prace Geograficzne, Special Issue, 3, 69–95.
- Krainer, K., & Ribis, M. (2012). A rock glacier inventory of the Tyrolean Alps (Austria). Austrian Journal of Earth Sciences, 105, 42–47.
- Křížek, M., & Mida, P. (2013). The influence of aspect and altitude on the size, shape and spatial distribution of glacial cirques in the High Tatras (Slovakia, Poland). Geomorphology, 198, 57–68. doi: 10.1016/j.geomorph.2013.05.012
- Křížek, M., Uxa, T., & Mida, P. (2016). Praktikum morfometrických analýz reliéfu [Practicum of morphometric analyses of relief]. Praha: Karolinum.
- Libelt, P. (1988). Warunki i przebieg sedymentacji osadów postglacjalnych w cyrkach lodowcowych Tatr Zachodnich na przykładzie Kotła Starorobociańskiego [Conditions and course of sedimentation of postglacial deposits by taking as example the Starorobociański Cirque, Western Tatra Mts.]. Studia Geomorphologica Carpatho-Balcanica, 22, 63–82.
- Lilleøren, K. S., & Etzelmüller, B. (2011). A regional inventory of rock glaciers and ice-cored moraines in Norway. Geografiska Annaler: Series A, Physical Geography, 93, 175–191. doi: 10.1111/j.1468-0459.2011.00430.x
- Lukniš, M. (1973). Reliéf Vysokých Tatier a ich predpolia [Relief of the High Tatra Mountains and their foreland]. Bratislava: Vydavateľstvo Slovenskej akadémie vied.
- Makos, M., Nitychoruk, J., & Zreda, M. (2013a). Deglaciation chronology and paleoclimate of the Pięciu Stawów Polskich/Roztoki Valley, High Tatra Mountains, Western Carpathians, since the Last Glacial Maximum, inferred from 36Cl exposure dating and glacier–climate modelling. Quaternary International, 293, 63–78. doi: 10.1016/j.quaint.2012.01.016
- Makos, M., Nitychoruk, J., & Zreda, M. (2013b). The Younger Dryas climatic conditions in the Za Mnichem Valley (Polish High Tatra Mountains) based on exposure-age dating and glacier-climate modelling. Boreas, 42, 745–761. doi: 10.1111/j.1502-3885.2012.00298.x
- Makos, M., Rinterknecht, V., Braucher, R., Żarnowski, M., & Aster Team. (2016). Glacial chronology and palaeoclimate in the Bystra catchment, Western Tatra Mountains (Poland) during the Late Pleistocene. Quaternary Science Reviews, 134, 74–91. doi: 10.1016/j.quascirev.2016.01.004
- Millar, C. I., Westfall, R. D., & Delany, D. L. (2013). Thermal and hydrologic attributes of rock glaciers and periglacial talus landforms: Sierra Nevada, California, USA. Quaternary International, 310, 169–180. doi: 10.1016/j.quaint.2012.07.019
- Nemčok, A., & Mahr, T. (1974). Kamenné ľadovce v Tatrách [Fossil rock glaciers in Tatras]. Geografický časopis, 26, 359–373.
- Nemčok, J., Bezák, V., Biely, A., Gorek, A., Gross, P., Halouzka, R., … Zelman, J. (1994). Geologická mapa Tatier 1 : 50 000 [Geological map of the Tatra Mts. 1 : 50 000]. Bratislava: State Geological Institute of Dionýz Štúr.
- Niedzwiedz, T. (1992). Climate of the Tatra Mountains. Mountain Research and Development, 12, 131–146. doi: 10.2307/3673787
- Nyenhuis, M., Hoelzle, M., & Dikau, R. (2005). Rock glacier mapping and permafrost distribution modelling in the Turtmanntal, Valais, Switzerland. Zeitschrift für Geomorphologie, 49, 275–292.
- Onaca, A., Ardelean, F., Urdea, P., & Magori, B. (2017). Southern Carpathian rock glaciers: Inventory, distribution and environmental controlling factors. Geomorphology, 293, Part B, 391–404. doi: 10.1016/j.geomorph.2016.03.032
- Partsch, J. (1923). Die Hohe Tatra zur Eiszeit [The High Tatra Mountains in the Ice Age]. Leipzig: F. Hirt & Sohn.
- Pánek, T., Engel, Z., Mentlík, P., Braucher, R., Břežný, M., Škarpich, V., & Zondervan, A. (2016). Cosmogenic age constraints on post-LGM catastrophic rock slope failures in the Tatra Mountains (Western Carpathians). Catena, 138, 52–67. doi: 10.1016/j.catena.2015.11.005
- Pánek, T., Mentlík, P., Ditchburn, B., Zondervan, A., Norton, K., & Hradecký, J. (2015). Are sackungen diagnostic features of (de)glaciated mountains? Geomorphology, 248, 396–410. doi: 10.1016/j.geomorph.2015.07.022
- Rączkowska, Z. (2007). Współczesna rzeźba peryglacjalna wysokich gór Europy [Present-day periglacial relief in high mountains of Europe]. Warszawa: Polish Academy of Sciences.
- Reynard, E., Lambiel, C., & Wenker, L. (1998). Rock glaciers, Printse Valley, Valais, Switzerland. [International Permafrost Association, Data and Information Working Group, comp. Circumpolar Active-Layer Permafrost System (CAPS), version 1.0]. Retrieved from http://nsidc.org/data/docs/fgdc/ggd291_rockglac_switzer/
- Scapozza, C. (2015). Investigation on protalus ramparts in the Swiss Alps. Geographica Helvetica, 70, 135–139. doi: 10.5194/gh-70-135-2015
- Schoeneich, P. (1998). Rock glaciers of the Prealps, Vaud, Switzerland [International Permafrost Association, Data and Information Working Group, comp. Circumpolar Active-Layer Permafrost System (CAPS), version 1.0]. Retrieved from http://nsidc.org/data/docs/fgdc/ggd292_rockglac_switzer/index.html
- Scotti, R., Brardinoni, F., Alberti, S., Frattini, P., & Crosta, G. B. (2013). A regional inventory of rock glaciers and protalus ramparts in the central Italian Alps. Geomorphology, 186, 136–149. doi: 10.1016/j.geomorph.2012.12.028
- Seppi, R., Carton, A., Zumiani, M., Dall’Amico, M., Zampedri, G., & Rigon, R. (2012). Inventory, distribution and topographic features of rock glaciers in the southern region of the Eastern Italian Alps (Trentino). Geografia Fisica e Dinamica Quaternaria, 35, 185–197. doi: 10.4461/GFDQ.2012.35.17
- Serrano, E., Agudo, C., & Martinez de Pisón, E. (1999). Rock glaciers in the Pyrenees. Permafrost and Periglacial Processes, 10, 101–106. doi: 10.1002/(SICI)1099-1530(199901/03)10:1<101::AID-PPP308>3.0.CO;2-U
- Sollid, J. L., & Sørbel, L. (1992). Rock glaciers in Svalbard and Norway. Permafrost and Periglacial Processes, 3, 215–220. doi: 10.1002/ppp.3430030307
- Urdea, P. (1992). Rock glaciers and periglacial phenomena in the Southern Carpathians. Permafrost and Periglacial Processes, 3, 267–273. doi: 10.1002/ppp.3430030317
- Uxa, T., & Mida, P. (2016). First results from thermal investigations of rock glaciers in the Slovak High Tatra Mountains, Western Carpathians. In F. Günther & A. Morgenstern (Eds.), XI. International Conference on Permafrost – Book of abstracts (pp. 1060–1061). Potsdam: Bibliothek Wissenschaftspark Albert Einstein.
- Uxa, T., & Mida, P. (2017). Ground surface thermal regime of rock glaciers in the High Tatra Mts., Slovakia. Geophysical Research Abstracts, 19, EGU2017–1740.
- Wagner, T., Pauritsch, M., & Winkler, G. (2016). Impact of relict rock glaciers on spring and stream flow of alpine watersheds: Examples of the Niedere Tauern range, Eastern Alps (Austria). Austrian Journal of Earth Sciences, 109, 84–98. doi: 10.17738/ajes.2016.0006
- Winkler, G., Wagner, T., Pauritsch, M., Birk, S., Kellerer-Pirklbauer, A., Benischke, R., … Hergarten, S. (2016). Identification and assessment of groundwater flow and storage components of the relict Schöneben rock glacier, Niedere Tauern range, Eastern Alps (Austria). Hydrogeology Journal, 24, 937–953. doi: 10.1007/s10040-015-1348-9
- Zasadni, J., & Kłapyta, P. (2009). An attempt to assess the modern and the Little Ice Age climatic snowline altitude in the Tatra Mountains. Landform Analysis, 10, 124–133.
- Zasadni, J., & Kłapyta, P. (2014). The Tatra Mountains during the Last Glacial Maximum. Journal of Maps, 10, 440–456. doi: 10.1080/17445647.2014.885854
- Zasadni, J., & Kłapyta, P. (2016). From valley to marginal glaciation in alpine-type relief: Lateglacial glacier advances in the Pięć Stawów Polskich/Roztoka Valley, High Tatra Mountains, Poland. Geomorphology, 253, 406–424. doi: 10.1016/j.geomorph.2015.10.032
- Żmudzka, E. (2011). Contemporary climate changes in the high mountain part of the Tatras. Miscellanea Geographica – Regional Studies on Development, 15, 93–102. doi: 10.2478/v10288-012-0005-6